Chapter 1 Applied Physiology of Body Fluids in Dogs and Cats
Distribution of body fluids
In health, approximately 60% of an adult animal’s body weight is water. Estimates of total body water in adult dogs that are neither very thin nor obese are 534 to 660 mL/kg.26,59 Total body water of adult cats also was determined to be approximately 60%.56 There are some species and individual variations in total body water, likely related to age, sex, and body composition. In humans, total body water decreases with age and is lower in women than in men.13 Neonatal dogs and cats have higher total body water content (80% of body weight) than adults (60% of body weight),30 and an age-related decrease in total body water has been described in puppies and kittens during the first 6 months of life.35 Total body water was approximately 70% of total body weight in racing Greyhounds, likely due to low body fat content.21 Because fat has a lower water content than lean tissue, fluid needs should be estimated on the basis of lean body mass to avoid overhydration, especially in patients with cardiac or renal insufficiency or in those with hypoproteinemia. Formulas for estimating lean body mass are based on the assumptions that (1) in normal small animal patients, approximately 20% of body weight is due to fat, (2) morbid obesity increases body fat to approximately 30% of body weight, and (3) body weight is a reasonable estimate of lean body mass in thin patients:
As shown in Figure 1-1, the largest volume of fluid in the body is inside cells. The intracellular fluid (ICF) compartment comprises approximately 40% of body weight (approximately two thirds of total body water). The composition of ICF is very different from extracellular fluid (ECF) (Fig. 1-2). Intracellular homeostasis is maintained by shifts in water, solutes, and numerous other substances across the cell membrane.
Unfortunately, data from dye dilution studies of ECF volume are difficult to interpret because no indicator is truly confined to the ECF space. Estimates of ECF vary dramatically with the indicator used. ECF volumes reported for adult, healthy dogs and cats vary between 15% and 30% of body weight. The wide range in estimates of ECF volume likely results from the variety of techniques used to measure this space and the heterogeneity of ECFs, which include interstitial fluid (ISF), plasma, and transcellular fluids. Dense connective tissue, cartilage, and bone also contain a small amount of ECF. From a physiologic perspective and based on multiple studies using various indicators, the most accurate estimate of the ECF in adult small animals is 27% of lean body weight. However, an easier distribution of body fluids to remember is the 60:40:20 rule: 60% of body weight is water, 40% of body weight is ICF, and 20% of body weight is ECF (see Fig. 1-1). Many clinicians use 20% as an estimate for ECF when calculating fluid therapy needs for their patients.
As mentioned above and as shown in Figure 1-1, ECF is distributed among several different subcompartments. Most ECF (about three fourths) is in spaces surrounding cells and is called interstitial fluid. Although accurate studies of the size of the ISF compartment in dogs and cats have not been reported, estimates derived from measurement of fluids in other compartments indicate that the ISF comprises approximately 15% of body weight (approximately 24% of total body water). About one fourth of the ECF is within blood vessels and is called the intravascular compartment (plasma). Intravascular fluids are approximately 5% of body weight (approximately 8% to 10% of total body water). Most of the intravascular fluid is plasma. Plasma volume estimates range from 42 to 58 mL/kg in adult dogs that are neither very thin nor obese.26 Estimates for plasma volume in cats are 37 to 49 mL/kg.26 Blood volume, which includes erythrocytes, is a function of lean body mass, and estimated blood volume in dogs is 77 to 78 mL/kg (8% to 9% of body weight) and in cats is 62 to 66 mL/kg (6% to 7% of body weight).24 Racing Greyhounds may have higher blood volumes (110 to 114 mL/kg) than other breeds, possibly related to higher lean body mass.21
Fluids produced by specialized cells to form cerebrospinal fluid, gastrointestinal fluid, bile, glandular secretions, respiratory secretions, and synovial fluid are in the transcellular fluid compartment, which is estimated as approximately 1% of body weight (approximately 2% of total body water). Dense connective tissues, bone, and cartilage contain approximately 15% of total body water. However, these tissues exchange fluids slowly with other compartments. Because this fluid usually is not taken into account for routine fluid therapy, this compartment is not shown in Figure 1-1. Thus, a more simplified distribution of total body water often used for fluid therapy is:
Distribution of body solutes
In addition to water, body fluids contain various concentrations of solutes. Total body content of solutes may be measured by cadaver analysis (desiccation) or by isotope dilution studies. Every solute has a space or apparent volume of distribution. Dilution studies of body solute content yield variable results, depending on the volume of distribution of the particular tracer used to estimate the solute space. There are limited data in the literature from cadaver and isotope dilution studies of body solute content in small animals, and most of the following discussion is based on data from studies in humans.13,48
Solutes are not distributed homogeneously throughout body fluids. Vascular endothelium and cell membranes have different permeabilities for various solutes. Healthy vascular endothelium is relatively impermeable to the cellular components of blood and to plasma proteins. Consequently, the volume of distribution of cells and proteins is the plasma space itself. However, the vascular endothelium is freely permeable to ionic solutes, and the concentration of these ions is almost the same in ISF as in plasma. Cell membranes maintain intracellular solutes at very different concentrations from those of the ECF. The compositions of solutes in the ECF and ICF are compared in Figure 1-2, and concentrations of solutes in plasma and in ISF and ICF are listed in Table 1-1.
The slightly increased concentration of cations and anions in ISF compared with plasma water occurs primarily because of the presence of negatively charged proteins in plasma. The equilibrium concentrations of permeable anions and cations across the vascular endothelium are determined by the Gibbs-Donnan equilibrium, which occurs because negatively charged, nondiffusible proteins affect the distribution of other small charged solutes. In clinical practice, the difference in concentrations of anions and cations across the vascular endothelium is negligible, and the effects of the Gibbs-Donnan equilibrium are usually ignored. Thus, in clinical practice, plasma concentrations of solutes are considered to reflect solute concentrations throughout the ECF. Average values for plasma concentrations of important solutes in dogs and cats are given in Table 1-2.
Table 1-1 shows that, although the solute compositions of ECF and ICF are quite different, the total numbers of cations and anions in all body fluids are equal to maintain electroneutrality. The most abundant cation in the ECF is sodium (Na+). Most of the body Na+ is in the extracellular space. Approximately 70% of body Na+ in humans is exchangeable, and 30% is fixed as insoluble salts in bone.48 The percentage of exchangeable sodium is important because only exchangeable solutes are osmotically active. Cell membranes are permeable to Na+, which tends to diffuse into cells. In health, however, cell membrane sodium, potassium-adenosinetriphosphatase (Na+, K+-ATPase) actively removes Na+ from cells, thus maintaining a steep extracellular-to-intracellular concentration gradient for Na+. The ECF also contains a small but physiologically important concentration of K+. For example, alterations in ECF K+ concentrations may result in muscle weakness (hypokalemia) or cardiotoxicity (hyperkalemia). The most abundant anions in ECF are chloride (Cl−) and bicarbonate (HCO3−). The volume of distribution of Cl− is primarily the ECF volume. Bicarbonate is present in all body fluids and can be generated from CO2 and H2O in the presence of carbonic anhydrase.
In contrast to ECF, the primary cations in ICF are K+ and magnesium (Mg2+). Most of the body K+ is in the ICF, where K+ is the most abundant cation. Cell membranes are permeable to K+. The K+ concentration gradient between ICF and ECF is maintained by cell membrane Na+, K+-ATPase, which moves K+ into cells against a concentration gradient. The ratio of intracellular to extracellular K+ concentration is important in generating and maintaining the cell membrane potential at approximately −70 mV (see Appendix). Almost 100% of body K+ in humans is exchangeable.48 Unfortunately, a reliable, practical method for measuring the intracellular K+ concentration is not available, and changes in serum K+ concentration may not reflect changes in total body K+ stores (see Chapter 5). The predominant anions in the ICF are organic phosphates and proteins.
Units of measure
Atomic mass (also referred to as relative atomic mass or atomic weight)
Most naturally occurring elements consist of one or more isotopes of that element, each of which has a different mass. For example, carbon in the environment consists of approximately 99% 12C and 1% 13C. The atomic mass of an element is an average mass based on the distribution of stable isotopes for that element, and is determined by the weight of that element relative to the weight of the 12C isotope of carbon, which is defined as 12.000. Atomic mass usually is reported with no units or as atomic mass units. The atomic mass is shown in most periodic tables of the elements. The atomic weights of some biologically important elements in body fluids are listed in Table 1-3.
Molecular mass (molecular weight)
Many elements combine to form physiologically important compounds. The molecular mass of a compound is the sum of the atomic masses of the atoms that form the compound. For example, the molecular mass of water (H2O) is 18 and represents two times the atomic mass of hydrogen (2 × 1) plus the atomic mass of oxygen (16). The molecular weights of important compounds in body fluids are shown in Table 1-3.
Equivalent weight
Phosphate can exist in body fluids in three different ionic forms: H2PO4−, HPO42−, and PO43− (see Chapter 7). At the normal pH of ECF, approximately 80% of phosphate is in the HPO42− form and 20% is in the H2PO4− form. Therefore, the average valence of phosphate in ECF is 0.8 × (−2) + 0.2 × (−1) = −1.8. At a normal plasma phosphate concentration of 4 mg/dL, the phosphate concentration expressed in mEq/L would be:
Osmolality and osmolarity
In clinical medicine, osmolality is measured in serum, because the addition of anticoagulants for plasma samples would increase solute in the sample. Serum osmolality usually is measured by freezing-point depression, which is more precise and accurate than vapor pressure determinations. One osmole of a solute in 1 kg of water depresses the freezing point of the water by 1.86° C.55 Average values for measured serum osmolality in the dog and cat are 300 and 310 mOsm/kg, respectively.8,17 Measured osmolality may not be the same as calculated osmolality (see later discussion).
Effective and Ineffective Osmoles
In any fluid compartment, the osmotic effect of a solute is in part dependent on the permeability of the solute across the membranes separating the compartment. Consider the two fluid compartments in a rigid box in Figure 1-3. Assume that the membrane dividing the two compartments is freely permeable to urea and water but is impermeable to glucose. When urea is added to the left compartment (top of figure), it moves down its concentration gradient from left to right, and water moves down its concentration gradient from right to left until there are equal concentrations of urea and water on both sides of the membrane. No fluid rises in the column attached to the left fluid compartment because urea is an ineffective osmole and does not generate osmotic pressure. In biologic fluids, urea is a small molecule that freely diffuses across most cell membranes and therefore does not contribute to effective osmolality.
Calculated Osmolality
Concentration of 0.9% NaCl: | 0.9 g NaCl/100 mL of solution = 900 mg NaCl/dL |
Convert milligrams to grams and deciliters to liters | 900 mg NaCl/100 dL × 1 g/1000 mg × 10 dL/L = 9 g NaCl/L |
Formula weight of NaCl: (use atomic weight from Table 1-3 or periodic table) | Atomic mass of Na + atomic mass of Cl = 23 + 35.5 = 58.5 |
Molar mass of NaCl: | 58.5 g |
Convert grams to moles: | 9 g NaCl × (1 mol/58.5g) = 0.154 mol NaCl |
Convert moles to millimoles: | 0.154 mol × (1000 mmol/mol) = 154 mmol NaCl |
Determine millimoles of Na+ and Cl− | NaCl in solution dissociates into Na+ and Cl−, yielding 154 mmol/L of Na+ and 154 mmol/L of Cl− |
Determine milliequivalents of Na+ and Cl− | millimoles × valence = milliequivalents Na+ and Cl− each have a valence of 1 154 mmol ×1 = 154 mEq of Na+ 154 mmol ×1 = 154 mEq of Cl− |
Determine milliosmoles of Na+ and Cl− | NaCl in solution dissociates into Na+ and Cl−, so the mOsm/L in 0.9% NaCl is the sum of the mOsm for each component: 154 mEq/L Na+ + 154 mEq/L Cl− 154 mOsm/L Na+ + 154 mOsm/L Cl− = 308 mOsm/L |
Concentration of 10% CaCl2: | 10 g CaCl2/100 mL of solution = 10 g CaCl2/dL |
To convert deciliters to liters: | 10 g CaCl2/dL × 10 dL/L = 100 g CaCl2/L |
Formula weight of CaCl2: (use atomic weight from Table 1-3 or periodic table) | Atomic mass of Ca + 2 × (atomic mass of Cl) = 40.1 + (2 × 35.5) = 111.1 |
Molar mass of CaCl2: | 111.1 g |
Convert grams to moles: | 100 g CaCl2 × (1 mol/111.1 g) = 0.9 mol CaCl2 |
Convert moles to millimoles | 0.9 mol × (1000 mmol/mol) = 900 mmol CaCl2 |
Determine millimoles of Ca+2 and Cl−: | CaCl2 in solution dissociates into Ca+2 and 2Cl, yielding 900 mmol/L of Ca+2 and 1800 mmol/L of Cl− |
Determine milliequivalents of Ca+2 and Cl− | millimoles × valence = milliequivalents Ca+2 has a valence of 2; Cl− has a valence of 1 900 mmol Ca+2 × 2 = 1800 mEq of Ca+2 1800 mmol Cl− × 1 = 1800 mEq of Cl− |
Determine milliosmoles of Ca+2 and Cl−: | CaCl2 in solution dissociates into Ca+2 + 2Cl− mOsm/L in 10% CaCl2 is the sum of the milliosmoles for each component: 1800 mEq/L Ca+2 + 1800 mEq/L Cl− 900 mOsm/L Ca+2 + 1800 mOsm/L Cl− = 2700 mOsm/L |
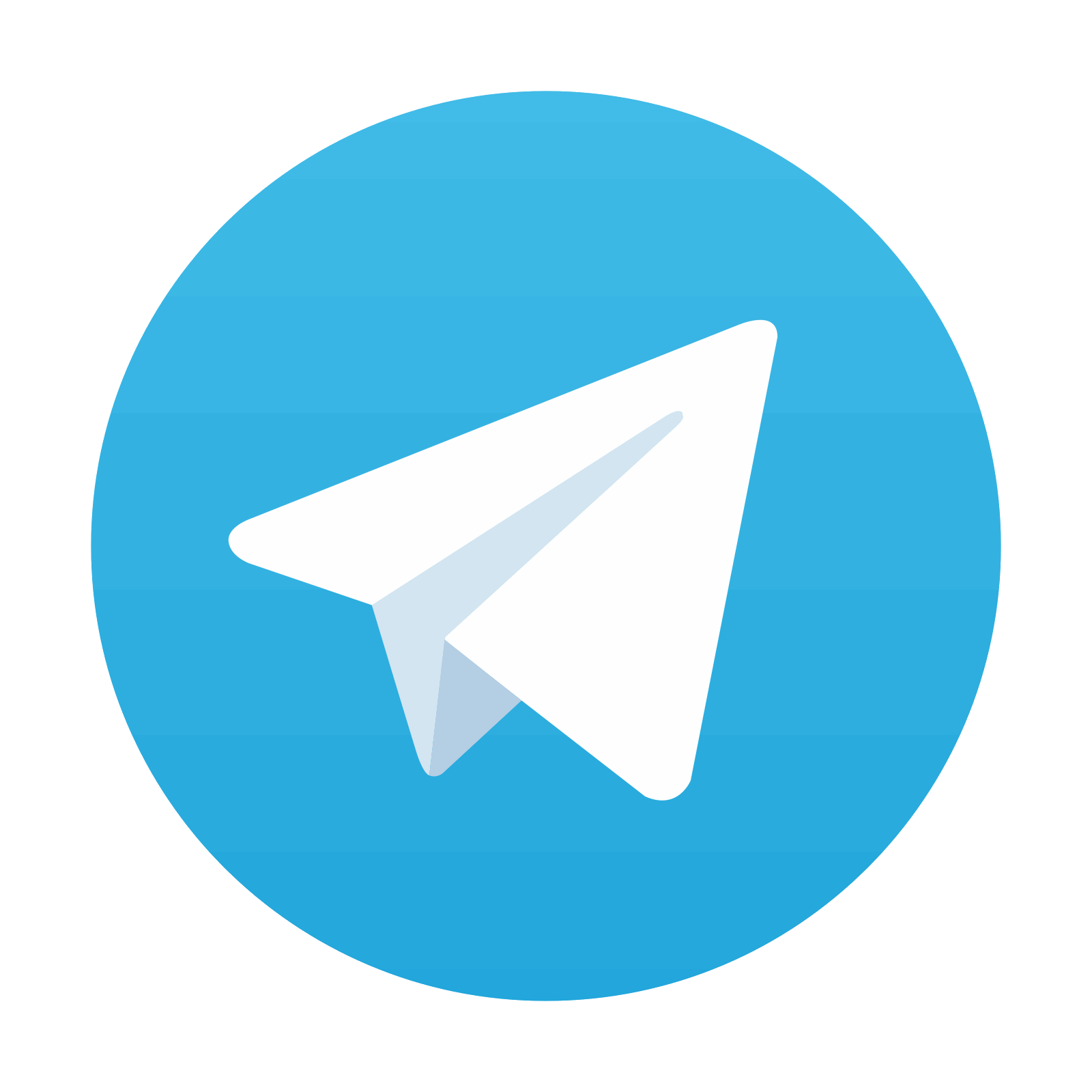
Stay updated, free articles. Join our Telegram channel

Full access? Get Clinical Tree
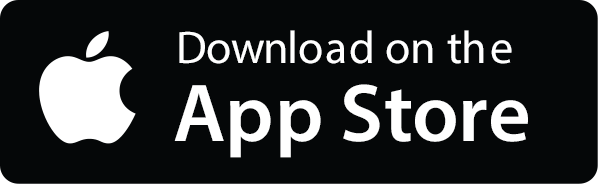
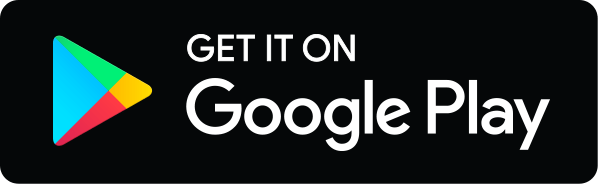