Xavier Iriart1, Meryl S. Cohen2, and Luc L. Mertens3 1 Bordeaux University Hospital (CHU), Pessac, France 2 The Children’s Hospital of Philadelphia, Philadelphia, PA, USA 3 The Hospital for Sick Children; University of Toronto, Toronto, ON, Canada Transposition of the great arteries (TGA) is a cardiac abnormality in which the aorta is aligned with the right ventricle (RV) and the pulmonary artery is aligned with the left ventricle (LV) resulting in discordant ventriculoarterial connections (Figure 25.1). This anomaly can occur in isolation or in association with other cardiac defects, most commonly ventricular septal defect (VSD). In this chapter we will focus on discordant ventriculoarterial connections in the presence of concordant atrioventricular connections. Congenitally corrected transposition (ventriculoarterial discordance with atrioventricular discordance) is discussed in Chapter 27. Transposition of the great arteries is also referred to as D‐TGA. This is, however, a nonspecific term as the “D” may relate to the ventricular loop (D or L) or to the spatial position of the aortic and pulmonary valves. In the majority of patients with TGA, the aorta is malpositioned rightward and anterior to the pulmonary artery but a wide variability in the spatial relationship between the great arteries may occur. Therefore, the term D‐TGA is better avoided; D‐loop TGA may be more accurate where the D‐loop refers to the ventricular looping. For the purpose of this chapter, discordant ventriculoarterial connections will be referred to as TGA. The physiology of TGA is quite unique. The systemic and pulmonary circulations are in parallel; the deoxygenated systemic venous blood returns to the aorta via the RV and the oxygenated pulmonary venous blood returns to the pulmonary arteries through the LV (Figure 25.1). Clinical presentation generally occurs in the immediate neonatal period because of cyanosis. Surgery in the neonatal period is the norm and, if the anatomy is suitable, the most common procedure is the arterial switch operation. The incidence of TGA is approximately 31.5 per 100,000 live births (range 23.1 to 38.8 per 100,000 live births). It is the tenth most common congenital heart defect and the second most common cyanotic lesion after tetralogy of Fallot [1]. TGA affects males more commonly than females with a ratio of over 2 : 1 [2]. Chromosomal anomalies in association with simple and complex forms of TGA are unusual. The incidence of TGA does not appear to be impacted by increased maternal age or increased parity [2]. The etiology of TGA remains unknown, although a genetic cause is probable in most cases. Familial recurrence may occur in TGA; 10% of afflicted individuals have a relative with a congenital heart defect. While 22q11.2 deletion is common in other anomalies of the great arteries such as tetralogy of Fallot and truncus arteriosus, it is quite rare in TGA [3]; however, single gene mutations such as CFC1 have been reported [4]. Several families have been reported in which some members had TGA, and others had heterotaxy syndrome or congenitally corrected TGA [5]. Mutations of some of the genes involved in laterality defects have been found in these families. Environmental factors have been implicated as well; TGA has been associated with maternal diabetes [6–8] and exposure to the teratogen retinoic acid during gestation. in vitro; fertilization is another risk factor [9]. Maternal exposure to pesticides (herbicides and rodenticides) [10] during the first trimester of pregnancy, as well as intake of antiepileptic [11] and other medications, have also been reported [12]. The developmental mechanisms leading to TGA have not been fully elucidated, especially because TGA is difficult to reproduce in animal models. The embryologic mechanism of TGA has classically been explained by two main theories. The first theory formulated by Goor and Edwards in the 1970s [13], called the “infundibular theory,” suggests that TGA could be related to an underdevelopment of the subpulmonary muscular conus with an abnormal persistence of the subaortic muscular conus, leading to a lack of the normal clockwise rotation of the aorta toward the left ventricle. Figure 25.1 Diagram of transposition of the great arteries (atrioventricular concordance and ventriculoarterial discordance). Ao, aorta; LA, left atrium; LV, left ventricle; MPA, main pulmonary artery; RA, right atrium; RV, right ventricle. The second theory formulated by De la Cruz [14] in the 1980s focuses on the abnormal spiralization of the aortopulmonary septum. In this theory, TGA results from linear rather than spiral development of the aortopulmonary septum, which puts the fourth aortic arch (the future aorta) in contact with the anterior muscular conus of the right ventricle. More recent studies have demonstrated that spiraling migration of the right second heart field [15] is required for elongation and appropriate alignment of the cardiac outflow tract [16], and emphasizes the pivotal role of Pitx 2 at the arterial pole of the developing heart [17–19]. In the most common form of TGA, the segmental anatomy is typically situs solitus with atrioventricular concordance and ventriculoarterial discordance with the aorta positioned anterior and to the right of the pulmonary artery. This has been described as {S,D,D}: the situs of the visceral organs and the atria is normal (solitus = S), the ventricles are D‐looped (D), the atrioventricular alignment is concordant, and the ventriculoarterial alignment is discordant with a rightward position of the aortic valve relative to the pulmonary valve (D, or dextro malposition). In some cases, the aorta is directly anterior to the pulmonary artery and in others the great vessels are side by side with the aortic valve typically to the right of the pulmonary valve. Rarely, the aorta can be leftward, in which case the segmental anatomy is {S,D,L}. This arrangement is typically associated with RV hypoplasia and can be seen in superior–inferior ventricles. More rarely, the aortic valve can be located posterior and rightward relative to the pulmonary valve (TGA with posterior aorta) [9]. In the setting of situs inversus totalis (mirror image), the segmental anatomy of TGA would be {I,L,L}. The anatomy of the ventricular outflow tracts in TGA is important for surgical management. In almost 90% of cases of TGA there is a subaortic conus (infundibulum) separating the aortic valve from the tricuspid valve and absence of a subpulmonary conus (and thus, fibrous continuity between the mitral and pulmonary valves) [20,21]. Variants of conal anatomy in TGA are seen in ~12% of patients – bilateral subarterial conus in ~7%, subpulmonary conus without subaortic conus in ~3%, and bilaterally absent conus in ~2% of patients [22]. Abnormalities in the outflow tracts can cause outflow tract obstruction, which is important when considering an arterial switch operation. Dynamic LV outflow tract obstruction can be seen when LV pressure is subsystemic. This usually resolves after anatomic correction. Fixed anatomic LV outflow tract obstruction can be the result of a discrete fibrous membrane or ridge, abnormal mitral valve attachments, or septal hypertrophy (Figure 25.2). The foramen ovale is usually patent at birth but can be small or absent, resulting in severe cyanosis due to lack of atrial mixing. A true secundum atrial septal defect or other types of atrial defects are less common. A left aortic arch without obstruction is the norm although right aortic arch can occur. Rare cases of coarctation of the aorta have been reported [23] even with intact ventricular septum. Arch obstruction is common in the setting of anterior malalignment type VSD. Systemic and pulmonary venous connections are typically normal. Most patients with TGA have a patent ductus arteriosus (PDA) at birth. A VSD is the most commonly associated defect in TGA occurring in ~40–45% of cases. Similar to normally related great arteries, any type of VSD can be seen in association with TGA. Approximately 33% patients have a central perimembranous defect (conoventricular, perimembranous), ~30% have a malalignment defect (often associated with obstruction of one of the outflow tracts), ~25% have a muscular defect, and ~5% each have an inlet (atrioventricular canal type) or outlet (doubly committed or conal septal) defect (Figure 25.3) (see Chapter 13 for details on VSD terminology) [24]. Central perimembranous defects may be large and result in fibrous continuity between the tricuspid and pulmonary valves. Spontaneous closure or restriction of the defect by tricuspid valve septal tissue is also possible. Muscular defects can occur anywhere within the trabecular muscular septum and may be multiple. Muscular defects may also close or decrease in size spontaneously. In those with inlet VSD, the tricuspid valve may straddle the ventricular septum, usually with associated RV hypoplasia. Outlet defects are characterized by deficiency or absence of the outlet (conal) septum with little or no muscular separation between the aortic and pulmonary valves (Figure 25.3). Surgical closure of this type of VSD can be challenging because the sutures must be anchored into the fibrous rim between the aortic and pulmonary valves, potentially distorting one or both semilunar valves. Figure 25.2 Diagram showing the different mechanisms of left ventricular outflow obstruction in transposition of the great arteries. More than one mechanism may be present. LV, left ventricle; MPA, main pulmonary artery; MV, mitral valve; PS, pulmonary stenosis; VSD, ventricular septal defect. Figure 25.3 Diagram of the different types of ventricular septal defects in the setting of transposition of the great arteries. AV, atrioventricular. Malalignment‐type VSDs are of particular surgical importance. In the setting of anterior malalignment of the outlet (conal) septum, the VSD is generally large and unrestrictive. This anatomy typically results in impingement on the RV outflow tract with multiple levels of obstruction. Subaortic stenosis and aortic annulus hypoplasia are common; distal obstruction can include aortic arch hypoplasia, coarctation of the aorta, or interruption of the aortic arch. In contrast, posterior malalignment of the outlet septum is often associated with LV outflow tract obstruction, including subvalvar and valvar pulmonary stenosis. The pulmonary valve annulus may be hypoplastic and the pulmonary valve leaflets and commissures are often abnormal (e.g., bicuspid or unicuspid pulmonary valve). Importantly, patients with TGA with posterior malalignment VSD usually cannot undergo the arterial switch operation (ASO) because subaortic obstruction may be severe after surgery. Coronary artery anatomy is particularly important in TGA given that the majority of patients with this diagnosis will undergo the ASO, which requires translocation of the coronary arteries to the neoaorta. Although the origin and proximal course of the coronary arteries are variable, they originate from aortic sinuses facing the pulmonary valve in almost all cases. The aortic sinuses that face the pulmonary root are labeled according to their spatial location [25]. In the majority of patients in whom the aortic valve is anterior and rightward relative to the pulmonary valve, the sinuses are termed right‐posterior‐ and left‐anterior‐facing sinuses. When the aortic valve is directly anterior the sinuses are termed right‐ and left‐facing and when the great vessels are side by side the sinuses are termed posterior‐ and anterior‐facing (Figure 25.4). Alternative taxonomies assign numbers or letters to the facing sinuses. The Leiden terminology describes the origin of the coronary arteries from the facing aortic sinuses of Valsalva as viewed from the nonfacing sinus [26]. The observer takes a stance in the noncoronary sinus looking toward the pulmonary trunk (Figure 25.4). Viewed from this position, one sinus is always to the right of the observer (sinus 1) and the other sinus is always to the left of the observer (sinus 2). This definition holds irrespective of the relationship of the arterial trunks towards each other. While the Leiden classification is convenient for the surgeon who looks down on the aortic valve, this classification can be confusing for the echocardiographer who looks at the aortic valve from the opposite perspective. If uncertain about the description, a schematic drawing of the coronary origins as illustrated in Figure 25.5 may clarify the issue. The eight major anatomic patterns of the coronary arteries in TGA are illustrated in Figure 25.5. Based on three studies encompassing 533 patients [27], the most common or “usual” pattern of coronary artery origin – left anterior descending (LAD) and circumflex (Cx) arising from the left‐facing sinus (sinus 1) and the right coronary artery (RCA) arising from the right‐facing sinus (sinus 2) – is present in approximately two‐thirds of patients. The second most common form – LAD from the left‐facing sinus (sinus 1) and RCA and Cx from the right‐facing sinus (sinus 2) – is present in 13–14% of patients. The Cx courses posterior to the pulmonary root before reaching the left atrioventricular groove. This variation of coronary arteries is more common in TGA when the aorta and pulmonary artery are more side by side than anterior–posterior. The third most common pattern seen in 7–8% of cases is a single RCA arising from the right‐facing sinus (sinus 2) where the left coronary artery (LCA) passes posterior to the pulmonary root before bifurcating into the LAD and Cx branches. Other variations include a single LCA from the left‐facing sinus (sinus 1) in 1–2% of cases, inverted coronary arteries (RCA from sinus 1 and LAD and Cx from sinus 2) in 2–3% of cases, and partially inverted coronary arteries (RCA and LAD from sinus 1 and Cx from sinus 2) in 6% of cases. Intramural coronary arteries are single coronary arteries that take a course between the aorta and pulmonary artery within the wall of the aorta. Intramural LCAs and RCAs are seen in 3% and 1% of patients, respectively. Based on analysis of nine studies from over two decades (total of 1942 patients), intramural or single coronary artery patterns were associated with an increased risk of death in patients undergoing an ASO [27]. Figure 25.4 Designations of the aortic sinuses of Valsalva when the spatial orientation of the aortic and pulmonary roots is oblique, anterior–posterior, and side by side. Transposition of the great arteries has been associated with a myriad of cardiovascular abnormalities such as anomalous systemic veins (e.g., persistent left superior vena cava) and pulmonary veins (e.g., partially and totally anomalous pulmonary venous connections [28]), atrioventricular canal defect, anomalies of the atrioventricular valves (e.g., stenosis, cleft, abnormal attachments, and straddling [29]), left and right ventricular outflow tract abnormalities, and anomalies of the great vessels (aortic arch hypoplasia, interruption [30], coarctation, and double aortic arch [31]). Leftward juxtaposition of the right atrial appendage occurs in approximately 2% of cases of TGA [32]. It is usually seen in association with other complex abnormalities such as dextrocardia or tricuspid atresia. Figure 25.5 The most common patterns of coronary artery anatomy in patients with transposition of the great arteries (D‐looped). (a) Usual coronary anatomy. (b) Left circumflex artery (LCx) from right coronary artery (RCA). (c) Single RCA. (d) Single left coronary artery (LCA). (e) Inverted origins with the RCA coming from the left‐facing sinus and the LCA coming from right‐facing sinus. (f) Partially inverted origins with the left anterior descending (LAD) artery and RCA coming from the left‐facing sinus and the LCx coming from the right‐facing sinus. (g) Intramural LCA from the right‐facing sinus. (h) Intramural RCA from the left‐facing sinus. The parallel arrangement of the systemic and pulmonary circulations in TGA results in poor effective pulmonary blood flow even though overall pulmonary blood flow is high. This physiology is tolerated well before birth due to mixing of oxygenated and deoxygenated blood through the foramen ovale and patent ductus arteriosus, although it should be noted that the aorta and thus the cerebral circulation receives more deoxygenated blood than in the normal heart. After birth, the decrease in pulmonary vascular resistance leads to increased pulmonary venous return to the left atrium, which is accentuated by flow from the aorta to the pulmonary artery through the ductus arteriosus. If the septum primum (valve of the fossa ovalis) effectively seals off the intra‐atrial communication, severe life‐threatening hypoxia may occur within the first hours after birth. In other cases, the foramen ovale remains open, allowing oxygenated blood returning to the left atrium to mix with the deoxygenated blood returning from the systemic veins. The presence of an adequate atrial communication is generally a requirement to obtain adequate aortic (preductal) saturations. If the atrial communication is inadequate, a balloon atrial septostomy may be required. When present, a VSD provides another opportunity for mixing of blood between the left and right sides of the heart, although adequate mixing at this level does not always occur. In the weeks after birth, pulmonary vascular resistance decreases and, in the absence of a large VSD or outflow tract obstruction, the afterload on the LV decreases. This causes the LV to remodel with a thinner free wall and a smaller short‐axis diameter. The RV meanwhile remains hypertrophied and dilated. Ultimately, LV mass decreases (deconditioning) to a level that precludes its ability to sustain the systemic circulation after the ASO. Thus, anatomic repair is performed in patients with TGA and intact ventricular septum or a small VSD within the first days to weeks of life in order to avoid LV remodeling. For patients presenting after age 2 months, careful assessment of LV size, wall thickness, and mass is required. Another factor that can lead to hemodynamic instability after birth is the presence of elevated pulmonary vascular resistance or persistent pulmonary hypertension. Echocardiography is the imaging modality of choice in children with TGA, and currently most patients are referred for cardiac surgery based solely on echocardiographic diagnosis. Therefore, a comprehensive, detailed description of cardiovascular anatomy and suitability for surgical repair is paramount for successful management. In addition, echocardiography is often used as the primary imaging modality to guide balloon atrial septostomy. The subxiphoid acoustic window provides a wide‐angle view of the heart and blood vessels and allows rapid and accurate diagnosis of TGA. After establishing the situs of the abdominal organs, a sweep of the transducer in the subxiphoid long‐axis (frontal) plane demonstrates the systemic and venous connections to the heart, the atrial septum, atrioventricular and ventriculoarterial connections and alignments, and the great vessels. As the transducer is swept from posterior to anterior (Video 25.1), the main pulmonary artery is seen originating directly from the LV bifurcating into the left and right pulmonary arteries (Figure 25.6a). Further anterior tilt of the transducer demonstrates the origin of the aorta from the rightward and superior aspect of the RV (Figure 25.6b). Origin of the coronary arteries from the aortic root, often seen from this window, helps establish the identity of the aorta. The great arteries are generally noted to arise in parallel rather than crossing each other as is seen in the normal heart. This view can also identify juxtaposition of the atrial appendages. Rotating the transducer clockwise ~90° to the subxiphoid short axis (oblique sagittal) plane demonstrates the superior–inferior and anterior–posterior relations of the cardiovascular structures. The transducer is swept from right to left, beginning at the bicaval view and ending at the level of the ventricular apex. This view is particularly helpful in highlighting the atrial septum, and the anatomy of the atrioventricular valves and their attachments, and the outflow tracts of both ventricles. In TGA, this view also nicely demonstrates the parallel course of the great arteries (Figure 25.7). Interrogation of blood flow by color Doppler provides essential information about atrial and ventricular septal defects, valve function, outflow tract obstruction, semilunar valve function, and ductus arteriosus. Evaluation of the atrial septum and adequacy of the atrial communication sometimes needs to be determined urgently. Subxiphoid sweeps are best to determine the location and size of interatrial communication. Adequacy of the atrial communication is based on size, but pulse oximetry and PO2 will also determine need for balloon atrial septostomy to provide adequate mixing. Preoperative atrial level shunting is almost always from left atrium to right atrium. The mean gradient of flow across the atrial septum can be measured by continuous‐wave (CW) spectral Doppler. Figure 25.6 Subxiphoid long‐axis (frontal) view showing ventriculoarterial discordance in transposition of the great arteries. (a) The main pulmonary artery (PA) is seen arising from the left ventricle (LV). (b) Sweeping anteriorly demonstrates the aorta (Ao) arising from the right ventricle (RV). RA, right atrium. Figure 25.7(a) Subxiphoid short‐axis (sagittal) view showing parallel great arteries with the aorta (Ao) anterior to the pulmonary artery (PA). (b) Color Doppler mapping showing a patent ductus arteriosus (PDA) connected to the posterior PA with left‐to‐right shunt. LV, left ventricle; RV, right ventricle. The apical window allows evaluation of the ventricular inflow and outflow tracts and the ventricular septum. This view is ideal for assessment of the atrioventricular valves for stenosis or regurgitation by color and spectral Doppler. Careful interrogation of the ventricular septum with particular attention to the apex is important to exclude muscular VSDs. In addition, the apical window provides an optimal angle for Doppler interrogation of the LV outflow tract to assess for obstruction. The parasternal window provides complementary anatomic and functional information. The parallel course of the great arteries is apparent in the parasternal long‐axis view where both great arteries can usually be imaged longitudinally in the same plane as they both dive posteriorly (Figure 25.8, Video 25.2). Although a parallel course of the aorta and main pulmonary artery in the same plane is not seen with normally related great arteries, it is important to recognize that it is not unique to TGA. For example, this anatomic arrangement can also be seen in double‐outlet RV and congenitally corrected TGA. The parasternal long‐axis view also demonstrates the fibrous continuity between the mitral and pulmonary valves if present. The aortic valve is seen anterior to the pulmonary valve and is supported by an infundibulum. Left juxtaposition of the atrial appendages is suspected on a parasternal long‐axis view as an oval structure lying between the main pulmonary artery and the left atrium and can be further confirmed on the parasternal short‐axis view (Figure 25.9). Figure 25.8 Parasternal long‐axis view showing parallel orientation of the great arteries as they dive posteriorly. Fibrous continuity between the mitral and the pulmonary valve can be seen indicating that there is no subpulmonary conus. Ao, aorta; LV, left ventricle; PA, pulmonary artery; RV, right ventricle. The parasternal short‐axis plane is used to determine the spatial relationships between the semilunar valves and great arteries (Figure 25.10, Video 25.3). The parallel orientation of the aorta and main pulmonary artery accounts for their simultaneous appearance in cross‐section as two circles. Most commonly, the aortic valve is anterior and to the right of the pulmonary valve but other relationships (e.g., side by side, directly anterior, leftward) are possible. Imaging from the high left parasternal position often optimizes this view. In most patients it is possible to obtain an image where the commissures between the aortic and pulmonary valve leaflets can be viewed simultaneously (Figure 25.11). The parasternal short‐axis view is useful for assessing commissural alignment between the valve leaflets, which is important for coronary artery transfer during the ASO (Video 25.4). In some patients, this view cannot be obtained due to a more superior position of the aortic valve relative to the pulmonary valve. Figure 25.9 Juxtaposition of the atrial appendages. (a) Parasternal long‐axis view showing left juxtaposition of the atrial appendages. The leftward position of the right atrial appendage (RAA) is seen between the main pulmonary artery (PA) and the left atrium (LA). (b) Parasternal short‐axis view showing the RAA lying posterior and to the left of the transposed great arteries. (c) Anatomic specimen demonstrating left juxtaposition in transposition of the great arteries with the RAA sitting on top of the left atrial appendage (LAA). Ao, aorta; LV, left ventricle; RA, right atrium. Source: Courtesy of Lucile Houyel, MD, Université Paris‐Sud. Assessment of biventricular size and function can be performed in multiple views. Assessment includes ventricular dimensions, wall thickness, and measures of systolic and diastolic performance. Biventricular systolic function is usually preserved in infants with TGA unless severe hypoxia and metabolic acidosis is present. 3D echocardiography does not add a great deal to the preoperative assessment of TGA with intact ventricular septum. Description of the location of VSDs in TGA is identical to patients with concordant ventriculoarterial alignments (see Chapter 13). A central perimembranous VSD, the most common defect in TGA, may be imaged from several imaging planes, including subxiphoid long‐and short‐axis, apical, parasternal short‐axis, and tilted long‐axis views (Figure 25.12, Video 25.5). In contrast to normally related great arteries, the parasternal short‐axis view in TGA does not highlight these defects as well because of the abnormal relationship of the great arteries. Figure 25.10 Parasternal short‐axis view demonstrating the spatial relationship of the great arteries. (a) In the most common form of transposition of the great arteries, the aorta (Ao) is anterior and to the right of pulmonary artery. (b, c) The aorta can also be directly anterior to the pulmonary artery (b) or side by side, with the aortic valve to the right of the pulmonary artery (c). (d) In more unusual cases, the aorta is anterior and the left of the pulmonary artery. Even rarer, the aorta can be posterior. Figure 25.11 Parasternal short‐axis view of the aortic and pulmonary valves in transposition of the great arteries. (a) Commissural alignment of the aortic and pulmonary valves. (b) Commissural malalignment with an aortic‐facing commissure (solid arrows) and pulmonary‐facing commissure (dotted arrows). Ao, aorta; PA, pulmonary artery. Figure 25.12 Transposition of the great arteries with a central perimembranous ventricular septal defect (VSD). (a). Subxiphoid left anterior oblique view in color compare mode demonstrating the VSD under the pulmonary valve shunting from the right ventricle (RV) to the left ventricle (LV). (b) Parasternal long‐axis view demonstrating shunting from the RV to the LV. Ao, aorta; PA, pulmonary artery. Figure 25.13(a) Transposition of the great arteries with anterior malalignment‐type VSD. Apical five‐chamber view demonstrating the parallel orientation of the great vessels. An anterior malalignment VSD is visualized with the outlet (conal) septum deviated anteriorly and rightward into the right ventricular outflow tract (arrow). (b) There is subaortic flow acceleration seen on color Doppler. (c) Suprasternal view of the semilunar valves demonstrating the smaller aorta (Ao) sitting almost directly anterior to the pulmonary artery (PA) in the setting of anterior malalignment VSD. The left coronary artery (LCA) is also seen arising from the left‐facing cusp of the aorta. Imaging in multiple cross‐sectional planes identifies muscular VSDs. The location of these defects is variable and requires detailed interrogation of the entire septum by 2D imaging and color Doppler. The apical four‐chamber view is particularly helpful for evaluation of the ventricular septum, although the anterior–superior aspects of the septum are not seen optimally in this view. The apical view best highlights the basal (inlet) and apical segments of the septum. The subxiphoid and parasternal short‐axis views are helpful in evaluating the anterior–superior and posterior–inferior aspects of the septum as well as the midseptum. Moderate to large defects can be easily detected using 2D imaging whereas small defects are usually best visualized using color Doppler. Lowering the Nyquist limit of the color Doppler scale is important to delineate VSDs in TGA given the often equal or near‐equal pressures in the LV and RV. Defects with anterior malalignment of the outlet (conal) septum are readily seen in most imaging planes (Figure 25.13, Video 25.6). The subxiphoid sagittal (short‐axis) view is particularly suitable to visualize the displaced outlet (conal) septum and associated RV outflow obstruction. This view is also used to measure the pressure gradient across the outflow using pulsed‐wave (PW) and CW Doppler. The presence of RV outflow tract narrowing should prompt careful assessment of the aortic arch and isthmus for obstruction or interruption. Defects with posterior malalignment of the outlet (conal) septum are best viewed from the subxiphoid frontal (long‐axis), left anterior oblique, and sagittal (short‐axis) views and from the parasternal long‐axis view (Figure 25.14, Video 25.7). Important elements of the echocardiographic evaluation include assessment of etiology and severity of LV outflow tract obstruction. The gradient across the LV outflow tract can be measured by PW and CW Doppler from the apical window. It should be noted, however, that as long as the PDA is large and pulmonary hypertension persists the gradient can be small despite significant obstruction. Figure 25.14 Transposition of the great arteries with various forms of left ventricular outflow tract obstruction. (a) Subxiphoid short‐axis (sagittal) view showing a posterior malalignment‐type VSD with posterior deviation of the outlet (conal) septum (arrow) causing tunnel‐like narrowing of the left ventricular outflow tract. (b) Subxiphoid short‐axis view showing left ventricular outflow tract obstruction as a result of abnormal attachment of the mitral valve (MV) apparatus to the outlet septum (star). The arrow indicates the VSD. (c) Parasternal long‐axis view showing left ventricular outflow tract obstruction as a result of tissue tags coming from the tricuspid valve apparatus. Ao, aorta; LV, left ventricle; PA, pulmonary valve; RV, right ventricle An inlet VSD in association with TGA is unusual but when seen it is often associated with tricuspid valve anomalies including abnormal chordal attachments to the crest of the ventricular septum, overriding, or straddling of the valve. Straddling the tricuspid valve can complicate surgical closure of the VSD and requires precise preoperative imaging. 3D imaging to assess the tricuspid valve and its attachments can be very useful in this situation. Inlet VSD is best imaged in the apical four‐chamber view but can also be visualized from other acoustic windows (Figure 25.15, Video 25.8). A posteriorly angled apical four‐chamber view shows the defect at the base of the ventricular septum with the atrioventricular valve annulus forming the superior–posterior border of the defect. Abnormal tricuspid valve attachments or accessory tissue related to the valve can prolapse through the VSD causing variable degrees of LV outflow tract obstruction. Figure 25.15 Transposition of the great arteries with inlet VSD. Subxiphoid short‐axis (sagittal) view centered on the tricuspid demonstrating the large inlet VSD and straddling of the tricuspid valve (TV) (star). The VSD (arrow) is seen at the inlet/posterior portion of the interventricular septum. LV, left ventricle; RV, right ventricle. VSD with hypoplasia or absence of the outlet (conal) septum (doubly committed conal septum) is unusual but can be seen in TGA. Subxiphoid and parasternal images demonstrate the absence or hypoplasia of the outlet (conal) septum with typically fibrous continuity between the aortic and pulmonary valve leaflets (Figure 25.16, Video 25.9). Echocardiographic delineation of coronary artery anatomy in TGA can be challenging but is important to surgical strategy, particularly for the ASO. Optimal imaging conditions, including proper position of the infant, removal of obstacles to imaging from the chest wall, and imaging when the patient is calm or asleep, are crucial for comprehensive evaluation of the origin and course of the coronary arteries. Imaging is performed predominantly from parasternal windows but complementary information is gleaned from subxiphoid and apical views. The modified high parasternal short‐axis view is used to image the origins of the coronary arteries (Figure 25.17). For viewing the origin and proximal course of the LCA, a slight clockwise rotation is used, reaching a transverse view with the transducer’s index mark at ~3 o’clock. For viewing the origin and proximal course of the RCA, a slight counterclockwise rotation of the transducer with the index mark at ~1 o’clock is helpful (Video 25.10). In those with usual coronary anatomy, a parasternal long‐axis view through the aortic root helps to visualize the origin of the RCA from the posterior sinus. When sweeping the transducer in the long‐axis view towards the left shoulder, the bifurcation of the LCA into the proximal LAD and Cx can be imaged. When the Cx originates from the right posterior‐facing sinus (sinus 2), the posterior course of the coronary artery relative to the pulmonary artery can be seen in parasternal short‐axis view and confirmed in the apical four‐chamber and subxiphoid views (Figure 25.18). An anterior course of the coronary arteries (Figure 25.19), or combined anterior and posterior course relative to the aorta and pulmonary artery, are present in cases of single coronary arteries arising from one sinus or in the inverted patterns (Video 25.11). Subxiphoid and apical views can also be helpful for delineating the relationship of the coronary arteries to the great vessels (Figure 25.18). Figure 25.16 Transposition of the great arteries with an outlet‐type ventricular septal defect (VSD). (a) Parasternal long‐axis view demonstrating outlet‐type VSD with fibrous continuity between the aortic and pulmonary valves. (b) Parasternal short‐axis view demonstrating the large outlet VSD just below the aortic and pulmonary valves; note that it is at 2 o’clock, similar to an outlet defect with normally aligned great arteries. Ao, aorta; LA, left atrium; LV, left ventricle; PA, pulmonary artery; RA, right atrium; RV, right ventricle. Figure 25.17 Parasternal short‐axis views showing (a) the origin of the right coronary artery (RCA) originating from right‐facing sinus and (b) the left coronary artery arising from the left‐facing sinus bifurcating in the left anterior descending artery (LAD) and circumflex artery (Cx). Note that coronary flow toward the myocardium can be seen on color Doppler by lowering the Nyquist limit. Figure 25.18 Variations of coronary artery anatomy in transposition of the great arteries. (a) High parasternal short‐axis view showing the proximal part of the right coronary artery (RCA) arising from the right‐facing sinus with the circumflex artery (Cx) originating from the RCA and coursing posterior to the pulmonary artery (PA) (arrows). (b) Subxiphoid long‐axis view tilted posterior to the PA, where the Cx is seen crossing posterior to the great arteries to the atrioventricular groove (arrows). (c) Subxiphoid short‐axis view showing the common origin of the RCA and the Cx from the right‐facing sinus. (d) Color Doppler showing the coronary artery flow in the posterior Cx achieved by lowering the Nyquist limit (arrow). Ao, aorta; LA, left atrium; LV, left ventricle; RA, right atrium; RV, right ventricle. An intramural course of a coronary artery is associated with increased operative risk for the ASO and thus is important to identify preoperatively. An intramural coronary artery can be identified as a vessel originating from the opposite sinus of Valsalva in close proximity to the commissure that faces the pulmonary valve with a parallel proximal course within the aortic wall. The origin of most intramural coronary arteries is tangential to the aortic wall. From the parasternal short‐axis view, the intramural coronary artery will often be seen coursing between the aorta and the pulmonary artery (Figure 25.20, Video 25.12). Every effort should be made to identify whether the origin of the intramural coronary artery is separate from the other coronary artery originating from the same aortic sinus of Valsalva (common orifice versus two separate orifices) although this is not always possible by echocardiography. Additional modalities such as computed tomography (CT) or magnetic resonance imaging (MRI) may be required if the coronary arteries are not well visualized or an intramural course is suspected [33]. Figure 25.19(a) Parasternal short‐axis view showing a single left coronary artery (LCA). All three coronary arteries arise from the left‐facing sinus from a single orifice with the right coronary artery (RCA) looping anterior to the aorta (Ao). (b) Parasternal short‐axis view showing a single RCA. In this case, the LCA loops anterior to the aorta arising from the right‐facing sinus. Cx, circumflex artery; LAD, left anterior descending artery; PA, pulmonary artery. Figure 25.20 Intramural course of the left coronary artery (LCA). Note the abnormal origin of the LCA from the right posterior sinus with a proximal intramural course between the great arteries (small arrows). Ao, aorta; PA, pulmonary artery; RCA, right coronary artery The presence of a PDA is important for mixing of the systemic and pulmonary circulations. In TGA, the orientation of the PDA is parallel to the long axis of the aortic arch and main pulmonary artery, allowing for imaging of the three vessels in one plane (Figure 25.21). The PDA is best seen from the suprasternal sagittal view, which demonstrates its aortic and pulmonary ends. Color and spectral Doppler demonstrate the direction of flow across the PDA. In most newborns with TGA, flow is from the main pulmonary artery to the descending aorta during systole and from the aorta to the main pulmonary artery during diastole (Video 25.13). In some patients, pulmonary vascular resistance is increased due to abnormal development of the pulmonary vascular bed. In that circumstance, flow through the PDA is predominantly right to left, which decreases effective pulmonary blood flow and results in severe cyanosis. Severe pulmonary hypertension can persist after the ASO and is associated with poor prognosis. Prenatal diagnosis of TGA can be made during the second trimester of pregnancy. In some cases, it can be detected with prenatal screening as early as 13–14 weeks’ gestation. Multiple studies have demonstrated that a screening ultrasound based solely on the four‐chamber view is inadequate for detection of anomalies of the great arteries, including TGA [34–37]. In fact, the four‐chamber view may appear normal in a fetus with TGA unless there is a large VSD. More cephalad views that include the outflow tracts will readily identify the parallel orientation of the great vessels with origin of the main pulmonary artery from the LV and origin of the aorta from the anterior and rightwards aspect of the RV (Figure 25.22, Video 25.14). Associated VSDs can also be demonstrated but small defects may be missed, particularly if located in the muscular septum. With anterior malalignment‐type VSD, the arch should be carefully assessed for coarctation or interruption. With posterior malalignment‐type VSD, the main pulmonary artery and branch pulmonary arteries may be small. Significant atrioventricular or semilunar valve regurgitation is rare. Figure 25.21 Suprasternal sagittal view in color compare mode showing the parallel course of the patent ductus arteriosus (PDA), the aortic arch (Ao), and main pulmonary artery (PA), visualized in one plane. The color Doppler demonstrates a left‐to‐right shunt (from the aorta to the main PA) during diastole. Figure 25.22 Fetal diagnosis of transposition of the great arteries. (a) At a gestational age of 22 weeks the outflow tracts must be visualized to make the diagnosis. The parallel course of the great vessels can be appreciated in this image. (b) The parallel vessels are also seen with color Doppler. (c) Fetus at 24 weeks’ gestation with TGA and outlet ventricular septal defect (VSD). Note the aortic and pulmonary valves are in fibrous continuity. Ao, aorta; LV, left ventricle; PA, pulmonary artery; RV, right ventricle. Evaluation of the atrial septum is important for identification of a restrictive patent foramen ovale or intact atrial septum, conditions that may result in severe cyanosis immediately after birth. The presence of a hypermobile septum on prenatal assessment may help to predict the need for an urgent balloon atrial septostomy postnatally, although the diagnostic accuracy of individual markers (including redundant atrial septum and bidirectional PDA flow) is quite limited [38]. The data on the effects of prenatal diagnosis of TGA on postnatal morbidity and mortality rates are conflicting. Some studies suggest that prenatal diagnosis is associated with improved postnatal outcomes [39,40], whereas other studies have found no apparent benefit [41,42]. Figure 25.23 Echocardiographic guidance of a balloon atrial septostomy. Subxiphoid views of the atrial septum are used to monitor the procedure. The position of the balloon in the left atrium can be checked prior to pulling the balloon back into the right atrium. (a) Subxiphoid left anterior oblique view showing the balloon in the left atrium up against the atrial septum. (b) There is now an atrial communication with left‐to‐right shunting after balloon septostomy. When balloon atrial septostomy is required, transthoracic echocardiography can guide the procedure and instantly evaluate the results. The subxiphoid long‐axis (frontal) view is generally the best to monitor the entire procedure. The position of the balloon before pulling it across the atrial septum should be assessed to assure it is free from the mitral valve, the left atrial appendage, and the pulmonary veins (Figure 25.23, Video 25.15). The pullback through the atrial septum can be imaged although the heart may shift momentarily when the catheter is pulled. After septostomy, subxiphoid imaging evaluates the size of the new atrial communication to determine whether the maneuver needs to be repeated and, importantly, whether a pericardial effusion has developed. Transesophageal echocardiography (TEE) is performed during surgery for TGA to evaluate the repair before and immediately after weaning from cardiopulmonary bypass (Figure 25.24). Epicardial echocardiography is an alternative approach preferred by some surgeons particularly to assess the branch pulmonary arteries and the coronary arteries. Here we summarize specific issues the echocardiographer needs to address for surgical procedures performed for TGA. The ASO, atrial switch operation, and Rastelli operation are discussed.
CHAPTER 25
Transposition of the Great Arteries
Definition
Incidence
Etiology
Morphology and classification
Developmental considerations
Anatomy
TGA with intact ventricular septum
TGA with ventricular septal defect
Coronary artery anatomy
Associated defects
Pathophysiology
Imaging
Imaging of TGA with intact ventricular septum
Subxiphoid views
Apical views
Parasternal views
Imaging of TGA with ventricular septal defect
Imaging of coronary arteries
Imaging of the patent ductus arteriosus
Prenatal assessment
Balloon atrial septostomy
Intraoperative assessment
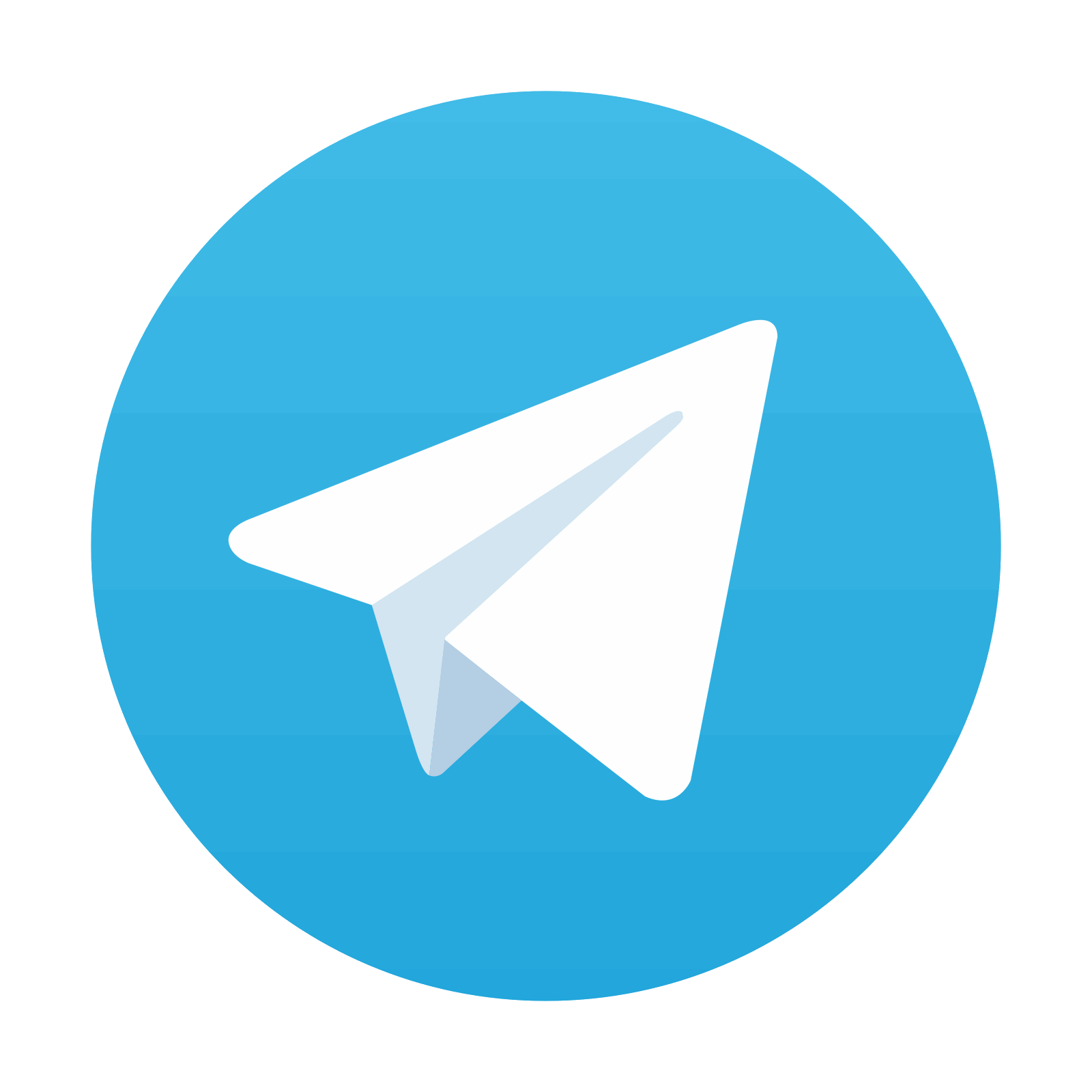
Stay updated, free articles. Join our Telegram channel

Full access? Get Clinical Tree
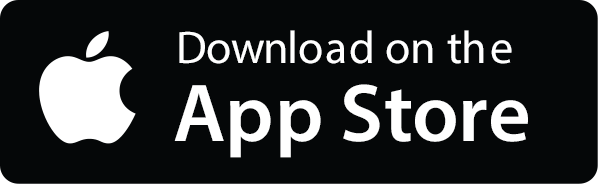
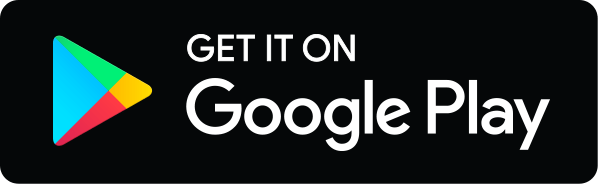