Chapter 31 Adverse Food Reactions
Definition
An adverse food reaction is defined as any abnormal clinical response that occurs following ingestion of a food or food component. Adverse food reactions have been classified according to the underlying pathophysiologic mechanism. These classifications vary from highly specific mechanisms to broad categories. The classification presented in Box 31-1 is based on the mechanism of the reaction, but it should be recognized that in the vast majority of cases, the mechanism of the adverse reaction to food remains undetermined. Thus diagnostic and management principles apply to all clinical cases unless a specific mechanism is identified.
Box 31-1
Mechanistic Classification for Adverse Reactions to Food
The prevalence of adverse food reactions in dogs and cats has not been precisely determined. In a study of 55 cats with chronic signs of gastrointestinal disease (vomiting and/or diarrhea), clinical signs resolved in 27 (49%) of the cats fed an elimination diet.1 Of those 27 cats, 16 (29%) relapsed when challenged with the original diet. Similar studies have not yet been published in dogs. However dogs with acute vomiting and/or diarrhea are frequently seen in primary practice, and adverse reactions to food are commonly suspected (Box 31-1).
Mechanism and Pathophysiology
Nonimmunologic Adverse Reactions to Food (Food Intolerance)
Nonimmunologic adverse food reactions refer to those in which an innate or acquired immunologic response is not the underlying mechanism for clinical signs. The term food intolerance has often been used to describe all nonimmunologic adverse reactions to food. Food “additives” are frequently thought to be responsible for intolerance reactions in dogs and cats. A food “additive” is generally defined as anything that is not directly nutritive but imparts increased nutritional, gustatory, or cosmetic appeal. These include preservatives, humectants (hygroscopic substances), emulsifying agents, flavors, gelling agents, and colors.2 Despite the wide range of compounds used in commercial pet foods under this term, and in contrast to popular opinion, there is little evidence that food additives are a cause of gastrointestinal disease in dogs and cats.
Food Toxicity
Food toxicity, or food poisoning, generally refers to reactions caused by microbial contamination, but could also include toxins such as benzoic acid or propylene glycol, which have been used in some pet foods. Food toxins include those incorporated into the manufacturing process, and those produced during storage as the result of microbial spoilage. Several mycotoxins have been identified in commercial foods, with corn, grain, and their by-products most commonly incriminated. Aflatoxins (produced predominantly by Aspergillus spp.) primarily cause acute hepatocellular necrosis, and dogs or cats may present with anorexia, vomiting, diarrhea that can progress to melena, and other signs of acute hepatopathy.3,4 Deoxynivalenol (DON, also endearingly termed “vomitoxin”) is the most prevalent mycotoxin produced by Fusarium spp. Dogs and cats are very sensitive to DON contamination, leading to anorexia, vomiting, bloody diarrhea, and leukopenia, signs that could be easily confused with those of parvoviral enteritis.5 Enterotoxigenic strains of bacteria can contaminate food and produce acute enteritis, including Escherichia coli, Staphylococcus pseudointermedius, and potentially Clostridium spp. In the majority of these cases, there is usually a clear association between ingestion of a particular food substance and the onset of clinical signs, but pet owners may not present affected animals unless the clinical signs are serious or persistent.
Alterations in the Microflora
Ingested food has a profound effect on the number, species, and metabolic activity of the intestinal microflora. Abrupt dietary change is a common cause of diarrhea, and occasionally vomiting. Most cases are mild and self-limiting, although some will persist until the animal has returned to regular feedings. Persistence of clinical signs following dietary change is reason to suspect a food hypersensitivity or persistent intolerance, but many short-term cases are probably the result of induced disturbances in the enteric microflora. Nondigestible carbohydrate and protein serve as substrates for the microflora, but bacterial species vary greatly in their ability to ferment dietary compounds. In humans, changing from a conventional diet to a highly digestible, fiber-free liquid enteral diet reduces fecal bacterial numbers on a dry weight basis, as well as the fecal concentration of short-chain volatile fatty acids (acetate, propionate, butyrate).6 The inclusion of fermentable fiber into liquid enteral diets provides substrate for distal ileal and colonic bacteria, and reduces the effect of shifting to highly digestible liquid enteral diets.
In addition to the effect of diet on total bacterial numbers, diet also has an important effect on microbial distribution. Dogs fed a high-protein canned diet have increased numbers of fecal Clostridium spp. and reduced numbers of fecal bifidobacteria when compared with dogs fed a lower-protein dry diet, although diet had no effect on fecal Clostridium perfringens enterotoxin detection.7 Human patients placed on a fiber-free liquid enteral diet experienced varied and inconsistent alterations in their fecal microflora.8 Patients who subsequently developed diarrhea during enteral feeding had higher total numbers of fecal Clostridium spp. and lower numbers of Bifidobacterium spp. Bifidobacteria have been shown to exert antimicrobial activity against many enteropathogens. Specific strains of bifidobacteria isolated from human feces inhibit the growth of Salmonella spp., E. coli, Klebsiella pneumoniae, and Staphylococcus aureus in vitro, and significantly reduce mortality when gnotobiotic mice are infected with Salmonella typhinurium.9
Similar mechanisms have been proposed to underlie most cases of antibiotic-associated diarrhea. Changes in the enteric microflora are associated with decreased fecal short-chain fatty acid concentrations and decreased concentrations of bifidobacteria species, both of which return to normal following antibiotic withdrawal.10 Disruption of the commensal population following antibiotic therapy permits pathogen proliferation, which could cause diarrhea through toxin production.
Sources of fermentable carbohydrate have been shown to affect numbers of mucin-degrading bacterial species, which are associated with increased mucosal permeability and diarrhea.11 A reduction in the specific strains of resident microflora, may reduce the overall pathogen inhibition effect, which then increases the risk of colonization with enteropathogens such as Clostridium difficile or C. perfringens. Substantial individual variability is seen in the susceptibility to dietary change. One of several explanations is the variability in an individual’s microflora, and even within similar compositions there is variation. For instance, not all Bifidobacterium strains possess antibacterial activity, and this contributes to individual variability in response to dietary changes.
Dysmotility
Foods that prolong gastric retention, especially high-fat diets and highly viscous diets (soluble fiber), or diets that contain poorly digestible starch may promote vomiting in susceptible patients.12–14 Predictably, gastric retention times increase with increasing meal size, but also with increased dry matter content.15 In humans with functional dyspepsia, dietary fat is frequently incriminated as an exacerbating and potentially causative factor leading to an exaggerated sense of fullness, nausea, and vomiting.16 This is supported by studies that demonstrate symptoms after duodenal lipid infusions, but not after isoenergetic infusions of glucose.17
Studies of dietary influences on colonic motility have largely centered on dietary fiber, and their fermentation by-products, the short-chain fatty acids. Constipation is a relatively common presentation, and in the absence of a mechanical obstruction, can develop as a result of excessive dehydration and impaired motility. Dietary variables that lead to constipation have not been well defined in dogs or cats. There is a clear association with the consumption of indigestible material such as bone or wool.18 In normal humans, colonic transit time is decreased as insoluble fiber is increased, whereas in patients with chronic constipation, increasing insoluble fiber does not speed fecal transit.19 Whether that reflects a cause or effect is uncertain, as is the significance to canine and feline patients. It is likely that colonic transit time partly determines the response to dietary manipulation, as it does in people.20 However, determination of colonic motility in dogs and cats is somewhat difficult (see Chapter 26), and there is no definitive evidence for recommending one intervention over another.
Insoluble, nonfermentable fiber increases fecal bulk and increases the frequency of defecation in healthy animals. Increasing fecal bulk may exacerbate constipation in an individual with impaired colonic motility. Perhaps the poorest choice of dietary fiber in constipation is a nonfermentable, insoluble fiber that increases fecal dry matter, but not fecal water content, such as cellulose.21 Fiber that produces viscous gels (e.g., psyllium husk) will increase the fecal water content, in addition to increasing fecal dry matter. Short-chain fatty acids from colonic fermentation have been shown to stimulate longitudinal colonic smooth muscle contractions in vitro in kittens and adult cats.22 However highly fermentable fiber may also result in the production of methane. Methane production varies greatly between individuals and depends on the presence of specific organisms.23 Physiologic concentrations of methane slow small intestinal transit by augmenting circular muscle contractions in the ileum.24 Constipation, small intestinal dysmotility, and discomfort are aggravated in human irritable bowel syndrome patients with methane production.24,25 Similarly, the induction of nonpropulsive segmental contractions by methane may be a cause of motility dysfunction in dogs and cats. Consequently, the supplementation of diets with rapidly fermentable purified fiber sources, such as hydrolyzed guar gum, may exacerbate some cases of constipation, irritable bowel syndrome, and other motility disorders.
Pharmacologic Reactions
Methylxanthines
Theobromine and caffeine toxicity is well recognized in dogs. Most dogs will present with signs relating to central nervous system and cardiovascular effects. Anxiety, aggression, tremors, and tachycardia are commonly reported.26 In other cases, vomiting and diarrhea are more prominent clinical signs, but still usually accompanied by concurrent neurologic signs.27
Histamine
Histamine is found in various home-prepared and commercial foods. Higher concentrations have been detected in foods containing fish by-products, although ingredient lists are not always complete.28–30 Histamine and other decomposition products are produced in raw fish by bacterial conversion of free histidine. Scombroid fish (e.g., tuna, mackerel, skipjack, bonito) have higher amounts of histamine because of the free histidine content in their muscles.31 Adverse reactions to the ingestion of raw anchovies that was responsive to antihistamine administration has been reported in cats, and similar signs have been reported in dogs.30 In those cases, salivation, vomiting, and diarrhea developed within 30 minutes of ingestion.
Some commercial foods contain concentrations of histidine equal to that of raw fish that, if spoiled, might result in high histamine concentrations. That said, the concentration of preformed histamine in unspoiled commercial foods is not that high.29–31 Ingested histamine is rapidly metabolized by intestinal diamine oxidase (DAO). There is great variation in DAO activity within the human population, rendering some individuals very sensitive to dietary histamine content.32 Variation in intestinal DAO activity in dogs and cats has not been reported, but large differences in plasma DAO activity do exist in cats.33 Failure to degrade ingested histamine can result in vomiting and diarrhea that can be hemorrhagic, along with other systemic effects.32,34
It has been shown that several drug classifications are capable of profoundly inhibiting the activity of porcine and human intestinal DAO, including the β-lactamase inhibitor clavulanic acid.34 The clinical significance of this is unknown, but it is conceivable that normally tolerated concentrations of dietary histamine may be sufficient to cause clinical signs when the capacity for intestinal metabolism is reduced during oral treatment with clavulanate.
Maldigestion and Malabsorption
Undigested dietary components can lead to osmotic diarrhea, and rapid fermentation in the distal intestine can lead to flatulence, abdominal pain, and vomiting. Lactose intolerance is the quintessential example of a maldigestive adverse food reaction. Intestinal lactase expression decreases rapidly around the time of weaning in most mammals. In kittens, lactase activity decreases almost tenfold between birth and 6 weeks of age.35 However, expression in adult cats is highly variable, explaining large individual differences in the ability of the cat to tolerate dietary lactose. Fasting also significantly reduces the expression and specific activity of mucosal enzymes such as disaccharidases, which can lead to impaired digestion following the reintroduction of food.36 Transient lactase deficiency is common, particularly after rotaviral gastroenteritis.37 Occasionally it persists, and lactose intolerance may be a cause of postgastroenteritis diarrhea. Undigested lactose can lead to diarrhea by a direct osmotic effect. In addition, colonic and distal small intestinal bacteria rapidly ferment luminal lactose producing gas and volatile fatty acids that can worsen diarrhea, abdominal bloating, borborygmus, and painful intestinal dilation. Healthy adult dogs may tolerate up to 2 g/kg body weight (BW) of lactose, whereas adult cats will not tolerate more than 1 g lactose/kg BW without showing clinical signs.38
High-fiber diets may have an effect similar to lactose intolerance in sensitive individuals. High-fiber diets increase gas production by colonic flora and inhibit gas transit leading to gas retention, notable borborygmus, abdominal pain, and flatulence.39 Ingestion of a “fiber-free” diet for 48 hours significantly reduces the total volume of flatus.40 Highly purified, highly fermentable fibers such as xylan and pectin predictably cause a greater production of hydrogen, carbon dioxide, and methane than does cellulose or corn bran.41 The amount of fermentable fiber that can be tolerated without adverse effects varies between individuals, and probably reflects differences in colonic transit time, as well as differences in intestinal microflora.42
Physical and Other Effects
Commercial pet foods and most home-prepared diets are unlikely to cause physical irritation, damage, or motility problems in the gastrointestinal tract. However, feeding of fresh carcass meat and “home-kill” often results in the ingestion of significant amounts of bone, wool, and hair, which can predispose to constipation, as well as acute colitis.43 Dietary indiscretion can result in the ingestion of toxins, indigestible physical abrasives, excessive fat, and compounds that can cause significant gastric fermentation.
Nonspecific Dietary Sensitivity
The term nonspecific dietary sensitivity has been used to describe animals that have loose or poorly formed feces when fed particular types of commercial diets.44,45 The most common cases are seen in large-breed dogs, although the phenomenon can also be seen in small dogs and cats. It has been suggested that reduced colonic capacity for fluid and electrolyte absorption in large-breed dogs makes at-risk individuals susceptible to high-moisture diets.46 Alternatively, rapid intestinal transit may lead to a degree of malabsorption.45 Only very mild lymphocytic infiltrates are seen in the small intestine of such animals, and it is not clear if these cases are truly a form of inflammatory bowel disease. To date, abnormal microflora populations have not been shown to be present, and although treatment of affected dogs with the probiotic Lactobacillus acidophilus strain DSM 13241 led to clinical improvement in one study, the fecal density of cultured bacteria such as C. perfringens and E. coli were not significantly altered.47 In summary, the mechanism of the reaction to food is unclear in these cases, although they will rapidly and readily respond to appropriate dietary manipulation (see below).
Immunologic Adverse Reactions to Food
Complex foods contain a myriad of different proteins, the great majority of which are potentially antigenic, and in a lifetime, an animal will ingest many kilograms of these foreign antigens. Luminal antigens are presented to the largest single collection of T lymphocytes in the body, separated by an epithelial layer within which intraepithelial lymphocytes are directly juxtaposed to this antigen mass. Intact antigens are directly sampled from the intestinal lumen, and a small fraction can even be found in the systemic circulation following a meal.48 For these reasons, it is perhaps more surprising that detrimental immune responses to dietary proteins do not more commonly occur.
Immune Responses to Dietary Antigens
Peyer’s patches are the primary inductive area of the intestinal immune system. Specialized M cells within the epithelium sample particulate and insoluble antigens, and whole microorganisms.49 Antigens and organisms are then transported to B cells, macrophages, and dendritic cells within basement membrane invaginations. In the normal intestine these antigen-presenting cells (APCs) lack costimulatory molecules such as CD80 and CD86. Antigens processed by these “unactivated” APCs are then presented to naive B and T cells within the follicle, resulting in the induction of hyporesponsive, T-helper (Th) type 3- or Th2-biased T cells.50 Activated cells then leave via lymphatics and pass via the mesenteric lymph nodes into the systemic circulation. They then exit at mucosal sites via engagement of cellular adhesion molecules (CAMs) specifically expressed by the high-endothelial venules of mucosal tissues. Activated or memory B and T lymphocytes enter the lamina propria to await a secondary encounter with their specific antigen (Fig. 31-1).
It is important that the immune system reserves the ability to rapidly respond to pathogens. This ability to recognize pathogenicity is based on the engagement of pathogen-associated molecular pattern (PAMP) receptors, such as Toll-like receptors (TLRs), producing “danger signals.” Predictably, expression of TLRs is much greater in mesenteric lymph nodes than in the small intestine, which is generally higher than the large intestine where there is increased potential for deleterious activation by normal microflora.51 The expression of TLRs is generally lower in the mucosal cells of the normal canine intestine than in the intestines of dogs with inflammatory bowel disease (IBD) or diet-responsive enteropathies, but expression can be rapidly turned on in response to inflammatory cytokines.51 The absence of these “danger signals” results in relatively inefficient antigen processing by intestinal APCs, markedly reduced or absent tumor necrosis factor (TNF)-α/IL-1/IL-12 production, and the absence of CD80/86 costimulatory molecule expression. T cells activated by such an APC, divide less, with most clones undergoing early deletion by apoptosis, while the surviving memory cells tend to secrete IL-10, TGF-β, or no cytokines.52 This combination of apoptosis, functional defects in surviving clones, and T cells secreting the antiinflammatory and IgA-supporting cytokines, is the general basis for immunologic tolerance to luminal antigens (Fig. 31-2).
Food Immunogenicity
In mammals, intact particulate and insoluble antigens are preferentially absorbed across the intestine through M cells overlying the Peyer patches.53 Classically, such antigens tend to invoke active immunity appropriate for microorganisms. In contrast, soluble antigens are associated with oral tolerance.54 It has also been shown oral tolerance can be abrogated when soluble proteins are fed in oil-in-water emulsions, resulting in robust systemic humoral responses.55 This effect may also have relevance to the pet food industry where interactions between dietary proteins and lipids in canned or extruded diets during the cooking and the manufacturing process could feasibly result in novel interactions not present in their native states.
In chickens, particulate antigens induce tolerance, whereas soluble antigens provoke active immunity.56 If the physical nature of the proteins within the natural diet of a species dictates how the intestinal immune system has evolved, this might have special relevance to species that are commonly fed diets different from their ancestors.
Commercial pet foods are subjected to significant heating during the manufacturing process. The effect of heat treatment on proteins is mostly to change the three-dimensional structure of the protein. Although this may disrupt some antigens, it may equally uncover previously hidden or create new antigenic determinants. Other reactions occurring at high temperatures include the Maillard reactions, which involve the reactions between certain amino acids and reducing sugars to produce less-digestible compounds called melanoidins, which give a characteristic brown color. Melanoidins tend to be less digestible and less soluble, and certain melanoidins are more “allergenic” than the original uncooked protein.57,58
The effect of heating during the canning process on the immunogenicity of dietary proteins has been evaluated in cats.59 Using soy and casein proteins, the canning process resulted in the creation of new antigens not present in the uncooked product. In addition, a product of heated casein induced a salivary IgA response that was not induced by the raw product. Thus commercial food processing can qualitatively and quantitatively alter the immunogenicity of food proteins. As obligate carnivores, felids have evolved on a highly digestible diet.60 In keeping with this is the relatively short intestinal tract of the cat, which suggests that they may be poorly suited to poorly digestible diets. It is well established that the commercial canning process decreases protein digestibility and that this has biologically significant effects in cats.61 Although the significance of this finding is uncertain at present, it emphasizes the need for feeding highly digestible protein sources, or perhaps even more so, hydrolyzed proteins, when enteritis is present.
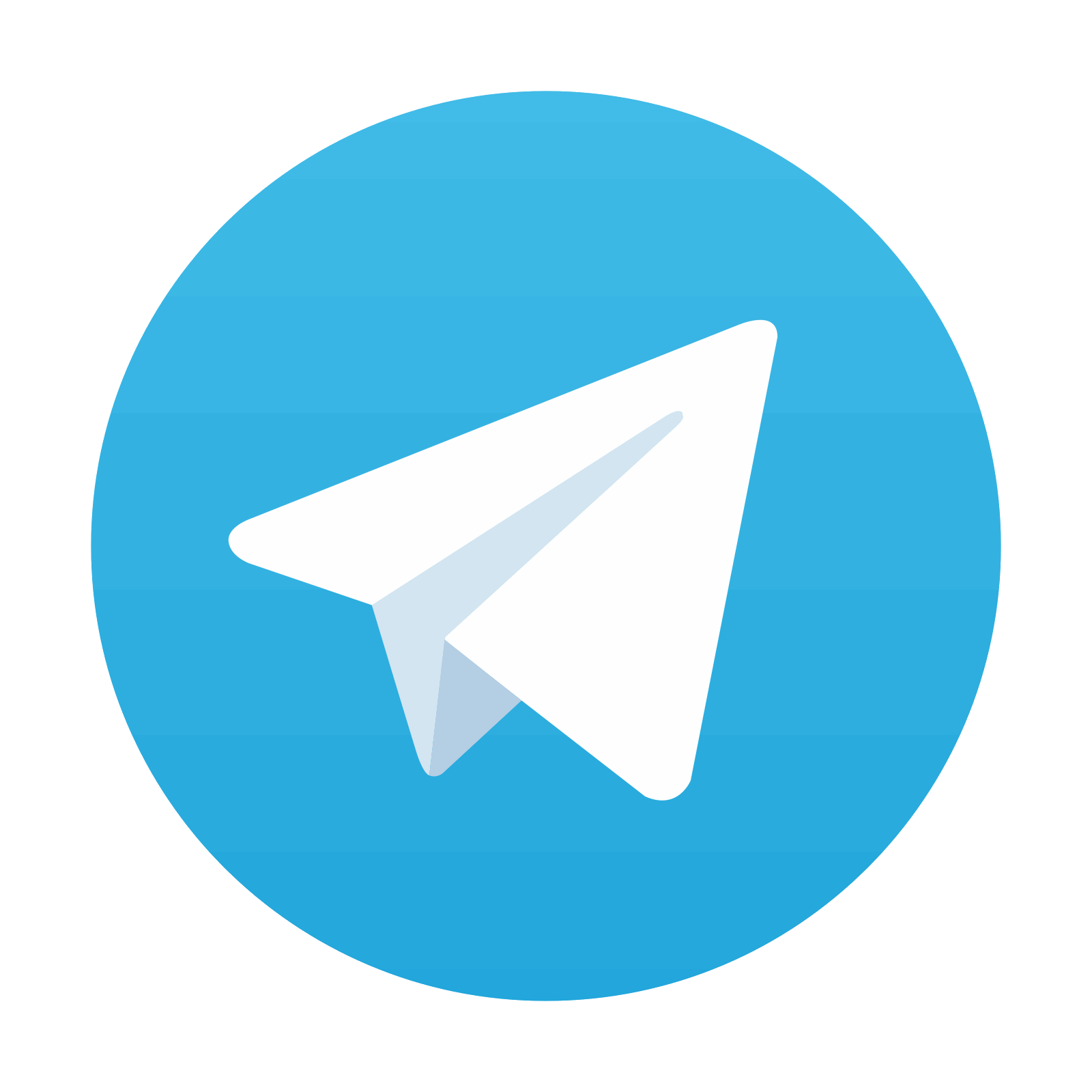
Stay updated, free articles. Join our Telegram channel

Full access? Get Clinical Tree
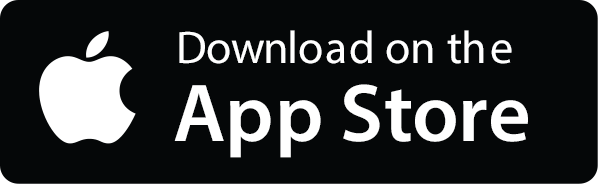
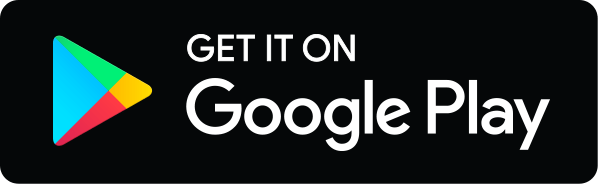