CHAPTER 17 Advanced Diagnostic Techniques
Cytopathology is a highly useful, noninvasive method for diagnosis of malignant vs. benign conditions and identification of infectious agents. However, a number of problems confront the cytopathologist on a daily basis because of limitations of conventional cytologic features. Adjunct techniques can be utilized to give additional information, which allows one to reach a definitive diagnosis. These techniques must be used in parallel with conventional cytologic features and include immunodiagnostics for cellular origin, electron microscopy for presence of subcellular structures, special histochemical stains for demonstration of chemical constituents, flow cytometry and image analysis for a quantitative evaluation of cellular markers, and molecular diagnostics for clonality or chromosomal abnormalities. In this chapter, we discuss adjunct diagnostic techniques and focus on both their applications in cytopathology and histopathology.
IMMUNODIAGNOSIS
The detection of antigens by immunologic and chemical reactions in tissue sections (immunohistochemistry-IHC-), or cytologic preparations (immunocytochemistry-ICC-) has become one of the most commonly used ancillary morphologic techniques in diagnostic pathology (Barr and Wu, 2006). The advantages of IHC and ICC are: 1) They do not require the use of expensive equipment. 2) Both prospective and retrospective studies can be done on a variety of samples. 3) Antigen detection can be correlated with morphologic changes (IHC) and its cellular location (ICC). 4) Stained slides can be stored for many months. 5) Routine processing of samples is usually acceptable for these techniques. Both IHC and ICC are practical in the characterization of poorly differentiated neoplasms, differentiation of primary from metastatic tumors, and determination of sites of origin of metastatic lesions and prognostic assessments (DeLellis and Hoda, 2006). The general consensus is that IHC/ICC methods, if properly applied and interpreted, increase diagnostic accuracy in pathology. Technical aspects of IHC and ICC, interpretation of results, and pitfalls will be reviewed. An algorithmic approach to the diagnosis of tumors, the diagnosis of metastatic disease, and the use of antibodies as prognostic markers will be presented. This review will not include detailed IHC or ICC procedures. For this purpose, the reader is referred to other published material (Polak and Van Noorden, 2003; Ramos-Vara and Saeteele, 2007).
Immunohistochemistry
Antibodies
Immunohistochemistry (IHC) demonstrates antigens in tissue sections by incubating the sections with specific antibodies and demonstrating the immunologic reaction with a histochemical (enzyme-substrate) reaction to produce a colored (visible) reaction (Ramos-Vara, 2005). Polyclonal or monoclonal antibodies can be used. In general, polyclonal antibodies are usually raised in rabbits and have higher affinity but lower specificity than monoclonal antibodies. Cross-reactivity (defined as recognition of unrelated antigens) is more common with polyclonal antibodies. Key in the use of polyclonal antibodies in diagnostic IHC/ICC is their degree of purification (examples of commercially available antibodies include whole serum antibodies, antibodies purified by precipitation of immunoglobulins, and immunoglobulins purified by affinity chromatography). Monoclonal antibodies, produced in mice using the hybridoma technology, recognize a single epitope (a 4-8 amino acid chain in a protein) and therefore are highly specific and have constant characteristics among different batches of antibody. Rabbit monoclonal antibodies are increasingly being used in human diagnostic IHC, but despite their reported advantages over mouse monoclonal antibodies (e.g., higher affinity, no need for antigen retrieval, use on mouse tissues), some of them neither react on animal tissues nor perform better than mouse monoclonal antibodies (Reid et al., 2007; Vilches-Moure and Ramos-Vara, 2005). Selection of a particular antibody will be determined by published information or the experience of other laboratories. There are no guarantees that an antibody that recognizes an antigen in one species will do so in another species; only testing will determine if this is the case. Needless to say, the large number of species from which samples can be obtained is one of the biggest challenges that a veterinary pathologist must face in immunodiagnostics.
Fixation
The universal fixative for histopathology and diagnostic IHC is buffered formalin. Attempts to replace formalin fixative in diagnostic IHC have failed, although for specific situations the use of nonformaldehyde fixatives, particularly glyoxal-based, has been reported (Yaziji and Barry, 2006). Fixation is necessary for preservation of cellular components, to prevent autolysis and displacement of cell constituents, to stabilize cellular materials (antigens), and to facilitate conventional staining and immunostaining (Ramos-Vara, 2005). The use of formalin is not without problems. First, the quality of formalin solutions varies widely in regard to concentration of formaldehyde, pH, and presence of preservatives. Second, formalin fixation, by producing methylene bridges between amino groups and other functional groups, alters the tertiary and quaternary structure of proteins and forms cross-links between soluble tissues and proteins. These chemical reactions may modify the targeted epitope. Amino acids that are especially sensitive to formalin fixation include lysine, glycine, tyrosine, arginine, histidine, and serine. Despite the fact that formalin fixation may impair immunohistochemical detection, good fixation is paramount to detect antigens with IHC. Underfixation is as bad as or worse than overfixation, and is a fairly common problem due to reduced turnaround times in diagnostic laboratories. With the advent of heat-induced epitope retrieval (HIER), overfixation or variable fixation time among samples is less critical in the detection antigens targeted in human diagnostic IHC (Webster et al., 2009). The same will probably be true with common antigens demonstrated in animal samples. Autolysis is a common problem in diagnostic pathology. Studies addressing the effects of autolysis in IHC have shown that most antigens are still detectable despite decomposition; however, caution in the interpretation of autolyzed material is necessary due to the loss of detection of some antigens (Maleszewski et al., 2007). Necrotic tissue tends to produce more background than normal tissue; however, IHC of necrotic tissue can provide valuable information when no other tissue is available, particularly for cytokeratins and CD45. Decalcification of formalin-fixed tissues generally does not reduce the immunoreactivity of most antigens, particularly when using weak acids; some loss of reactivity is apparent when using strong acids for decalcification, but it does not affect all antigens.
Sample Processing
Processing of samples for diagnostic IHC is the same as for routine histopathology. Antigens have been successfully detected in formalin-fixed, paraffin-embedded (FFPE) tissues stored for several decades (Litlekalsoy et al., 2007). Autolyzed samples or those with biopsy artifacts should be avoided. For IHC and ICC, samples are mounted onto silanized slides, poly-L-lysine-coated slides, or charged slides to allow a strong bond between the slide and the tissue section. Pooling of reagents under the tissue section or tissue loss can occur when using noncharged slides or slides without special coatings. Complete deparaffination is critical to achieve optimal immunostaining. Deparaffination is somewhat cumbersome and there are commercial products to perform deparaffination and antigen retrieval simultaneously although results may not be completely satisfactory. A simple approach to deparaffination and antigen retrieval with heat has recently been published (Boenisch, 2007).
Protocols
For technical aspects of IHC and detailed protocols, the reader is referred to a more recent review (Ramos-Vara and Saeteele, 2007). Table 17-1 includes the antibodies used by the Animal Disease Diagnostic Laboratory at Purdue University for infectious and neoplastic diseases of dogs and cats. Immunohistochemical protocols can be divided into three stages: 1) Pretreatment procedures; 2) Incubation of the primary antibody, secondary, and tertiary reagents; 3) Visualization of the immunologic reaction.
TABLE 17-1 List of Selected Antigen Markers, Sources, Tissue Controls, and Uses for Selected Antibodies Used in Dogs and Cats
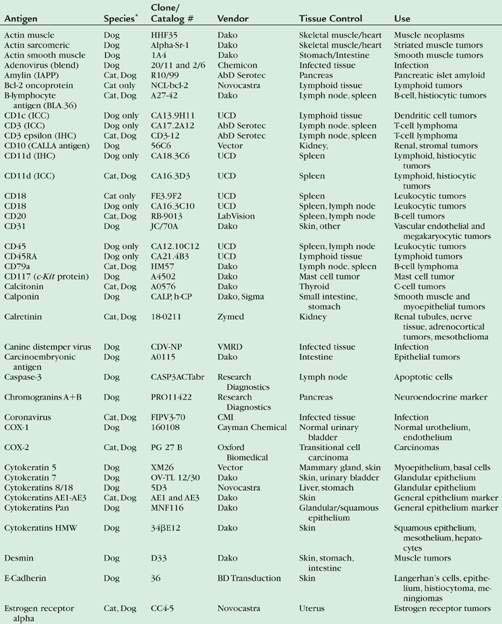
Pretreatment Procedures
These procedures include blocking of endogenous activities, blocking of nonspecific binding, and antigen retrieval (the lattermost already discussed). Endogenous peroxidase (for immunoperoxidase procedures) is common in numerous tissues although formalin fixation destroys most of it. Endogenous alkaline phosphatase (for alkaline phosphatase detection methods) is blocked in procedures using this enzyme. Mammalian tissues have two alkaline phosphatase isoenzymes; the nonintestinal form is easily blocked with levamisole; the intestinal isoform unfortunately requires acetic acid to be blocked, a chemical that can damage some antigens. Numerous tissues have endogenous avidin-biotin activity that must be blocked before adding biotinylated reagents in avidin-biotin detection systems. Nonspecific binding of immunoglobulins to tissue is blocked by incubating tissue sections with bovine serum albumin or serum from the same species as the secondary reagent before the incubation with the primary antibody. There are commercially available reagents to block endogenous activities and nonspecific immunoglobulin binding.
Immunohistochemical Reaction
The immunohistochemical reaction can be divided into an immunologic (antigen-antibody) reaction followed by its demonstration with a histochemical (colored) reaction. The sensitivity of the immunohistochemical reaction is mostly the result of the detection method used (Ramos-Vara, 2005); progress in this regard has been dramatic in the last decade. Two main enzymes are used in IHC: peroxidase and alkaline phosphatase. Peroxidase is probably the enzyme most commonly used, but in some occasions, particularly with heavily pigmented samples or samples rich in endogenous peroxidase, alkaline phosphatase is an excellent alternative. Current IHC methods can be divided into avidin-biotin or non–avidin-biotin systems. After incubation with the primary antibody, a secondary antibody specific for the primary antibody (secondary reagent) is added. For avidin-biotin systems the secondary reagent is biotinylated. For avidin-biotin methods, a tertiary reagent labeled with avidin molecules and an enzyme (peroxidase or alkaline phosphatase) is needed. The most common non–avidin-biotin method is based on polymer technology. The polymers contain many molecules of secondary antibodies and enzyme. Polymer methods are usually two-step methods, whereas avidin-biotin methods are usually three-step methods. Polymer-based methods have fewer steps, do not have endogenous avidin-biotin background problems, and are usually more sensitive, but are beyond the scope of this review (Vosse et al., 2007). Detection of multiple antigens in the same tissue section is also possible. Issues to keep in mind in double or multiple immunostaining is the compatibility of AR among antigens to be detected, the type of primary antibodies (polyclonal or monoclonal), cellular localization of antigens, and the color of chromogens used.
Standardization and Validation of an IHC Test
Like any other ancillary technique, IHC needs to be standardized and validated. Optimization (standardization) of a new antibody/test is the process of serially testing and modifying components of the procedure (e.g., fixation, antigen retrieval, antibody dilution, detection system, incubation time, etc.) with the aim of producing a consistent, high-quality assay. The reader is advised to standardize every antibody used in his/her laboratory despite the existence of published protocols, to ensure optimal results. Standardization includes adequate tissue fixation. Tissues should be fixed in 10% neutral buffered formalin for a minimum of 8 hours. Every new antibody is tested following a standard protocol that includes three pretreatments: no antigen retrieval, AR with a proteolytic enzyme (e.g., proteinase K), and HIER (e.g., citrate buffer, pH 6.0); and four, two-fold dilutions of the primary antibody (Ramos-Vara and Beissenherz, 2000). With this standard protocol, the total of slides initially processed for each antibody is 15, including a negative reagent control for each pretreatment. The positive control section used in standardization (and later in a diagnostic setting) is one in which the antigen in question has been detected with a different method (e.g., virus isolation) and its cellular location is known. A negative control section (containing cells known by independent methods to lack the antigen in question) also should be included. Usually, the same tissue block used for the positive control can be used for the negative control.
Test validation in IHC follows standardization; however, because it is time consuming and expensive, it is seldom done in veterinary medicine. Validation of a test examines technical aspects such as the effects of prolonged fixation, but focuses more on the ability of the antibody to be used as a marker of a specific cell, tumor, or infectious agent. Antibodies used as tumor markers need to be tested against tumors that may be difficult to distinguish from the one in question (tumors with similar phenotype, e.g., round cell tumors) with routine stains and tumors present in the same location/organ (Ramos-Vara et al., 2007). Validation should also include evaluation of staining differences among different tumors, staining differences within tumors—particularly when different phenotypes are present (e.g., spindle and epithelioid melanomas staining differences with Melan-A)—and differences between primary and metastatic tumors. Validation is critical given the relative immunologic promiscuity (recognition of more than one cell type or tumor) of most antibodies. Finally, and due to the proven variation of antibody reactivity among different species, standardization and validation of an immunochemical procedure must be done in each species examined.
Immunocytochemistry
Processing of Cytologic Samples
Immunocytochemistry can be performed on most types of cytologic samples including cytospins, cell smears, cell blocks, and liquid-based monolayer preparations (Fetsch and Abati, 2004). Cytospins and cell smears are used when the sample volume is small. The advantage of cytospins is better preservation of cytomorphology. However, ICC on cytospins, cell smears, and liquid-based monolayer preparations (ThinPrep) tends to produce more background staining (Barr and Wu, 2006). Cell blocks of FFPE thrombin clots are the method of choice when there are abundant cells (e.g., effusions). The advantage of cell blocks is the similarity of processing to surgical pathology specimens (and therefore comparable results), the possibility of preparing multiple sections of the same block (e.g., for testing multiple markers), and ease of storage. Cell blocks may present some disadvantages such as loss of cytomorphology and loss of antigenicity due to formaldehyde fixation (Brown, 2001). Cell blocks are preferred for nuclear antigens (e.g., Ki-67, p53, PCNA), whereas air-dried cytospins are preferred for the detection of surface antigens (e.g., leukocytic antigens). ThinPreps are less suitable than cell blocks for detection of nuclear antigens (Gong et al., 2003).
ICC may be performed on previously stained Romanowsky or Papanicolau slides when that is the only available specimen and produces similar results to that of unstained slides. The ICC staining can be done with or without previous destaining (with acid alcohol) of the routine stain (Abendroth and Dabbs, 1995; Barr and Wu, 2006; Miller and Kubier, 2002). However, there are some technical drawbacks to using previously stained slides: loss of cells from the slide, cell disruption (affecting mostly ICC of membranous and cytoplasmic markers), and signal reduction for some markers (e.g., S100) due to repeated passage of the sample through graded alcohols. In cases in which only a slide is available and the area containing cells is large, multiple markers can be tested simultaneously. Alternatively, the sample can be divided following tissue-transfer techniques (Sherman et al., 1994).
Fixation
Cytology slides are either wet-fixed or air-dried and fixed immediately before performing ICC (Dabbs, 2002). When wet-fixed preparations were compared with air-dried samples, there were no significant changes in terms of cytologic preservation or ICC staining. However, air-dried preparations may lose fewer cells than wet-fixed samples. Air-dried preparations can be stored at 2 to 8 °C for up to 2 weeks before immunostain without loss of antigenicity (Fetsch and Abati, 2004). Samples are put in a plastic microscope slide box, then in a zip-lock plastic bag containing desiccant. Samples should equilibrate to room temperature before the bag is opened to avoid cell rupture. Storage for several weeks can be done at −70°C (Suthipintawong et al., 1996).
One of the main problems in comparing the quality of immunostaining in cytology preparations is the wide range of fixatives and fixation protocols used in different laboratories. This issue is very different from diagnostic IHC, in which a universal fixative, 10% buffered formaldehyde is used in most instances. Here we are giving general rules, but each laboratory should standardize and validate their protocols to obtain consistent results. Fixation is necessary to preserve cell integrity during multistep immunostaining procedures. Fixation should be performed immediately before immunostaining; theoretically, the type of fixative is selected on an antibodyby-antibody basis. In general, for lymphoid and melanoma markers, samples should be fixed for 5 to 10 minutes at room temperature in acetone; for epithelial markers, 5 minutes at room temperature in alcohol (95% ethanol or a 1:1 mixture of methanol and 100% ethanol); for nuclear antigens, 3.7% buffered formalin for 15 minutes (Fetsch and Abati, 2004). Some authors favor formal saline as a universal fixative suitable for most antigens (Leong et al., 1999). S100 protein, gross cystic disease fluid protein-15, and hormone receptors are not well demonstrated in ethanol-fixed samples due to cytoplasmic antigen leakage (Dabbs, 2002).
Method
Immunocytochemical methods parallel those of immunohistochemistry. There are several steps previous to the incubation of the primary antibody (e.g., endogenous peroxidase block, nonspecific binding block, avidin-biotin block, antigen retrieval). After the primary antibody incubation, a secondary, and sometimes a tertiary, reagent is necessary to demonstrate the immune reaction. The peroxidase block is necessary when using immunoperoxidase techniques; the usual method is with 3% H202 in deionized water. The peroxidase blocking step can be omitted in acetone-fixed specimens. Antigen retrieval is necessary in numerous occasions. Unfortunately, there is no rule to determine a priori whether antigen retrieval is needed or which method is optimal. The approach to standardize ICC is similar to that for IHC. A comprehensive list of antibodies used in ICC at the National Cancer Institute including antibody sources, dilution of the primary antibody, and fixation and antigen retrieval methods is available (Fetsch and Abati, 2004).
Interpretation of ICC and IHC
Immunohistochemistry is an ancillary method and therefore needs to be interpreted in conjunction with clinicopathologic data, including cytologic and surgical biopsy findings, if available. In very few cases is IHC considered a standalone technique in human or veterinary pathology. Specific knowledge of the right staining pattern of a given marker is extremely important to determine whether the staining is significant or not. Interpretation of ICC is more challenging than that of IHC because of the difficulty in obtaining positive and negative control samples treated in a similar way to the test sample and the additional difficulty of distinguishing normal from neoplastic cells. As stressed in the upcoming section on immunohistochemical diagnosis of metastatic tumors, there are very few, if any, antibodies that are truly specific for a single cell type. The interpretation of an IHC reaction is based on the expected “antibody personality profile” (see below) and the infidelity of tumor-specific markers (e.g., reactivity in T-cells with B-cell markers) (Yaziji and Barry, 2006). Simultaneous presence of an antigen in more than one cellular compartment is possible in neoplastic cells, but usually results from diffusion of proteins due to cellular damage during processing. Detection of an antigen in an unusual location should be interpreted with caution and indicated in the immunohistochemical report. Generally speaking, antibodies used in IHC can be used in ICC. In addition, some antibodies that are nonreactive in surgical biopsy specimens are suitable for cytologic preparations.
The interpretation of an immunohistochemical reaction requires a definition of positive or negative staining. This is a controversial issue so only guidelines will be given. Some markers are expected to be present in most cells in a tumor (e.g., cytokeratins in a carcinoma), whereas the detection of other markers (e.g., uroplakin III in transitional cell carcinoma) in only a small group of cells is considered a positive result. Perhaps, as recommended recently in human pathology (Goldstein et al., 2007), a statement in the IHC report indicating the intensity of the staining and the percentage of positive tumor cells would be more informative than merely a positive or negative result as provided in a recent veterinary study (Höinghaus et al., 2008). The lack of expression of a particular antigen may be as significant as its presence in prognostication (e.g., absence of expression of progesterone receptor is linked to poor outcome in human breast cancer; lack of bcl-6 expression combined with MUM1 expression in cutaneous large B-cell lymphoma is linked to short survival) (Bardou et al., 2003; Sundram et al., 2005). In human pathology IHC antibodies are classified as Class I devices by the FDA, meaning that antibodies are considered special stains as adjuncts to conventional histopathologic diagnostic examination (Rhodes, 2005). In other words, with some exceptions, IHC is not a standalone technique and results must be interpreted by the pathologist in the context of the disease. Some IHC tests (e.g., assays for ER, PR, HER2/neu) are considered class II devices with potential predictive or prognostic value. Similarly in veterinary medicine, immunohistochemical results are part of the pathology report and need to be interpreted by the pathologist (Ramos-Vara et al., 2008).
Limitations of Immunohistochemistry
Although IHC has largely displaced electron microscopy as the ancillary technique of choice in diagnostic pathology, it has some limitations (Fisher, 2006). One of the main problems is the lack of standardization and quality control among laboratories, particularly in regard to antigen retrieval. This interlaboratory lack of reproducibility is significant when dealing with prognostic markers (Mengel et al., 2002). Interpretation of immunostaining is also subjective (wide range of interobserver interpretations) and a degree of knowledge of IHC and the antibodies used is required to interpret results correctly. What constitutes a positive result (percentage of positive cells needed, intensity of the reaction) is still controversial, but a critical issue for therapeutic decisions in oncology. Some tumors do not express specific markers beyond the generic ones, which makes testing with multiple markers expensive and unrewarding. Neoplastic cells up- and downregulate gene expression, resulting in the lack of expression of expected antigens or the expression of new antigens. All these issues are perhaps more serious in veterinary medicine, where the degree of sophistication and use of IHC techniques is not as advanced as in human pathology, and are exacerbated by interspecies differences in antigen expression and detection.
Troubleshooting
General Lack of Staining
The most common cause of lack of staining in the test and control samples is improper procedure (from fixation to immunocytochemical procedure including antigen retrieval, antibody concentration, and an improper counterstain) (Dabbs, 2002). A systematic approach to the entire IHC procedure is necessary to determine the cause of staining failure.
Weak Staining
In this context, weak staining applies to both the positive (tissue) control and the test sample and is the result of too much buffer left after a rinsing step, excessive antibody dilution, insufficient incubation time, or improper storage of reagents including buffers, antibodies, and substrates (Fetsch and Abati, 2004). If weak staining only affects the test sample it might be the result of loss of epitopes in the tissue or overfixation.
Background Staining and False-Positive Staining
There are multiple causes of background staining. A common one is inadequate blocking of serum proteins. Blocking is usually done with normal serum or protein (Brown, 2001). Bovine serum albumin has been used extensively in the past as a blocking reagent of nonspecific reactions. However, there is recent evidence that adding albumin to antibody and diluent solutions increases the background of immunohistochemical reactions (Mittelbronn et al., 2006). Other causes of false-positive staining are necrotic tissue, crushed cells, improper fixation, incomplete blocking of endogenous peroxidase or endogenous biotin, and high concentration of the primary antibody (Dabbs, 2002). Samples that are too thick tend to trap reagents and produce background staining. Carcinoma cells in fluids often express vimentin and lose their immunoreactivity for cytokeratins; antigens shed into effusion fluid can be absorbed onto the surfaces of other cells present in the same fluid. Some antigens in cytologic samples such as factor VIII-rAg and immunoglobulins tend to diffuse into the surrounding tissue contributing to incorrect interpretation of immunostaining (Barr and Wu, 2006). To avoid overstaining due to the concentration of the primary antibody, retitration of primary antibodies on cytologic preparations is recommended. Other causes of background staining are included elsewhere (Ramos-Vara, 2005). When using detection kits that recognize primary antibodies made in goats, extensive background in both the positive and negative controls is observed if using tissue sections from the same or related species (ruminants) due to the presence of endogenous immunoglobulins recognized by the secondary antibody (anti–goat IgG). A similar problem is observed with rabbit monoclonal antibodies tested on rabbit tissues or mouse monoclonal antibodies tested on mouse tissues. Special detection procedures are commercially available to avoid this background staining. Although not an example of nonspecific background staining, it is very important in immunocytochemistry to distinguish positive staining in normal or reactive cells from that of neoplastic cells. This distinction can be challenging when the number of reactive cells is higher than that of the neoplastic cells (e.g., T-cell–rich B-cell lymphoma). Fig. 17-1 and Fig. 17-2 show examples of background and nonspecific staining.
The Use of Controls
The same type of controls described under IHC is used for ICC (tissue and reagent controls). Ideally, the control tissue sample should contain the antigen of interest and be fixed and processed in an identical way to the test sample. The ideal positive control should demonstrate immunoreactivity that is weak in some places and strong in others. A negative reagent control is also necessary for each antibody tested (see Fig. 17-2). For the negative reagent control, either an irrelevant antibody or nonimmune serum from the same species as the primary antibody (and ideally the same Ig isotype for monoclonal antibodies) replaces the primary antibody (Fetsch and Abati, 2004). The slide with the negative reagent control should be processed in an identical manner as the slide with the primary antibody. The negative control slide is used to assess nonspecific staining that is not the result of specific antigen-antibody binding (background staining). If only one slide is available, it may be used divided into test and negative reagent control by circling the areas of interest with a diamond or wax pen or using the cell transfer technique already mentioned (DeLellis and Hoda, 2006). In some instances, a slide negative for one marker can be used to test a second marker.
Panel Markers for Diagnostic Immunohistochemistry of Tumors
The goal of diagnostic IHC is to maximize sensitivity without compromising specificity of results. A typical approach is to cover the main tumor types with antibody panels that include cytokeratins (carcinoma), vimentin (sarcoma), S-100 (melanomas or peripheral nerve sheath tumors), and CD45 (leukocytic neoplasms). To achieve maximum sensitivity, the use of “redundant” antibodies for a given antigen is recommended: in other words, the use of several antibodies that should label the same cell type. Table 17-2 lists cell markers and their use in the immunochemical diagnosis of tumors, with emphasis on organ system. In human pathology, the following expanded panel has been proposed: Pan-cytokeratin (carcinomas), CD45 and CD43 (lymphomas), S-100 and Melan-A or gp 100 (melanomas), vimentin and collagen IV (sarcoma) (Yaziji and Barry, 2006). Some of these markers are not available or not reactive in animal tissues, so alternatives need to be found. Once other clinicopathologic data has been examined judicious use of antibodies is the best approach: it will reduce both the cost of testing and the need to explain unexpected reactions to the client. Once a particular tumor group has been identified (e.g., sarcoma), more specific markers to determine the type of tumor are used. This approach is based on algorithms. Fig. 17-3 shows a basic algorithm to characterize tumors frequently found in domestic species. This algorithmic approach is borrowed from the human experience; unfortunately many markers currently used in human pathology are not reactive in animal tissues or their reactivity is different (in other words, when dealing with IHC, not all animal species and antibodies are created equal). This problem is compounded because the extensive testing (validation) of antibodies used in human diagnostic IHC is almost nonexistent in veterinary medicine. The lack of predictive behavior (percentage of positive cases of a tumor with a particular antibody) is one of the most difficult barriers to overcome in veterinary diagnostic IHC. The use of a particular marker will be also determined by its availability in the laboratory. Many antibodies with diagnostic or prognostic significance in human pathology await validation in similar tumors of animals (Capurro et al., 2003). A similar algorithmic approach can be used in immunocytochemistry.
TABLE 17-2 Markers Used for the Differential Diagnosis of Major Tumor Categories
Tumor Tissue | Markers |
---|---|
Adrenal | |
Endocrine tumors (generic) | Chromogranin A, synaptophysin, PGP 9.5, neuron specific enolase (NSE), S100 |
Epithelial vs. mesenchymal | Cytokeratins (epithelial), vimentin (mesenchymal), E-cadherin (epithelium), p63 (basal cells, myoepithelium) |
Leukocytic | CD45 (panleukocytic), CD18 (with emphasis in histiocytic), CD11d (dendritic cells), E-cadherin (Langerhan’s cells), lysozyme (histiocytes), myeloid histiocytic marker (histiocytes, myeloid cells) |
Liver | Hep Par 1 (hepatocytes), cytokeratin 7 (bile duct epithelium) |
Lymphoid | CD3 (T-cell), CD79a and CD20 (B-cell), CD45 and CD18 (panleukocytic), MUM1 (plasma cells) |
Mast cell tumors | CD117, tryptase, OCT3/4 |
Melanocytic tumors | Melan A, S100, NSE |
Muscle differentiation | Actin muscle (all muscle), smooth muscle actin (smooth muscle), myoglobin (skeletal muscle), actin sarcomeric (striated muscle), desmin (all muscle), calponin (smooth muscle, myofibroblast, myoepithelium) |
Neurogenic tumors | S100 (neurons, glial cells), neurofilament (neurons), GFAP (glial cells), glut1, nerve growth factor receptor (perineural cells) |
Pancreas (endocrine) | Insulin, glucagon, somatostatin, synaptophysin, PGP 9.5, chromogranin A |
Squamous vs. adenocarcinoma | Squamous cell carcinoma (CK5, p63); adenocarcinoma (CK7) |
Testis and ovary | Sex cord–stromal tumors (inhibin-α, NSE); germ-cell tumors (calretinin, KIT, PGP 9.5) |
Thyroid | Thyroglobulin (follicular cells), calcitonin (medulla, C-cells), TTF1 (follicles and medulla) |
Urinary tumors | Uroplakin III, cytokeratin 7, COX-2, COX-1 |
Vascular tumors (endothelium) | Factor VIII–related antigen, CD31 |
Antibody personality profile (APF) is a new concept introduced by Yaziji and Barry (2006). An APF is defined by 1) location of expected signal (e.g., cytokeratins are exclusively cytoplasmic; S-100 protein and calretinin are cytoplasmic and nuclear; CD45, CD11 are in the cell membrane; laminin, collagen IV are found only in the interstitium); 2) antibody pattern (S-100 produces a homogeneous signal; cytokeratins, a filamentous signal; chromogranin A and Melan-A, a granular signal); 3) antibody-characteristic pattern across tissues and tumors (thyroid transcription factor-1 [TTF-1] stains most neoplastic cells in a pulmonary carcinoma; uroplakin III stains only a small percentage of tumor cells). Knowledge of the profile facilitates accurate interpretation of immunohistochemical results. Keep in mind that the APF may vary among animal species (Ramos-Vara et al., 2000, 2002b).
Immunochemical Diagnosis of Anaplastic or Metastatic Tumors
The number of antibodies available for diagnostic purposes has increased exponentially in the last few years. This gives the diagnostician more opportunities to make a definitive diagnosis. Keep in mind that regardless of the number of markers used to characterize a particular tumor, the gold standard before attempting IHC should be HE. A careful examination of HE-stained slides will reduce the number of markers needed to arrive to a definitive diagnosis. Even after that, it is uncommon to make a definitive diagnosis with only one marker because expression (or lack of thereof) of proteins in tumor cells may differ from that in the normal cell counterpart. Upregulation and downregulation of gene expression and the proteins codified by such genes is common in neoplastic cells. The use of tumor marker panels in the diagnosis of metastatic disease is key to improving our chances of arriving at a definitive diagnosis. Considering the relatively low cost of IHC and the expenses of treating some tumors, clinicians are keen to get a definitive answer from the pathologist. A treatment tailored to a specific tumor will more likely improve the quality of life of the animal.
The proposed series of steps to characterize a metastatic tumor has been modified from Dabbs (2006) and include: 1) Determine the cell line of differentiation using major lineage markers. 2) Determine the cytokeratin type for carcinomas and possible coexpression of vimentin. 3) Determine if there is expression of cellspecific products, cell-specific structures, or transcription factors unique to specific cell types. The main difference with the algorithmic approach for a metastatic tumor is that without knowing the location of the primary tumor, the differential diagnosis includes more tumor types and the tumor marker panel therefore includes more antibodies.
Determine the Cell Line of Differentiation
Markers should include keratins as well as lymphoid, melanoma, and sarcoma markers (Chijiwa et al., 2004; Höinghaus et al., 2008). A basic panel of markers for small animals is: pancytokeratins (clones AE1/AE3 or MNF 116), CD45 (panleukocytic marker), Melan-A or S100 (melanocytic differentiation), and vimentin (mesenchymal differentiation).
Determine the Cytokeratin Type for Carcinomas and Coexpression of Vimentin
Cytokeratins comprise approximately 20 polypeptides with different molecular weight, numbered 1 through 20. They are separated by charge into acidic (type I) and basic (type II) keratins. Cytokeratins are paired together as acidic and basic types. Most low–molecular weight keratins (e.g., CK 7, 8, 18, 20) are present in all epithelia except squamous epithelium, whereas high–molecular weight keratins (e.g., CK 1, 2, 3, 4, 9, 10) are typically present in squamous epithelium. Almost all mesotheliomas and carcinomas, except squamous cell carcinomas, have CK 8 and 18. The coordinate expression of CK 7 and CK 20 is one criterion to classify carcinomas in human pathology. This approach has proven very useful in metastatic carcinomas of undetermined origin. There is only one paper published regarding domestic animals that examines a wide range of carcinomas in dogs and cats for expression of CK 7 and CK 20 (Espinosa de los Monteros et al., 1999). Results for CK 7 were similar to those in humans, but major differences were observed for CK 20 among both animal species. CK 5 is a useful marker of myoepithelial differentiation in glandular tumors as well as for squamous epithelium and mesothelial cells. Although cytokeratins are the typical marker of epithelial differentiation, they can be detected in mesenchymal tumors (melanoma, leiomyosarcoma, gastrointestinal stromal tumors, liposarcoma, meningioma, and angiosarcoma), although usually in only a few cells, as opposed to the diffuse and strong staining of carcinomas and sarcomatoid carcinomas (Dabbs, 2006). Coexpression of intermediate filaments has been reported in certain human fetal and adult tissues.
Expression of Cell-Specific Products
Neuroendocrine Markers.
Within the generic neuroendocrine markers, synaptophysin and chromogranin A are the most commonly used and specific for this group of tumors. Antibodies for these markers work well in most animal species. Keep in mind that synaptophysin, in addition to staining the majority of pheochromocytomas of the adrenal gland, may stain a significant number of adrenal cortical tumors as well. Neuron specific enolase (NSE) is another classic generic neuroendocrine marker. Unfortunately, this marker is less specific than its name claims and stains other nonendocrine cell types, making its use in diagnostic IHC questionable. A recently used neuroendocrine marker in veterinary pathology, protein gene product (PGP) 9.5, a ubiquitin hydrolase, labels many neuroendocrine cells but also labels unrelated tumors (RamosVara and Miller, 2007). Antibodies to peptide hormones (e.g., thyroglobulin, calcitonin, glucagon, insulin) usually cross-react among different animal species and demonstrate specific endocrine cell types.
Specific Markers.
Every year, numerous scientific papers report the characterization of “novel” markers (antibodies) that are extremely specific for particular human cells or tumors. Most eventually will be relegated to use in combination with other antibodies (as part of a tumor panel). In this section are presented some markers that are useful in the characterization of specific animal tumors. Thyroid transcription factor-1 (TTF1), a nuclear transcription factor, is frequently expressed in thyroid tumors (more common in follicular, but also present in medullary, tumors) and pulmonary tumors (Ramos-Vara et al., 2002a, 2005). Other tumors, including mesotheliomas, are usually negative. Hep Par 1 (hepatocyte paraffin 1) is consistently detected in hepatocytes and their tumors with no staining of biliary epithelium, which makes it a good choice to distinguish these tumors, particularly when used in conjunction with CK 7 (Ramos-Vara et al., 2001a). However, some intestinal, and probably pancreatic, tumors can be positive (Ramos-Vara and Miller, 2002). Melan-A is one of the best specific and sensitive markers of melanomas in dogs (less sensitive in feline melanomas) and certainly more specific than other classic markers such as S100 and NSE (Ramos-Vara et al., 2000, 2002b). It should be noted that many steroidproducing tumors from the adrenal cortex, testis, and ovary show strong reactivity for Melan-A (Ramos-Vara et al., 2001b). Uroplakin III, a major component of the asymmetric unit of transitional epithelium, is expressed in most canine transitional cell carcinomas and, in conjunction with CK 7, the number of transitional cell carcinomas detected approaches 100% (Ramos-Vara et al., 2003). Uroplakin III has not been detected in nonurothelial normal or neoplastic tissues of dogs which makes this marker the exception to the rule (coexpression of the same marker in different cell types).
A marker widely used in human pathology to discriminate mesothelioma from carcinoma is calretinin. However, attempts to use it in canine mesotheliomas with a variety of antibodies have been unrewarding. There is a report of calretinin staining in equine mesothelioma (Stoica et al., 2004). The differential diagnosis of mesothelioma and pulmonary carcinoma is challenging in human pathology and numerous antibodies have been tested. A recent study indicates that the combination of D2-40 and calretinin (both positive in mesothelioma and negative in lung carcinoma) and CEA and TTF-1 (both negative in mesothelioma and positive in pulmonary carcinoma) antibodies is an economic way to distinguish these two types of tumors (Mimura et al., 2007). Desmin is detected in reactive mesothelial cells but not in mesothelioma or carcinoma in cytologic preparations (Afify et al., 2002). As previously mentioned, TTF-1 is a specific and sensitive marker of canine pulmonary and thyroid carcinomas. The use of CEA on animal tumors is very limited. We are not aware of D2-40 staining of mesotheliomas in animal species. The use of both cytokeratins and vimentin (usually coexpressed in mesotheliomas) is probably the best approach to distinguish mesothelioma from pulmonary carcinoma in animals (Geninet et al., 2003; Morini et al., 2006; Sato et al., 2005; Vural et al., 2007).
A smooth muscle–specific protein, calponin, has been evaluated in canine mammary tumors (Espinosa de los Monteros et al., 2002). In addition, Webster et al. (2007b) studied the expression of the embryonic transcription factor OCT4 in canine neoplasms.
Antibodies as Prognostic Markers in Veterinary Oncology
Proliferation/Cell Cycle Markers
This group includes Ki67, PCNA and cyclins, and in general indicates the proportion of proliferating/cycling cells in a given tumor; these markers correlate well with mitotic index. Malignant tumors generally have more proliferating cells than benign tumors, with some exceptions. Lymphomas, mammary tumors, melanocytic tumors, and mast cell tumors are probably the tumors in domestic species in which these markers have been studied most extensively (Ishikawa et al., 2006; Kiupel et al., 1999; Madewell, 2001; Sakai et al., 2002). In mast cell tumors, there is good correlation between decreased survival time and Ki67 index and between the histochemical detection of nuclear organizing regions (AgNORs), which determine the rate of cellular proliferation (generation time) and decreased disease-free interval (Webster et al., 2007a). In a different study (Scase et al., 2006), both AgNORs and Ki67 scores were considered useful prognostic markers for canine mast cell tumors, with Ki67 score used to divide Patnaik grade 2 mast cell tumor into 2 groups showing markedly different actual survival times. PCNA score did not correlate with differences in survival times of several types of tumors (Roels et al., 1999; Scase et al., 2006; Webster et al., 2007a). The prognostic significance of detection of cyclins in animal tumors has not been fully evaluated (Murakami et al., 2001).
Telomerase
Telomeres are portions of repetitive DNA that protect chromosomes from degradation and loss of essential genes (Cadile et al., 2007). With each cell division, telomeres progressively shorten in all somatic cells until cells undergo replicative senescence or apoptosis. Telomerase is a ribonucleoprotein enzyme complex that synthesizes telomere DNA. In normal cells telomerase is detected in male germ cells, activated lymphocytes, lens tissue, and stem cell populations but not in somatic cells. In human cancer, telomerase activity is detected in 85% to 90% of cases and in dogs more than 90% of tumors examined express telomerase activity (Kow et al., 2006). Telomerase expression in dogs is significantly associated with tumor proliferation (Ki67 labeling index) and/or tumor grade (Long et al., 2006). Immunohistochemical detection of telomerase could be useful as a prognostic marker and tool to determine the therapeutic approach to cancer (Argyle and Nasir, 2003).
KIT
The KIT protein, a tyrosine kinase receptor product of the c-kit proto-oncogene, is expressed in numerous tissues and cells including mast cells and mast cell tumors. Immunohistochemical staining patterns of KIT in canine mast cell tumors have been used as a prognostic tool (Kiupel et al., 2004). In a normal mast cell, KIT is localized in the cell membrane; localization within the cytoplasm in mast cell tumors has been linked to increased rate of local recurrence, decreased survival rate, or increased tumor grade (Reguera et al., 2000).
Epithelial-Mesenchymal Transition (EMT)
Observed in some human cancers is the loss or redistribution of epithelial markers and gain of mesenchymal markers. EMT is usually associated with one or more of the following tumor features: increased tumor cell motility, invasive potential, tumor grade or tumor stage (Baumgart et al., 2007). The most common proteins affected in EMT are epithelial markers (E-cadherin, beta-catenin, and plakoglobin) and mesenchymal markers (N-cadherin and vimentin). Although it is likely to occur, there is no published evidence of EMT or its prognostic significance in cancer of domestic animals.
ELECTRON MICROSCOPY
The ultrastructural examination of tissues and cells is one of the most common ancillary methods used in diagnostic cytology and pathology (Dardick et al., 1996). If the markers of immunohistochemistry are structural or secretory proteins specific for a cell or tissue, then the markers of electron microscopy (EM) are subcellular structures such as organelles or matrix constituents. Electron microscopy has contributed in great measure to an understanding of the structural features of normal and pathologic tissues. Although the use of EM has declined in the last decade and been partially replaced by other techniques (e.g., immunohistochemistry), EM is still a very valuable tool to reach a definitive diagnosis in some difficult cases, particularly in peripheral nerve sheath tumors, some synovial sarcomas, pleomorphic sarcomas, and mesotheliomas (Dardick and Herrera, 1998; Mackay, 2007). EM and IHC should be used in a complementary fashion based on the type of diagnostic problem (Fisher, 2006). As an ancillary technique, EM raises the level of confidence in diagnoses based on light microscopy. Of the three main types of ancillary techniques currently used in veterinary pathology (EM, IHC, PCR), EM is the most mature technique, meaning that it has gone through the usual stages of development, evaluation and, stabilization as opposed to the other two techniques that are still in development or evaluation stages. As the saying goes, embrace the new techniques if they are worthy and keep the proven old ones.