Lindsay S. Rogers and Meryl S. Cohen The Children’s Hospital of Philadelphia, Philadelphia, PA, USA Cardiac malposition is defined as any position of the heart other than normal with normal being the heart positioned in the left chest with apex pointing leftward (also known as situs solitus with levocardia) [1]. Situs, a Latin term for position, is used to describe the position of lateralized structures (viscera and heart). The term malposition is also used to describe abnormal spatial arrangements of the individual cardiac segments – atria, ventricles, and the great arteries – and thus encompasses a wider range of anomalies. This section covers malposition of the entire cardiac mass (dextrocardia and mesocardia) and isolated ventricular inversion [2,3]. Heterotaxy syndrome [4] is characterized by the presence of cardiac malposition, abnormal spatial organization of the thoraco‐abdominal organs, and often anomalies of multiple cardiac segments. Cardiac malposition in heterotaxy syndrome is common. Cardiac malposition due to ectopia cordis is not addressed in this chapter. Dextrocardia and mesocardia are types of cardiac malposition [5]. In levocardia the base‐to‐apex axis points to the left, whereas it points to the right in dextrocardia, and more caudally in mesocardia [6]. Situs inversus refers to the mirror‐image arrangement of the normal thoracoabdominal visceral situs (see Chapter 3). Situs inversus is generally associated with dextrocardia, but may be rarely seen with levocardia. The term dextroposition is used to describe cardiac displacement into the right hemithorax by extracardiac factors such as hypoplasia or agenesis of the right lung, pulmonary mass, or left diaphragmatic hernia. Another term used in the literature is dextroversion, or pivotal dextrocardia. It usually describes dextrocardia with situs solitus, where the cardiac apex appears as having been rotated from the left hemithorax to the right. Given the variety of terms used to describe malposition of the heart, stating the position of the heart in the chest (dextrocardia, levocardia, or mesocardia) followed by the apex direction allows for clarity and avoids miscommunication. For instance, the normal scenario is levocardia with a leftward pointing apex. Isolated ventricular inversion is a term first used in 1966 [2] to describe a rare situation where ventricular inversion is not associated with transposition of the great arteries (TGA) as in the usual case of “congenitally corrected” TGA. Segmental anatomy using the Van Praagh method allows for accurate diagnosis of this abnormality. The case originally presented displayed thoracoabdominal situs solitus, L‐loop ventricles, atrioventricular discordance, and ventriculoarterial concordance, segments {S,L,S}. The definition of isolated ventricular inversion also applies to atrial situs inversus with D‐loop ventricles and inverted normally‐related great arteries (ventriculoarterial concordance with anterior and rightward pulmonary artery; segmental set: {I, D, I}) [7]. This specific entity is also referred to as isolated ventricular noninversion. The incidence of fetal dextrocardia is reported as 220 to 830 per 100,000 fetuses referred for echocardiography [8–10]. Postnatally, dextrocardia is reported in 2.8% of cyanotic congenital heart disease (CHD) and 0.4% of acyanotic heart disease [11]. The most common type of dextrocardia in the general population is associated with situs inversus totalis. The prevalence of situs inversus is approximately 1 in 10,000 for situs inversus totalis [12] and 1 in 22,000 for situs inversus with levocardia [11]. Dextrocardia with situs inversus (heart and stomach on the same side) is usually associated with normal intracardiac anatomy [9]. In contrast, dextrocardia with situs solitus, or levocardia with situs inversus (heart and stomach on opposite sides) is associated with a high rate of cardiovascular malformations. Isolated ventricular inversion is a very rare anomaly and only case reports or small case series exist in the literature. Although the genetic basis of isolated cardiac malposition is not well described in the literature, recent studies suggest that it likely has shared origins with situs inversus and heterotaxy syndrome [13]. It has been long understood that mutations in genes defining laterality and left–right asymmetry influence cardiac position and ventricular looping, causing laterality defects associated with CHD [14]. Recently, abnormal ciliary development, long known to be associated with laterality defects, has been implicated in the formation of isolated CHD (without malposition), highlighting the important influence of ciliary motion in the developing embryo. It is important to note that the developmental mechanisms of most congenital heart defects are speculative and are inferred from knowledge about normal human cardiac development and abnormal development in animal models. Dextrocardia in D‐loop ventricles is probably related to arrested migration of the cardiac apex to the left hemithorax, a process that normally occurs between 23 and 35 days of gestation. L‐loop ventricles, however, have a tendency to migrate to the right hemithorax, which explains the high incidence of dextrocardia in this type of looping. The embryogenesis of isolated ventricular inversion [2] is believed to be due to twisting of the ventricles and the great arteries in different directions during early cardiovascular development. Isolated ventricular inversion typically occurs with normal conotruncal development with a ventricular L‐loop. A broad spectrum of cardiac anomalies can be associated with dextrocardia, ranging from an isolated ventricular septal defect (VSD) to the most complex forms of congenital heart disease. In the presence of situs inversus with dextrocardia (segmental set {I,L,I}), the most commonly associated congenital heart defects are tetralogy of Fallot and VSD [15], but rarer conditions such as single coronary ostium and hypoplastic left heart syndrome have been reported [16,17]. The aortic arch is usually right‐sided with mirror‐image branching [18]. In isolated ventricular inversion (atrioventricular discordance with ventriculoarterial concordance connections) the ventriculoarterial connections are normal. Therefore, with levocardia the segments are {S,L,S} and with dextrocardia, called ventricular (non)inversion, the segments are {I,D,I}. However, the spatial positioning of the great arteries relative to the ventricular chambers is not normal with a more inferior position of the great arteries [19,20]. Associated intracardiac defects include a VSD, hypoplastic tricuspid valve with right ventricular hypoplasia, and coarctation of the aorta [20]. The presentation of dextrocardia depends on the associated cardiac anomalies and can vary from an incidental finding on a chest X‐ray or echocardiography to symptoms of the underlying congenital heart defects. Isolated ventricular inversion is associated with transposition physiology causing cyanosis in the neonatal period. When the heart is in the right chest, a distinction should be made between a rightward apex (true or mirror‐image dextrocardia) and a leftward orientation of the apex with rightward position (Figure 30.1). It is crucial when imaging dextrocardia to maintain the probe in standard orientations (see Chapter 4). In the subxiphoid frontal (long‐axis) view the apex is oriented rightward (Figure 30.2a, Video 30.1). With clockwise rotation of the transducer the normal subxiphoid (sagittal) short‐axis views are obtained, but the standard sweeps should be performed from left to right (base to apex) instead of right to left as done in levocardia. In the apical four‐chamber view the probe is placed on the right chest with the notch pointing to the left (Figure 30.2b). For the parasternal long‐axis view the probe is placed on the right chest with the notch pointing to the left shoulder. This results in the normal display of the cardiac apex pointing to the left of the screen. To obtain the right parasternal short‐axis view, the probe is rotated clockwise from the parasternal long axis, with the notch now pointing to the left hip (Figure 30.2c). A common pitfall in the right parasternal short‐axis view is the erroneous rotation of the probe counterclockwise toward the patient’s right shoulder. Left parasternal long‐ and short‐axis views can also be utilized and have been found helpful in delineating great artery relationships [6]. Suprasternal notch views should be obtained in the traditional manner, with frontal view showing the pulmonary artery to the right of the aorta and the left pulmonary artery crossing under the ascending aorta (Figure 30.2d). The segmental approach should be used to describe the entire cardiac anatomy and associated lesions. Figure 30.1 Dextrocardia (a) versus dextrocardia with levoversion (b). Figure 30.2 Situs inversus totalis. (a) Subxiphoid long‐axis image of situs inversus totalis {I,L,I} with a membranous ventricular septal defect (arrowhead). (b) Apical four‐chamber view showing situs inversus of the atrium and L‐looping of the ventricles. (c) Right parasternal short‐axis image of situs inversus totalis with a membranous ventricular septal defect (not shown). (d) Suprasternal frontal view showing mirror‐image systemic venous drainage and relationship of ascending aorta crossing over the left pulmonary artery. Ao, aorta; IV, innominate vein; LA, left atrium; LSVC, left superior vena cava; LV, left ventricle; RA, right atrium; RPA, right pulmonary artery; RV, right ventricle; RVOT, right ventricular outflow tract; TV, tricuspid valve. For these rare and often complex lesions, a full segmental approach using all standard imaging views should be used. Pasquini et al. [19] highlighted certain imaging findings specific to isolated ventricular inversion: Figure 30.3 Dextrocardia with ventricular (non) inversion {I,D,I}. (a) Subxiphoid frontal (long‐axis) view showing aorta (Ao) arising from left ventricle (LV; on left) with pulmonary artery (PA) from right ventricle (RV; on right). Pulmonary valve anterior and rightward to aortic valve. (b) Subxiphoid frontal (long‐axis) view with color showing the ascending aorta crossing in front of the left pulmonary artery (LPA), making the great artery relationship situs inversus (I). There is also a moderate central perimembranous ventricular septal defect, partially profiled in this image. (c) Subxiphoid frontal (long‐axis) view focused on the atria. The morphologic left atrium (LA) is on the right with pulmonary veins (LPV and RPV) entering from both lungs. The foramen ovale is not seen in this view. The left superior vena cava (LSVC) enters the morphologic right atrium (RA) on the left side. (d) Subxiphoid sagittal view showing the anterior left ventricle with two mitral valve papillary muscles (PM) and posterior right ventricle, with only a small portion of the right ventricle wrapping anteriorly to give rise to the pulmonary artery. TV, tricuspid valve. Identification of abnormal position of the heart in the thorax is generally obtained during the routine obstetric ultrasound exam, and often is the main reason for referral for detailed fetal echocardiography. Differentiation between dextrocardia and dextroposition can be determined on the fetal echocardiogram. Intrathoracic abnormalities, including congenital diaphragmatic hernia, cystic adenomatoid malformation of the lung, bronchogenic cysts, and pericardial and pulmonary tumors, are the main causes for dextroposition. Dextroposition without an intrathoracic space‐occupying lesion can be present most commonly in the context of right lung hypoplasia and hypoplasia of the right pulmonary artery, and the most common cardiac defect encountered in this setting is scimitar syndrome [22]. Agenesis of the right lung, which is associated with absence of the mainstem bronchus and right pulmonary artery and veins, is another rare cause of dextroposition. Before surgical planning, a full segmental evaluation is required with a complete description of the anatomy and physiology. Postoperative assessment is obviously determined by the type of surgical repair or palliation. Isolated ventricular inversion can be managed surgically with an atrial switch procedure to correct the transposition physiology and keep the LV connected to the aorta. Associated intracardiac defects like VSDs can be repaired during the same procedure. In cases of ventricular inversion, the conduction system is expected to course on the right‐sided left ventricular side of the septum so the patch needs to be placed on the left side [23]. In contrast to the remarkable external symmetry of all vertebrates, the internal organs are asymmetrically positioned along the left–right axis of the body [24]. This asymmetry refers to lateralization of unpaired organs (e.g., heart, liver, spleen, and stomach) as well as morphologic differences of paired organs (lungs, bronchi, and atrial appendages) that do not allow them to be mirror images of each other. The term heterotaxy (Greek for “other arrangement”) refers to the failure to generate normal left–right asymmetry. Heterotaxy syndrome is defined as the presence of a pattern of spatial organization of the thoracic and abdominal viscera that is neither situs solitus nor situs inversus [4]. Alternatively, heterotaxy can be thought of as the existence of characteristics of both situs solitus and situs inversus in the same individual; some call this situs ambiguous (see Chapter 3). According to the definitions provided by the Working Group of the International Society for Nomenclature in Pediatric and Congenital Heart Disease [4], patients with heterotaxy syndrome can be categorized into the subsets of isomerism of the right atrial appendages/asplenia syndrome or isomerism of the left atrial appendages/polysplenia syndrome. Although right and left atrial isomerism are commonly used terms, it is important to note that atrial isomerism does not exist. It is only the atrial appendages that can be isomeric (symmetric mirror images of each other). Furthermore, appendage and spleen anatomy can be inconsistent within the various subtypes of heterotaxy syndrome. As such, these names help us group commonly seen abnormalities in the two main subtypes, but do not necessary reflect an individual patient’s atrial appendage and/or spleen anatomy. The terms right isomerism and left isomerism are used in this chapter as synonyms of asplenia syndrome and polysplenia syndrome, respectively. Traditionally, it has been described that patients with right isomerism frequently have bilaterally trilobed lungs, each with a short bronchus, eparterial bronchi (the pulmonary artery courses in front of the bronchus), and absence of the spleen. Those having left isomerism frequently have bilaterally bilobed lungs, each with a long bronchus, bilateral hyparterial bronchi (the pulmonary artery courses over the bronchus), and multiple spleens [25]. However, autopsy studies have shown that many patients with heterotaxy syndrome do not follow these anatomic rules. In fact, in a review of autopsy cases (n = 76) at our institution, only about 50% of left isomerism cases had bilateral left atrial appendages and bilateral hyparterial bronchi. The correlation was higher with right isomerism, with at least 88% having bilateral right atrial appendages and about 80% having bilateral eparterial bronchi (with about 20% unknown relationship). These findings support the understanding that many patients with heterotaxy syndrome have a mix of laterality defects and that right atrial isomerism tends to be a more “pure” form of heterotaxy with more consistency in appendage anatomy, bronchoarterial relationships, and more uniform types of cardiac disease. The distinction between the subtypes of heterotaxy syndrome is based on the common concurrence of cardiac anomalies, although there is a wide variation and significant overlap may be found in any given patient. In general, the cardiac abnormalities in right isomerism are more complex and the prognosis is worse. Whatever classification system is used, the echocardiographer should provide a comprehensive description of the thoracoabdominal visceral situs and of all cardiovascular abnormalities through a detailed and systematic segmental analytic approach. Classification of heterotaxy syndrome has traditionally been approached using mixed terminology, making most series difficult to interpret. With that understanding, the majority of the published studies estimate the incidence at 10–14 per 100,000 live births [26–29]. The relative frequency of heterotaxy syndrome is reported as 2.3–4.2% of CHD [26,28]. Heterotaxy results from disruption in the normal left–right symmetry of the lateralized organs, controlled by different signaling pathways and having complex genetic underpinnings. Aneuploidies, complex chromosomal rearrangements, and microdeletions have all been implicated in heterotaxy syndrome. Autosomal dominant, autosomal recessive, and X‐linked inheritance patterns have been described [13]. Pathogenic mutations in ZIC3, a transcription factor required for normal left–right signaling and heart looping in the embryo, have been implicated, especially in those with X‐linked inheritance pedigrees. In addition, environmental factors such as maternal diabetes [29], in utero retinoic acid exposure [30], and monozygotic twinning have been associated with the occurrence of laterality defects. A link between ciliary motility and the regulation of left–right asymmetry was proposed due to the association of primary ciliary dyskinesia with situs inversus totalis [31]. The normal left–right asymmetry of the heart results from left–right signaling cues generated by ciliated embryonic structures (e.g., the left–right organizer, also known as the embryonic node). These cilia generate directional flow that breaks the bilateral symmetry of the early embryo by asymmetric gene expression (genes including cerl2, nodal, lefty, and pix2) [13,32]. Aberrations in left–right organizer cilia play an essential role in laterality defects. More than 30 genes have been identified to be involved in ciliary dyskinesia and associated with various forms of heterotaxy syndrome. Despite increased understanding of the genetic basis of heterotaxy syndrome, the direct genotype–phenotype relationship is less well understood. Recently there has been increasing appreciation that genetic defects in left–right signaling, while known to cause heterotaxy, can also cause isolated CHD [33]. A prime example is loss‐of‐function variants of ZIC3, known to be associated with traditional forms of heterotaxy, which have now been identified in those with isolated CHD. The major events in normal cardiac development that appear to be arrested or absent in heterotaxy syndrome occur largely during the fifth week of gestation [34]. The hearts of many patients with heterotaxy syndrome bear a remarkable resemblance to the heart of a normal embryo at 35 days of gestation. More specifically, the growth of the endocardial cushions, septation of the conotruncus, lobation of the lungs, and rotation of the gut begin between days 28 and 35 of gestation whereas the spleen is present at about 32 days and is well demarcated by 35–36 days [34]. Heterotaxy syndrome is characterized by a wide spectrum of pathology, and its clinical diagnosis is not generally based on strict anatomic criteria. According to Banka et al. [35] the fulfillment of at least three of the criteria outlined in Table 30.1 correctly identifies patients with heterotaxy syndrome. The atrial appendages have traditionally received special attention as a diagnostic criterion because they have been considered by some [36,37] to be the unifying link in the definition and classification of patients with heterotaxy syndrome. Whether isomerism accurately applies to the heart or not, both appendages can share characteristics of a right atrial appendage in right isomerism and of a left atrial appendage in left isomerism. It has been suggested that the extent of the pectinate muscles can reliably distinguish between left and right atrial appendages. In hearts with isomeric appendages, there is either bilateral extension of the pectinate muscles beyond the appendage, onto the wall of the atrium in cases of right isomerism, or confinement of pectinate muscles to the appendage itself in cases of left isomerism [38]. However, appendage anatomy, bronchopulmonary pattern, and abdominal organ arrangement are not always consistent within the same patient with known heterotaxy syndrome [39]. This, along with increasing understanding of the genetic underpinnings of laterality defects and their crossover with isolated forms of CHD, has resulted in the proposal to redefine how we categorize heterotaxy with a push to move away from the traditional classification based on isomerism and/or spleen anatomy [40]. These proposals merit important consideration and will likely lead to a shift in classification as the field advances. However, for ease of communication, consistent with historical literature surrounding this topic, we will use isomerism/spleen anatomy as a way to categorize commonly seen associations with heterotaxy syndrome. Table 30.1 Diagnostic criteria for heterotaxy syndrome. Source: Modified from Banka P, Adar A, Schaetzle B, Sleeper JA, Emani S, Geva T. Changes in prognosis of heterotaxy syndrome over time. Pediatrics 2020;146:e20193345. The usual combination of findings in right isomerism is outlined in Table 30.2 and includes: totally anomalous pulmonary venous connection, usually to a systemic vein; bilateral superior venae cavae; unroofed/absent coronary sinus; common atrium; complete common atrioventricular canal (CAVC); functionally single ventricle, usually of dominant RV morphology; pulmonary stenosis or atresia; double‐outlet right ventricle (DORV), or TGA (Figure 30.4, Video 30.3). Table 30.2 Typical cardiac findings in right isomerism/asplenia and left isomerism/polysplenia DORV, double‐outlet right ventricle; TGA, transposition of great arteries. Figure 30.4 Typical findings for heterotaxy syndrome with right isomerism. Subxiphoid frontal (long‐axis) view. (a) Double‐outlet right ventricle (RV) with aorta (Ao) and pulmonary artery (PA) both arising from the RV. Note the smaller pulmonary valve, indicative of pulmonary stenosis. (b) Bilateral superior vena cavae seen entering their respective atria with the left superior vena cava (LSVC) having a completely unroofed coronary sinus (directly to left atrium). Behind the vena cavae you can see symmetry of the pulmonary arteries (bilateral right pulmonary arteries). There is a common atrioventricular valve (CAVV), secundum and large ostium primum atrial septal defect (only a small amount of atrial septal tissue is present). (c) Posterior, pulmonary vein (PV) confluence seen behind the RA. (d) Color shows bilateral venae cavae to atria and vertical vein (VV) draining the pulmonary vein confluence superiorly to the innominate vein: supracardiac total anomalous pulmonary venous drainage.AS, atrial septum; LPA, left pulmonary artery; RPA, right pulmonary artery; RSVC, right superior vena cava. In left isomerism, the cardiovascular anomalies are generally not as severe (outlined in Table 30.2) and usually consist of interruption of the inferior vena cava (IVC) with azygos continuation to the right or left superior vena cava; bilateral superior venae cavae; pulmonary veins draining to ipsilateral atria; CAVC in 50%; left ventricular outflow tract obstruction; rarely, TGA; and, uncommonly, pulmonary stenosis (Figure 30.5, Video 30.4). The various cardiac anomalies frequently encountered in heterotaxy syndrome and their incidences in reported series are listed in Table 30.3. Additional details of the encountered anomalies are given in the following sections. Figure 30.5 Typical findings for heterotaxy syndrome with left isomerism. (a) Subxiphoid frontal (long‐axis) view with a common atrium, pulmonary veins (PV) returning to the left side of the atrium, and a transitional atrioventricular canal. (b) The left atrial appendage (LAA) is seen; the right‐sided appendage is not well characterized. The great arteries are normally related with an anterior and leftward pulmonary artery (PA). (c, d) Subxiphoid left axial oblique showing a normal ventriculoarterial relationship and a well‐balanced atrioventricular valve (with two orifices). The patient also has an interrupted inferior vena cava with azygos continuation to the right superior vena cava. Ao, aorta; CAVV, common atrioventricular valve; LV, left ventricle; RSVC, right superior vena cava; RV, right ventricle. Table 30.3 Incidence of cardiac anomalies in heterotaxy syndrome CAVC, complete common atrioventricular canal; DORV, double‐outlet right ventricle; IVC, inferior vena cava; LA, left atrium; LVOT, left ventricular outflow tract; RA, right atrium; TAPVC, totally anomalous pulmonary venous connection; TGA, transposition of the great arteries. For right and left isomerism, bilateral superior vena cavae are common. In patients who have many features of situs inversus there may be absent right superior vena cava with innominate vein to left superior vena cava. Interrupted IVC is considered to be pathognomonic for left isomerism, but rare case reports have shown its association with right isomerism [41,42]. In cases with interrupted IVC the intrahepatic portion of the IVC is missing and the hepatic veins connect with the atrium directly, either as a single trunk or as separate connections of the left and right hepatic veins. The azygos vein carries the blood from below the liver into one of the superior venae cavae and courses parallel to the aorta (Figure 30.6, Video 30.5). When the IVC is intact, the hepatic veins drain through the IVC in the majority of cases, although in about one‐third of the cases the hepatic veins draining the lobe of the liver opposite to the IVC can connect with the atrium directly (Figure 30.7, Video 30.6). In any patient with suspected isomerism, the hepatic venous drainage needs to be identified to inform surgical planning. Figure 30.6 Heterotaxy with left isomerism and interrupted inferior vena cava. Subxiphoid sagittal view with color showing descending aorta (Ao; red) and azygos vein (AV; blue) coursing toward the heart with drainage to the superior vena cava. Figure 30.7 Heterotaxy syndrome with right isomerism. Subxiphoid frontal view with color compare. The right‐sided inferior vena cava (IVC) is seen draining to the right atrium (RA) with a separate entrance of the left hepatic vein (HV) to the left atrium (LA) in a patient with a midline liver. Ao, aorta; PA, pulmonary artery. In right isomerism, totally anomalous pulmonary venous connection to a systemic vein is common (Figure 30.8, Video 30.7). Pulmonary venous obstruction is frequently observed and is associated with poor outcome, particularly in those with a single ventricle [35]. In left isomerism, the pulmonary venous anatomy is normal in the majority of patients. However, malposition of septum primum can result in partially or, rarely, totally anomalous pulmonary venous drainage to the right atrium, though the anatomic course and connections of the pulmonary veins in this anomaly are usually normal (Figure 30.9, Video 30.8). Figure 30.8 Heterotaxy syndrome with right isomerism. Cardiac specimen and echocardiographic correlation. (a) Pathology specimen, viewed from posterior aspect. Infradiaphragmatic total anomalous pulmonary venous connection is demonstrated. Pulmonary veins from each lung are seen entering a vertical confluence with vertical vein (VV) coursing below the diaphragm. (b) Suprasternal frontal view with color compare showing bilateral superior venae cavae, which drain to their respective atria. The pulmonary arteries appear symmetric and are anatomic right pulmonary arteries. Infradiaphragmatic total anomalous pulmonary venous drainage is present. Br, bronchus; LLPV, left lower pulmonary vein; LPA, left pulmonary artery; LSVC, left superior vena cava; LUPV, left upper pulmonary vein; RLPV, right lower pulmonary vein; RPA, right pulmonary artery; RPV, right pulmonary vein; RSVC, right superior vena cava. Figure 30.9 Heterotaxy syndrome with left isomerism. Subxiphoid frontal (long‐axis) view with color compare showing ipsilateral pulmonary venous drainage. In addition, there is leftward displacement of atrial septum primum. ASP, atrial septum primum; LA, left atrium; LPV, left pulmonary vein; RA, right atrium; RPV, right pulmonary vein. In right isomerism, both septum secundum and septum primum may be absent, resulting in a common atrium. In left isomerism, it has been proposed that the partially or totally anomalous pulmonary venous connection to the contralateral atrium is due to malposition of septum primum, probably secondary to the absence of septum secundum [43]. The pulmonary veins appear to be connected normally to the left atrial wall externally but drain to the right atrium anomalously because of the malposed septum primum [44]. Complete unroofing (or absence) of the coronary sinus is commonly associated with right isomerism. In left isomerism, the coronary sinus may be enlarged, particularly if there is interrupted IVC to the left superior vena cava. Partial or complete common atrioventricular (AV) canal is the most common cardiac malformation in all forms of heterotaxy syndrome. In right isomerism, the defect is usually unbalanced with right ventricular dominance. In left isomerism, CAVC is generally balanced with two well‐developed ventricles. Common AV valve regurgitation is common. Determining ventricular looping is essential in heterotaxy syndrome because the atrioventricular and the ventriculoarterial connections are often abnormal. D‐looped ventricles in the context of left isomerism usually possess a solitary AV node, situated where the ventricular septum meets the AV junction postero‐inferiorly; the AV conduction axis is often discontinuous, providing the substrate for AV block that is common in left isomerism. L‐looped ventricles are encountered in 22–38% of patients with right isomerism and in 12–30% of cases with left isomerism [45,46] and usually have dual AV nodes [47]. Preoperative recognition of ventricular looping is important because it can predict the location of the conduction tissue. Abnormal ventriculoarterial alignment and conotruncal anomalies are common features of heterotaxy syndrome. In right isomerism, there is frequently DORV or TGA, often associated with pulmonary outflow obstruction and rarely with aortic outflow obstruction. In contrast, in left isomerism ventriculoarterial alignment is often normal. However, tetralogy of Fallot, DORV, and TGA have all been reported. These patients can also have absence of a subaortic conus, even in cases of TGA [45]. Pulmonary stenosis or atresia is common, especially in right isomerism, and, together with pulmonary venous obstruction, constitutes one of the most severe problems in the neonatal period. This finding is often in the setting where the aorta arises from the RV. A study of 72 postmortem cases of right isomerism [43] reported that when the pulmonary artery branches were severely hypoplastic, stenotic, or discontinuous, anomalous pulmonary venous connections were more probable than in those with pulmonary arteries that were only mildly hypoplastic or normal (86% versus 51% respectively). In postmortem studies [46,48], subaortic obstruction was seen in 4–10% of patients with right isomerism and in 28–33% of patients with left isomerism. More specifically, there was no subaortic conus in any of these cases, and the obstruction was caused by the following lesions [48]: Coarctation of the aorta and interrupted aortic arch are relatively uncommon in heterotaxy syndrome, particularly in right isomerism. In left isomerism, variations of hypoplastic left heart syndrome may be seen. Right aortic arch with mirror‐image branching is common in both subtypes of heterotaxy syndrome, 30–40% in our autopsy series. Isolated left innominate artery, arising solely from ductus arteriosus with no connection to the aorta, has also rarely been described (three cases), with both subgroups of heterotaxy syndrome represented [50]. Another reported association is the presence of bilateral ductus arteriosus in 25% of cases with pulmonary atresia and right isomerism [51]. In right isomerism, in addition to the frequently encountered bronchial symmetry with bilateral trilobed lungs and eparterial bronchi, a midline liver is present in the majority of cases, whereas gastrointestinal and genitourinary anomalies are present in one‐third and one‐tenth of patients, respectively [52]. The most common gastrointestinal abnormality is intestinal malrotation, which has been reported in up to 60% of cases [53]. In left isomerism, extracardiac anomalies are seen in up to 55% of patients [27,54]. Gastrointestinal anomalies are common, with biliary atresia and intestinal malrotation occurring in up to 11% and 34% of patients, respectively Genitourinary and neurologic abnormalities occur in 6% and 4% of patients, respectively. Cyanosis in the newborn period is the presenting symptom in many patients with right isomerism, due to the frequent presence of pulmonary stenosis or atresia, transposition physiology, and/or obstructed totally anomalous pulmonary venous connection. In left isomerism, symptoms of congestive heart failure from a large left‐to‐right shunt in the context of CAVC frequently dominate the clinical presentation. Patients with left isomerism and severe aortic outflow and aortic arch obstruction can present with a clinical picture of systemic hypoperfusion or shock. Rhythm abnormalities are common in both groups of heterotaxy syndrome. There are dual sinus nodes in the majority of right isomerism patients, whereas in left isomerism the sinus node tends to be hypoplastic or absent, resulting in abnormal origin of the atrial rhythm (often low atrial rhythm) [47]. Bradycardia and complete AV block mainly affects patients with left isomerism and has been traditionally thought to be associated with near 100% mortality. However, a recent series showed 1‐year survival of 63% in fetuses diagnosed with bradycardia or complete AV block (n = 15, 4 bradycardia and 11 complete AV block) [55]. The high occurrence of complete AV block has been attributed to discontinuity between the AV node and the ventricular conduction tissue [56]. Postnatally, it has been described in 7% of patients with left isomerism [28]; additionally, a small percentage of patients develop complete AV block postoperatively. In those with left isomerism and complete AV block, noncompaction of the ventricular myocardium may occur with development of ventricular dysfunction, often despite early pacing (Figure 30.10, Video 30.9). Figure 30.10 Heterotaxy with left isomerism. Apical four‐chamber view showing biventricular noncompaction. There is a common atrium (CA) and partial atrioventricular canal defect. There is severe right ventricular dilation and biventricular function is poor. This patient had congenital complete heart block. LV, left ventricle; RV, right ventricle; VS, ventricular septum. The splenic abnormalities that accompany heterotaxy syndrome contribute significantly to morbidity and mortality. Asplenia can result in fulminant sepsis and patients require lifelong antibiotic prophylaxis. Splenic imaging is therefore important and can include abdominal ultrasound, CT, or magnetic resonance imaging. Because functional asplenia can develop with age [57], it is recommended that patients with heterotaxy syndrome, even with documented multiple or solitary spleens, are monitored regularly for splenic function. The highly variable and often complex anatomy of heterotaxy syndrome requires a systematic segmental approach to imaging.
CHAPTER 30
Cardiac Malposition and Heterotaxy Syndrome
Cardiac malposition
Definitions
Incidence
Etiology
Morphology and classification
Developmental considerations
Anatomy
Pathophysiology
Imaging
Dextrocardia and situs inversus
Isolated ventricular inversion
Prenatal assessment
Pre‐ and postoperative assessment
Heterotaxy syndrome
Definitions
Incidence
Etiology
Morphology and classification
Developmental considerations
Anatomy
Diagnosis of heterotaxy syndrome requires the presence of three or more of the following criteria
Characteristic congenital heart disease
Pulmonary venous anomalies:
Totally anomalous pulmonary venous connection or drainage
Partially anomalous pulmonary venous connection or drainage
Systemic venous anomalies:
Bilateral superior venae cavae
Interrupted inferior vena cava
Absent coronary sinus septum
Atrial anomalies:
Atrial situs ambiguous or inversus
Common atrium
Common atrioventricular canal (septal) defects:
Complete atrioventricular canal defect
Partial (incomplete) atrioventricular canal defect
Transitional atrioventricular canal defect
Ventricular abnormalities:
Hypoplastic or single left ventricle
Hypoplastic or single right ventricle
Ventricular malposition (e.g., L‐loop, superior‐inferior, crisscross)
Ventriculoarterial alignment abnormalities:
Double‐outlet ventricle
D‐loop transposition of great arteries
L‐loop transposition of great arteries
Truncus arteriosus
Tetralogy of Fallot
Ventricular outflow abnormalities:
Subvalvar and valvar pulmonary stenosis
Pulmonary atresia with intact ventricular septum
Pulmonary atresia with ventricular septal defect (not tetralogy of Fallot type):
Valvar or subvalvar aortic stenosis
Coarctation of the aorta
Biliary atresia
Absent gallbladder
Abdominal situs abnormality:
Abdominal situs inversus
Situs ambiguous (midline or transverse liver, midline aorta, ipsilateral aorta and inferior vena cava)
Spleen abnormality (confirmed by imaging, autopsy, or by Howell–Jolly bodies):
Asplenia
Polysplenia
Single right‐sided spleen
Isomerism of bronchi:
Bilateral left bronchial morphology
Bilateral right bronchial morphology
Isomerism of lungs
Bilateral two lobes (left‐sidedness)
Bilateral three lobes (right‐sidedness)
Similar morphology of atrial appendages
Intestinal malrotation
Malrotation or nonrotation colon
Malrotation small intestine
Right isomerism/asplenia
Left isomerism/polysplenia
Atrioventricular (AV) connections
Atrioventricular canal
Atrioventricular canal
Ventricular anatomy
Single or dominant right ventricle
Two well‐developed ventricles
Ventriculoarterial (VA) alignment
DORV, TGA
Most with normal VA alignment
Outflow obstruction
Pulmonary stenosis or atresia
Subaortic/aortic stenosis
Pulmonary veins
Totally anomalous pulmonary venous return to a systemic vein
Ipsilateral pulmonary venous drainage
Systemic veins
Bilateral superior venae cavae
Bilateral superior venae cavae
Absent coronary sinus
Interrupted inferior vena cava
Abnormal hepatic vein connection to heart
Conduction system
Bilateral sinus nodes
Complete heart block
Paired AV nodes
Right isomerism/asplenia syndrome (%) [25,34,38,52]
Left isomerism/polysplenia syndrome (%) [25,38,49]
Dextrocardia
33–37
27–45
Interrupted IVC
0–3
65–80
Hepatic veins entering the atria directly
17–38
83–86
Unroofed coronary sinus
32–100
31–41
Bilateral superior venae cavae
38–71
47–62
TAPVC to a systemic vein
42–64
0–13
Pulmonary veins to RA (partial or total)
21
39–60
Common atrium
40–84
10–53
Atrial situs solitus
50
78
Atrial situs inversus
31
22
Atrial situs ambiguous
19
0
CAVC
81–92
16–66
Pulmonary stenosis/atresia
79–84
40–43
Aortic valve hypoplasia/LVOT obstruction/coarctation
2–15
12–28
Single ventricle
42–73
26
TGA or DORV
45–75
31–45
Abnormal coronary pattern
10–64
8–24
Systemic venous connections
Pulmonary venous connections
Atrial septum
Coronary sinus
Atrioventricular valves
Ventricles
Ventriculoarterial alignment
Pulmonary outflow tract obstruction
Aortic outflow tract obstruction
Aortic arch, brachiocephalic vessels, and patent ductus arteriosus
Noncardiac anomalies
Pathophysiology
Imaging
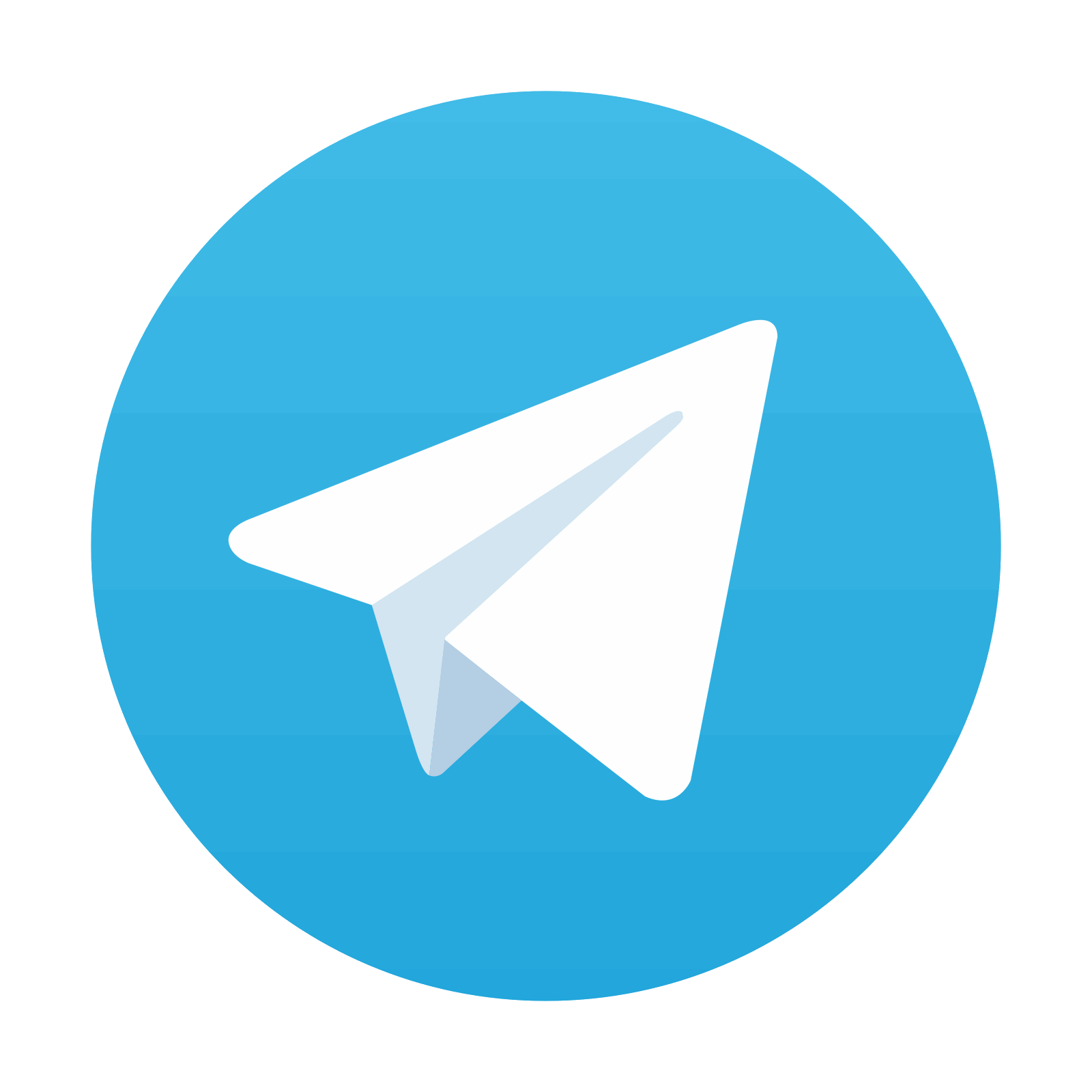
Stay updated, free articles. Join our Telegram channel

Full access? Get Clinical Tree
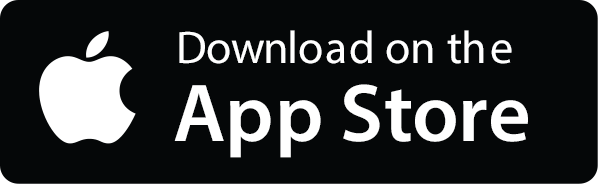
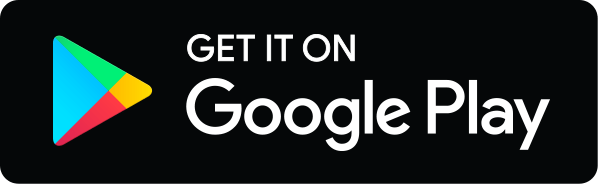