Anne‐Sophie Le Guern and Javier Pizarro‐Cerdá Yersinia was named after Alexandre Yersin, who first described the plague bacillus in Hong Kong in 1894. Considered as Pasteurella for many years, the genus Yersinia was proposed in 1944 but was established only in 1974 and classified within the Enterobacteriaceae family (Bottone et al. 2015). Since 2016, the genus Yersinia has been reclassified into the Yersiniaceae family within the Enterobacterales order (Adeolu et al. 2016). Because of its importance as the agent of human plague, and potentially also as an agent of biological warfare, there is a considerable amount known about the pathogenesis of Yersinia pestis and the related highly pathogenic Yersinia. Yersinia are facultative anaerobes, oxidase‐negative, and Gram‐negative rods or coccobacilli that ferment glucose. They grow slowly and form single colonies after growth for 48 hours at 25–30°C. They are psychotropic bacteria and may grow at 4°C. Phenotypic characteristics for species identification are often temperature dependent and usually more expressed by cultures incubated at 25–30°C (Bottone et al. 2015). Yersinia are widely distributed in nature and some species are adapted to specific animal hosts and humans. Five species are well‐known pathogens for humans and/or animals: Y. pestis, Y. pseudotuberculosis, Y. enterocolitica, Y. ruckeri and Y. entomophaga. Y. pestis is the causative agent of plague, a zoonotic disease usually transmitted by flea bite from rodents to rodents or accidentally from rodents to humans, resulting in a bubonic plague. A secondary pneumonic form may occur in infected animals or humans, and infected droplets may be transmitted by aerosolization to other animal or human, resulting in an often‐fatal primary pneumonia. The septicemic form may occur when the bacteria are directly inoculated in a blood vessel. Y. pseudotuberculosis and Y. enterocolitica are enteropathogens, responsible for enteric yersiniosis in humans and in a large variety of mammals and birds, causing syndromes ranging to mild diarrhea to systemic infection. Transmission generally occurs after ingestion of water or food contaminated by infected fecal matter. Y. ruckeri is a fish pathogen, the causal agent of the enteric redmouth disease in rainbow trout and other cultured salmonids. Bacteria enter the fish by infecting the secondary gill lamellae and spread to blood system. Y. entomophaga is an insect pathogen that kills larvae of coleoptera and is being studied for biological control of insect pests. Figure 9.1 Schematic representation of the basic pathogenesis of Yersinia infections. (Upper left) Yersinia pestis: fleas are infected upon a blood meal on contaminated rodents, and transmit Y. pestis to new mammalian hosts (including humans) by inoculating bacteria in the skin. Y. pestis migrates to draining lymph nodes, which swell (bubos) upon bacterial proliferation and infiltration of immune cells (bubonic plague). Bacteria may disseminate via the blood stream to organs such as the spleen and the liver leading to septicemia, and to the lungs leading to secondary pneumonic plague. Aerosols generated during coughing by infected mammalian hosts may lead to direct transmission of Y. pestis to new hosts, leading to the development of primary pneumonic plague. (Upper right) Yersinia pseudotuberculosis and Yersinia enterocolitica: bacteria contaminating food products are ingested and colonize the intestine leading to enteritis, then cross the intestinal barrier and migrate to the mesenteric lymph nodes provoking a mesenteric adenitis. Bacteria may disseminate via the blood stream resulting in granulomatous yersiniosis or in septicemia. (Lower left) Yersinia ruckeri: bacteria contaminating the water enter fishes generally through the gills and provoke septicemia. (Lower right) Yersinia entomophaga: bacteria are ingested by insect larvae, cross the intestinal barrier and induce septicemia. Source: Created with http://BioRender.com. A summary of the essential features of disease caused by these organisms is given in Figure 9.1. The non‐pathogenic species are Y. aldovae, Y. aleksiciae, Y. alsatica, Y. artesiana, Y. bercovieri, Y. canariae, Y. frederiksenii, Y. hibernica, Y. intermedia, Y. kristensenii, Y. massiliensis, Y. mollaretii, Y. nurmii, Y. occitanica, Y. pekkanenii, Y. proxima, Y. rohdei, Y. thracica and Y. vastinensis (Le Guern et al. 2020). A recent phylogenetic analysis of representative Yersinia strains, based on 500 core genes, led to update the taxonomy of the Yersinia genus with a total of 26 species (Figure 9.2; Bottone et al. 2015; Savin et al. 2019). Four genetically close species comprised the Y. pseudotuberculosis complex (Savin et al. 2014; Adeolu et al. 2016): Y. similis, Y. wautersii, Y. pseudotuberculosis and Y. pestis. Y. pseudotuberculosis, and Y. pestis are well‐known pathogens of humans and animals. Y. pseudotuberculosis is divided into at least 32 lineages based on phylogenetic analysis, into 6 serotypes based on the immunoreactivity of its O antigens, and into 21 genoserotypes based on the presence of specific O‐antigen genes (Bogdanovich et al. 2003; Bottone et al. 2015). Strains that are considered highly pathogenic host the Yersinia virulence plasmid (pYV) and harbor a high pathogenic island (HPI; see below). Figure 9.2 Phylogeny of the 26 species within the genus Yersinia. Maximum‐likelihood phylogenetic tree of the genus Yersinia based on 500 concatenated multiple sequence alignments from 242 strains. The most recently described species are highlighted in color. Yersinia pestis (represented by the strain CO92) clusters within the genus Yersinia pseudotuberculosis. Y. pestis is a particular lineage of Y. pseudotuberculosis that diverged around 20 000 years ago from the enteropathogen Y. pseudotuberculosis through events of gain of genes as well as gene and genome reduction. The denomination “Y. pestis” is still used because of the drastic differences in severity of the disease and mode of transmission, together with the presence of distinctive virulence factors. Y. pestis strains may be classified into four biovars (antiqua, medievalis, microtus, orientalis) based on glycerol acidification and nitrate reduction. Yersinia wautersii can be considered as a pathogen despite the absence of the pYV plasmid (which was probably lost during laboratory subcultures of the type strain), since these bacteria are pyrazinamidase negative, a phenotypic characteristic of Yersinia strains pathogenic for humans (Savin et al. 2014). Y. similis is found in the environment and can be isolated from animals but is not associated with clinical disease. Y. enterocolitica is divided into 13 lineages based on phylogenetic analysis, into 6 biogroups (1A, 1B, and 2–5) based on biochemical characteristics and into approximatively 60 serogroups based on the immunoreactivity of its O antigens (Bottone 1999). Strains belonging to biotype (BT) 1A are considered non‐pathogenic since they lack the pYV plasmid. BT1A strains, which may belong to various serotypes, are abundant in the environment, including soil and water. Biotypes 1B, 2, 3, 4 and 5 contain pathogenic strains harboring the pYV plasmid. Pathogenic biotypes are associated with certain serotypes: the most common bioserogroups in humans and animals are BT4/O:3, BT2/O:9, BT2/O:5,27 and BT3/O:3. BT1B is often associated with serogroups O:8, O:16, or O:21, and is considered highly pathogenic since the strains possess the HPI (see Section below). Two Yersinia species are specific animal pathogens. Y. ruckeri is a fish pathogen divided into eight serotypes based on the immunoreactivity of its O antigen and two biotypes based on motility and lipase activity. Most fish outbreaks are caused by strains belonging to serotype O:1 biotype 1. Y. entomophaga is an insect pathogen, which is currently studied for its use as biological pesticide. Aspects of the evolution of pathogenic of Yersinia are given in a description of the individual pathogenic species. Sources of infection, epidemiology, and types of disease are discussed with the individual pathogenic species. The highly pathogenic Yersinia possess two critical virulence factors. A major molecular virulence factor of Y. enterocolitica (all biotypes except BT1A), Y. pseudotuberculosis and Y. pestis is a type III secretion system (T3SS) encoded in the pYV plasmid (known also as pCD1 in Y. pestis). This system is composed of an injectisome, a nanomachine that spans the bacterial cell wall and allows the injection of bacterial effectors (known as Yersinia outer proteins or Yops) directly into the cytoplasm of host cells. Expression of the system is activated at 37°C and depends on RNA‐based thermoregulation of the virulence regulator LcrF, since its mRNA displays a hairpin structure that is occluded at environmental temperatures and is only accessible to ribosomes at mammal host temperatures (Böhme et al. 2012). Upon bacterial contact with host cells, a decrease in calcium triggers translocation of Yops into host cells (Figure 9.3). Yops have been shown to interfere with several host signaling cascades, inhibiting in particular phagocytosis and activation of immune responses, and promoting cell death. Phagocytosis inhibition is promoted by inactivation or downregulation of host cell Rho GTPases, which are master regulators of actin cytoskeleton polymerization. For example, YopT is a cysteine protease that inactivates RhoGTPases such as RhoA, Cdc42 and Rac by removing post‐translational modifications that are required for the membrane attachment and function of these host‐cell enzymes (Shao et al. 2003). YopE mimics GTPase activating proteins (GAPs), increasing the hydrolytic activity of RhoGTPases which leads to their inactivation upon hydrolysis of GTP to GDP (Von Pawel‐Rammingen et al. 2000). YopO (also known as YpkA) displays a guanine nucleotide dissociation inhibitor domain which allows to sequester inactive GDP‐bound RhoGTPases (Prehna et al. 2006). Interestingly, YopO can also use actin as a bait and through a serine/threonine kinase domain it can phosphorylate/inactivate multiple actin binding proteins (Lee et al. 2015). Inactivation of immune responses is achieved by molecules such as YopH, a phosphatase that dephosphorylates critical proteins required for T‐cell receptor signaling (Gerke et al. 2005), or YopJ (named YopP in Y. enterocolitica), an acetyltransferase that blocks mitogen‐activated protein kinase and nuclear factor‐κB (NF‐κB) signaling by inhibit their phosphorylation (Orth et al. 2000). Host cell death is promoted by YopM, which displays E3 ubiquitin ligase activity targeting NLRP3. YopK, a protein with no sequence homology to any protein outside pathogenic Yersinia species, functions not by interacting with host proteins but by preventing recognition of the T3SS by the host cell by associating/masking the YopB/YopD proteins, which constitute tip of the T3SS needle (Brodsky et al. 2010). Figure 9.3 Host–pathogen interactions at the cell surface. Adhesins Invasin and YadA in Yersinia pseudotuberculosis and Yersinia enterocolitica, and YadB and YadC in Yersinia pestis bind tightly to the host cell receptors β1‐integrins ensuring a close association between the bacterium and host cells. T3SS forms a pore across the host cell membrane resulting in the delivery of Yersinia outer proteins (Yops) into the host cell. The Yop effector proteins induce the inhibition of phagocytosis and the down‐regulation of immune system. Source: Created with http://BioRender.com. It is important to note that LcrV, which is also localized at the tip of the injectisome and is essential for the assembly of a functional translocation pore formed by YopB and YopD, is highly immunogenic and has been extensively used as a target for vaccination against pathogenic Yersinia, and particularly Y. pestis. The most highly pathogenic Yersinia are characterized by the presence of a mobile element, the HPI, which encodes the yersiniabactin‐based iron acquisition system (Carniel 2001). Genes responsible for the biosynthesis, transport and regulation of yersiniabactin (Ybt) are repressed by the iron‐loaded ferric uptake regulator (Fur) and are activated by the AraC‐type YbtA transcriptional activator. Inactivation of the Ybt biosynthetic and/or transport genes results in significant virulence attenuation of highly pathogenic Yersinia (Rakin et al. 2012). Interestingly, some pathogenic Y. pseudotuberculosis strains have been shown to be devoid of the HPI, suggesting the presence of other iron‐sequestering systems. Indeed, the pseudochelin (also known as Yersinia non‐ribosomal peptide, Ynp) and the yersiniachelin (Ych, encoded by the ysu gene cluster) have been subsequently identified in Y. pestis and Y. pseudotuberculosis, but not in Y. enterocolitica. Functional studies are currently lacking to fully understand the contribution of these alternative iron‐sequestering system to the pathogenicity of Y. pestis and Y. pseudotuberculosis. Y. pestis is the agent of plague, a disease of enormous importance in human history as the cause of devastating pandemics; the “Black Death” in Europe killed one third of the population between 1346 and 1353. It is a zoonotic infection acquired by people mostly through bites by fleas but also by direct contact with infected animals including their carcasses and meat. Y. pestis evolved less than 20 000 years ago from its ancestor Y. pseudotuberculosis. Both gene gain and gene inactivation events played an important role in the evolution from the mildly pathogenic, foodborne ancestor Y. pseudotuberculosis toward the highly pathogenic, vector‐borne, Y. pestis. Besides the virulence plasmid pYV/pCD1, present also in Y. pseudotuberculosis and Y. enterocolitica, Y. pestis harbors two additional plasmids, pFra and pPla (also known as pMT1 and pPCP1, respectively), which play important roles in adaptation to the flea vectors and in pathogenesis in mammalian hosts. A key step in the adaptation of Y. pestis to its flea vector was the silencing of urease activity through mutation of the ureD gene, which reduces significantly the bacterial oral toxicity (Chouikha and Hinnebusch 2014). Another important adaptive step was the formation of a bacterial biofilm that blocks the flea’s proventriculus (the valve connecting the esophagus to the midgut), inhibiting the arrival of the blood meal to the midgut. Starving infected fleas bite their mammalian hosts with high frequency in their attempt to feed, and Y. pestis released from the biofilm and regurgitated at the feeding site can transmit plague. The biofilm is enveloped in a beta‐1,6‐N‐acetyl‐D‐glucosamine polymer and its production by the HmsHFRS complex is favored through inactivation in Y. pestis of the rcsA gene, whose product RcsA forms a dimer with RcsB that transcriptionally represses the hmsHFRS operon (Sun et al. 2014). Cyclic di‐GMP activates biofilm formation in Y. pestis, and inactivation of the genes rtn and y3389, which encode two phosphodiesterases that degrade cyclic di‐GMP, also contributes to increased biofilm formation in the flea (Sun et al. 2014). Two additional genes which participate to Y. pestis resistance to toxic compounds encountered in the flea gut, and which also contribute directly or indirectly to biofilm formation, are the ancestral gene rpiA, which encodes for a ribose‐5 phosphate isomerase, and the recently acquired gene ymt (present in the pFra plasmid), which encodes a phospholipase (Dewitte et al. 2020). Y. pestis is endemically present in diverse sylvatic and periurban mammal populations in the Americas, in Africa and in Asia. Rodents (279 species) are the main reservoirs, but carnivores (31 species), and lagomorphs (14 species) have been also reported as reservoirs (Mahmoudi et al. 2020). In North America (mainly the United States), for example, reservoir species include prairie dogs (genus Cynomys), California voles (Microtus californicus) and deer mice (genus Peromycus; Mahmoudi et al. 2020). In South America (mainly Brazil and Peru), mammals found to harbor Y. pestis include hairy‐tailed bolo mice (Necromys lasiurus), common yellow‐toothed cavy (genus Galea), and also the lagomorph tapeti (Sylvilagus brasiliensis) (Bonvicino). In Africa (mainly Democratic Republic of Congo and Madagascar), black rats (Rattus rattus), brown rats (Rattus norvegicus), Southern African vlei rats (Otomys irroratus), and the house shrew (Suncus murinus) have been reported as reservoirs. In Asia (China, Mongolia, former Soviet Union, Iran), reservoirs include many different species, including meriones and gerbils (genus Meriones), great gerbils (Rhombomys opimus), marmots (genus Marmotta), and ground squirrels (genus Spermophilus) (Mahmoudi et al. 2020). It is proposed that in animal reservoirs, Y. pestis can silently persist during long periods in enzootic cycles within plague‐resistant wild rodents, and that transmission to susceptible hosts (such as periurban rodents) leads to epizootic cycles, in which high host mortality is observed. The organism can also survive in soil, such as in the burrows of rodents, allowing contamination of animals that circulate in these environments. Plague can be transmitted through the bite of fleas infected with Y. pestis (Figure 9.3). Fleas can be infected upon feeding on septicemic mammalian hosts. Fleas species are often specific to individual mammalian hosts species and, since the mammalian reservoirs of Y. pestis are diverse as described above, many flea species can be potentially involved in plague transmission. Y. pestis infection of its flea vector has been particularly well studied in the species Oropsylla montana (associated with ground squirrels in North America), the Oriental rat flea Xenopsylla cheopsis, and the cat flea Ctenocephalides felis. Y. pseudotuberculosis, the ancestor of Y. pestis, is orally toxic to fleas due to the expression of urease, which leads to insect diarrhea, immobility and up to 40% mortality of infected insects (Erickson et al. 2007). In the case of pulmonary plague, Y. pestis can be transmitted via aerosols produced upon coughing. Airborne plague transmission from animals to humans has been reported (Doll et al. 1994). There are three presentations of plague in animals and in humans: bubonic, the most common form, associated with lymph‐node swelling with abscessation, called buboes, and fatal febrile illness; pneumonic plague, associated with fatal pneumonic disease; and septicemic plague, characterized by septic shock and death. Plague primarily affects wild and domestic rodents, although felines are also very susceptible. Disease in rodents can vary from subacute and mild to acute, severe, and fatal. In acute disease, rodents die within a few days with hemorrhagic buboes and splenomegaly, whereas in subacute disease, rodents develop necrotic and suppurative lymph nodes and similar lesions in the liver, lung, spleen, and other organs; some animals may recover. Secondary pneumonic plague characterized by severe hemorrhagic pneumonia develops in a small proportion of subacutely infected animals. Cats (and other felines) develop typical bubonic plague, with abscesses and lymphadenopathy, often of the cervical and submandibular lymph nodes, spread of infection to and development of lesions in the liver and spleen, and secondary suppurative pneumonia. Cats may also develop septicemic illness without buboes. Disease occurs uncommonly in dogs and has been rarely reported in species such as mule deer and antelope, but does not occur in cattle, horses, sheep, and pigs. Infection in humans usually follows a flea bite, with sudden onset of severe systemic illness and development of a bubo in the local draining lymph node, and subsequent spread to other lymph nodes in the body; patients go on to develop septicemia and multiple organ failure (including lung collapse during pneumonic plague). The name “black death” comes from the gangrene associated with the coagulopathy characteristic of septic or endotoxic shock. The most common form of plague in humans is bubonic plague, which arises upon intradermal inoculation of Y. pestis by fleas. Bacteria disseminate to locally draining lymph nodes, which swell upon bacterial replication and migration of immune cells and are named buboes. Y. pestis then reach the bloodstream, resulting in systemic spread and host death caused by septicemia (see below). Early stages of bubonic plague are characterized by Y. pestis replication in the dermis and by the recruitment of neutrophils which play an important role in controlling bacterial proliferation (Shannon et al. 2013; Gonzalez et al. 2015). Macrophages and dendritic cells also migrate toward flea bite sites containing Y. pestis, but these cell populations interact less actively with bacteria compared to neutrophils (Shannon et al. 2015). The plasmid pYV, which encodes the T3SS, plays an important role in Y. pestis escape from neutrophil control (Shannon et al. 2015). Bacteria can be found in local draining lymph nodes less than an hour after intradermal inoculation (Gonzalez et al. 2015; Shannon et al. 2015), indicating that Y. pestis can reach lymph nodes with the flow of the lymph, and do not need to be intracellularly associated to immune cells to reach the local draining lymph nodes (Gonzalez et al. 2015). In a rat model of bubonic plague, Y. pestis first proliferates in the lymph node marginal sinus within 24 hours of infection, before expanding toward the lymph node cortex by 36 hours post‐infection. Late in the infection, bacteria completely colonize the whole organ and are mixed with abundant cellular debris and necrotic neutrophils (Sebbane et al. 2005). The T3SS plays a critical role in inhibiting bacterial phagocytosis, in blocking host immune responses and in promoting death of immune cells. Extracellularly proliferating Y. pestis are exposed to nitric oxide‐derived reactive nitrogen species as well as to iron limitation, and bacteria activate genes involved in nitric oxide detoxification, and iron or heme transport systems (Sebbane et al. 2006b). A major bacterial factor required for bubonic plague is the broad‐spectrum, outer membrane protease Pla, encoded in the Y. pestis‐specific plasmid pPla/pPCP1. Pla (plasminogen activator) was initially identified as an enzyme responsible for fibrinolytic and plasma‐coagulase activities in Y. pestis. It had been proposed that the Pla proteolytic activity was required for digestion of the fibrin clot formed during the flea bite at the inoculation site, as well as for degradation of the surrounding connective tissue. However, it was subsequently found the pla‐negative Y. pestis is able to reach lymph nodes, but cannot induce bubonic plague (Sebbane et al. 2006a). It had been also hypothesized that the broad protease activity of Pla might have a role in infiltration and destruction of the draining lymph node, but it was recently shown that this is not the case and that Pla instead plays a role in bacterial survival and replication in this organ (Guinet et al. 2015). Despite an important number of host protein substrates which have been identified in vitro for Pla, very few have been validated in vivo. Interestingly, Pla cleaves the Y. pestis autotransporter YapE, and a yapE‐negative Y. pestis displays a similar dissemination defect to a pla‐negative mutant after bacterial subcutaneous inoculation (Lawrenz et al. 2009). Therefore, it has been postulated that Y. pestis may use Pla to cleave its own rather than host proteins to produce bubonic plague (Sebbane et al. 2020). In the mammalian host, Y. pestis also expresses a pseudocapsule, composed of the antigen fraction 1 (F1) encoded by the caf1 gene located in the pFra/pMT1 plasmid, which inhibits phagocytosis (Du et al. 2002). A role for the F1 pseudocapsule has been reported in bubonic plague (Sha et al. 2011), but the importance of F1 in the pathogenesis of Y. pestis in the lymph node seems to depend on the genetic background of the infected animal models (Weening et al. 2011). Y. pestis can efficiently proliferate in the lungs of mammalian hosts, leading to the form of the disease known as pneumonic plague, which is almost always fatal. Bacteria can reach the lungs via the bloodstream upon dissemination from primary infected lymph nodes, giving rise to secondary pneumonic plague. Primary pneumonic plague can occur upon inhalation of aerosols produced by a person (or animal) infected with pneumonic plague. In humans, acute pneumonia, intra‐alveolar hemorrhage and edema follow Y. pestis replication in the alveoli, leading to collapse of pulmonary functions and death (Smith 1959
9
Yersinia
Introduction
Characteristics of the Organism
Pathogenic Species
Taxonomy
Major Virulence Factors of the Highly Pathogenic Yersinia
Type 3 Secretion System
Iron‐Sequestering Systems
Yersiniapestis
Source of Infection: Evolution, Ecology and Epidemiology
Types of Disease and Pathogenesis
Bubonic Plague
Pneumonic Plague
Stay updated, free articles. Join our Telegram channel

Full access? Get Clinical Tree
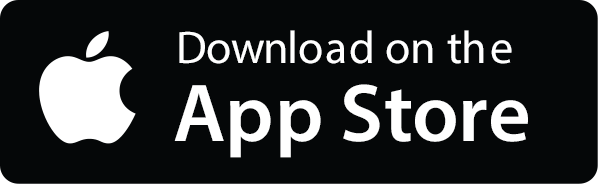
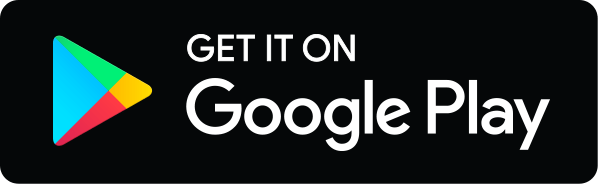