Anders M. Bojesen Virginia Aragon and Pat J. Blackall In addition to Pasteurella (Chapter 10), Mannheimia (Chapter 11), and Actinobacillus (Chapter 12) there are several other genera belonging to the family Pasteurellaceae that cause important animal diseases. These “other Pasteurellaceae” (Avibacterium, Bibersteinia, Gallibacterium, Glaesserella, and Histophilus) described in this chapter are generally less well studied so there are more gaps in our understanding of their mechanisms of pathogenesis. Nevertheless, these genera continue to cause economically significant diseases in many countries and are worthy of study. The genus Avibacterium consists of bacteria previously regarded as either Haemophilus or Pasteurella and includes five species: Avibacterium avium, Avibacterium endocarditidis, Avibacterium gallinarum, Avibacterium paragallinarum and Avibacterium volantium (Blackall and Soriano‐Vargas 2020). Members of the genus Avibacterium are Gram‐negative non‐motile bacteria. Most isolates of A. avium, A. paragallinarum and A. volantium require nicotinamide adenine dinucleotide (NAD) for in vitro growth. A. paragallinarum isolates typically require an atmosphere of 5–10% carbon dioxide. Members of the genus are grown at 37–38°C. Three species have been linked with disease: A. endocarditidis, A. paragallinarum and A. gallinarum (Blackall and Soriano‐Vargas 2020). The genus is associated with birds, with carrier birds being the main reservoir of A. paragallinarum. A. paragallinarum causes infectious coryza of poultry, an acute inflammation of the upper respiratory tract involving the nasal passage and sinuses with a serous to mucoid nasal discharge, facial edema, and conjunctivitis (Blackall and Soriano‐Vargas 2020). Infectious coryza causes a marked drop in egg production (10–40%) (Blackall and Soriano‐Vargas 2020). A. gallinarum has been associated acute respiratory disease, coughing and sneezing, with some outbreaks involving periorbital swelling and keratoconjunctivitis, and swollen wattles. A. endocarditidis causes valvular endocarditis in broiler breeders. The virulence factors that have been identified as playing a role in infectious coryza include: (i) capsule, based on evidence that isogenic acapsular mutants are less virulent in chickens than the parent strain (Tu et al. 2015); (ii) a trimeric autotransporter that has hemagglutination activity (termed HMTp210) and which when not expressed results in reduced virulence (Wang et al. 2014); (iii) lipopolysaccharide (LPS; Chiang et al. 2013); (iv) fimbrial protein FlfA (mutants lacking this protein are less virulent than the parent strains; Liu et al. 2016a); (v) a bivalent serine‐protease repeats in the structural toxin (RTX) termed Avx, which has the classic RTX operon structure (activator gene avxC, structural gene avxA, and the genes for a type 1 secretion system avxBD) and is cytotoxic for an avian macrophage‐like cell line (HD11; Küng and Frey 2013); (vi) an apparent cytolethal distending toxin (CDT), which causes typical CDT activity (cell‐cycle arrest and apoptosis) when HeLa cells or chick embryo fibroblast cells are exposed to filter‐sterilized lysates of A. paragallinarum (Chen et al. 2014); (vii) a metalloprotease which can partially degrade chicken immunoglobulin (Ig) G (Rivero‐García et al. 2005); and (viii) hemocin; the locus containing the hemocin structural gene also has an immunity gene (Terry et al. 2003; Hsu et al. 2007). Phase‐variable expression of ChoP, which controls expression of phosphorylcholine on lipopolysaccharide; Chiang et al. 2013 and FlfA have been reported (Liu et al. 2016a). Epigenetic regulation of virulence by random switching of DNA methylation patterns appears to be a feature in A. paragallinarum (Atack et al. 2020). Figure 13.1 Mechanisms of pathogenesis of Avibacterium paragallinarum, illustrating the four key stages of the disease process: colonization, multiplication, evasion of host defenses, and host tissue damage. Some virulence factors play a role in multiple areas; for example, lipopolysaccharide (LPS) may play a role in colonization by aiding attachment, and LPS that is decorated with phosphorylcholine (ChoP) has decreased susceptibility to the chicken antimicrobial peptide fowlicidin‐1. The photographs show the typical clinical signs of a mild case of infectious coryza with mildly swollen sinus (bottom) and more advanced disease with clear sinus swelling (top). AvxA, bivalent serine‐protease repeats in the structural toxin; CDT, cytolethal distending toxin; FlfA, fimbrial protein; HMTp210, trimeric autotransporter; IgA, immunoglobulin A; TbpA, transferrin‐binding protein A; TbpB, transferrin binding protein B. Source: Photographs provided by Dr. Alejandro García Flores, Laboratorios Avilab, Mexico. The upper respiratory tract is the primary site of colonization of A. paragallinarum with HMTp210 activity (Wang et al. 2014) and FlfA (Liu et al. 2016a) playing a role in this process (Figure 13.1). The ability to multiply and survive appears to be aided by two outer‐membrane protein (OMP) in A. paragallinarum that are specific receptors for chicken and turkey ovotransferrin (Ogunnariwo and Schryvers 1992). The capsule of A. paragallinarum appears to play a role in evasion of host defenses, as acapsular mutants are less virulent. The addition of ChoP to LPS decreases the susceptibility of A. paragallinarum to fowlicidin‐1 (Chiang et al. 2013). The RTX toxin produced by A. paragallinarum (AvxA) is cytotoxic for an avian macrophage‐like cell line (Küng and Frey 2013). The ability of a metalloprotease to partially degrade chicken IgG (Rivero‐García et al. 2005) may also contribute to an evasion of host defenses. Comparison of acapsular and capsulated variants has shown that capsule causes cilia and microvilli loss, infiltration of leucocytes, and deposition of mucopurulent substances. A CDT may also contribute to host‐cell damage (Chen et al. 2014). In the individual bird and in the absence of a concurrent infection, the clinical signs of coryza typically resolve within two to three weeks (Blackall and Soriano‐Vargas 2020). Pullets that have suffered an infectious coryza outbreak during their growing stage are typically protected against egg drops in later coryza challenges (Blackall and Soriano‐Vargas 2020). Artificial challenge produces resistance to reinfection as early as two weeks post‐exposure. The resistance associated with infection is effective across the three recognized Page serovars unlike the protection provided with killed vaccines (see Section 13.2.5). Inactivated whole cell vaccines, if prepared adequately, are typically able to provide effective protection against infectious coryza (Blackall and Soriano‐Vargas 2020). Unlike natural infection, vaccination with killed coryza vaccines is Page serovar specific (e.g. chickens vaccinated with Page serovar A are protected against challenge from Page serovar A but not Page serovars B or C). More recent studies have challenged this picture and have suggested that there may only be partial protection within Page serovars. Various sulfonamides and antibiotics, particularly tetracycline, have been used to reduce the severity and course of an infectious coryza outbreak (Blackall and Soriano‐Vargas 2020). However, both relapses after treatment and the inability to eliminate the carrier state have long been recognized. As well, antibiotic resistance and the occurrence of plasmid‐associated multidrug resistance have been reported (Hsu et al. 2007). There is still a lack of understanding of the full range of virulence factors of A. paragallinarum. Perhaps, the most puzzling feature that has received little attention to date is the process by which an upper respiratory infection drives a marked, prolonged, egg drop. Another key issue is why the natural infection produces cross‐serovar protection, but a killed vaccine provides only serovar‐specific protection at best. The genus Bibersteinia consists of a single species, Bibersteinia trehalosi, an organism previously called Pasteurella haemolytica biovar T (for trehalose fermenting) and Pasteurella trehalosi Blackall et al. 2007; see also Chapter 11. Members of this genus are Gram‐negative, non‐motile, rod‐shaped, or pleomorphic cells. Bibersteinia trehalosi is a facultative anaerobe and is typically grown at 37°C. Hemolysis of sheep red blood cells and a positive cyclic adenosine monophosphate (cAMP) reaction are common. Variable reactions have been reported for the catalase and oxidase tests. B. trehalosi is present in the upper respiratory tract and/or tonsils of ruminants including sheep, cattle, goats, and wild ruminants: bison (Bison bison), chamois (Rupicapra rupicapra) and bighorn sheep (Ovis canadensis). The occurrence of B. trehalosi in domestic livestock and wildlife species has raised concerns about the potential movement of the organism between these species. A study of domestic and wild ruminants in the French Alps found no evidence of such movement (Villard et al. 2006). However, in‐contact movement of both B. trehalosi and Mannheimia haemolytica from domestic sheep to bighorn sheep, resulting in a severe pneumonia, has been reported (Onderka and Wishart 1988). B. trehalosi is an important pathogen of sheep, causing septicemia in lambs and pneumonia in adult sheep. In cattle, there is a view that the organism is not a primary respiratory pathogen of calves. However, B. trehalosi was the sole pathogen in 18 of 65 cattle with pneumonia (Collins 2011) and was reported as the causal agent of necrotizing pleuropneumonia and septicemia in 15 dairy calves (Brown et al. 2021). A controversial topic has been the role of B. trehalosi in the decline of bighorn sheep in North America. The fact that over 85% of B. trehalosi isolates from bighorn sheep lack the ability to produce a key virulence factor, a leukotoxin, caused the suggestion that the organism is simply part of normal microbiota. However, leukotoxin‐producing B. trehalosi do occur in bighorn sheep and have been linked with respiratory disease in both adults and young (Wood et al. 2017). The virulence factors that have been identified or suggested as having a role to play in the development of the various disease manifestations associated with B. trehalosi are as follows: (i) capsule, which may be involved with host‐cell attachment, although there is also the suggestion that the capsule serovar T3 may have a reduced virulence in sheep (Davies and Quirie 1996); (ii) collagenase activity, which has been demonstrated in a B. trehalosi capsule serovar T10 isolate (McNeil et al. 2003); (iii) fibrinogen‐binding proteins, which may contribute to the characteristic fibrinous pneumonia associated with B. trehalosi and may assist in adhesion and help block phagocytic activity (McNeil et al. 2002); (iv) leukotoxin (Lkt), an exotoxin that belongs to the family of RTX and which shares considerable homology with the Lkt of M. haemolytica (Murugananthan et al. 2018). The observation that disease in bighorn sheep is typically caused by Lkt producing B. trehalosi (Wood et al. 2017) and the experimental infection work showing that an Lkt positive B. trehalosi isolate caused severe pneumonia in all four bighorn sheep, while an Lkt negative isolate did not cause pneumonia (Dassanayake et al. 2013) support the role of Lkt; (v) neuraminidase, although the activity is typically lower than that of M. haemolytica (Straus et al. 1993); and (vi) superoxide dismutase, which protects aerobic bacteria from reactive oxygen species such as superoxide anion (O2–) and H2O2, has been detected in the periplasm of B. trehalosi capsule serovar T10 and presumably explains why exogenous superoxide does not reduce the viability of this strain (Rowe et al. 1997). There is a lack of knowledge on the regulation of virulence in B. trehalosi and while a range of disease conditions and hosts are associated with B. trehalosi, our best understanding occurs in the septicemic disease seen in lambs. The basic pathogenic process appears to involve colonization of the upper respiratory tract and tonsillar mucosal epithelia, entry into the blood stream from which the organism may cause septic shock in young lambs or reaches the lungs where the destruction of phagocytes by the Lkt allows uncontrolled proliferation of the organism and acute inflammation through the effect of LPS release of inflammatory mediators. Details of pathogenesis are not as well described as for M. haemolytica (Chapter 11) but the basis of pneumonia is likely very similar. Disease may be predisposed to by other pathogens, notably Mycoplasma ovipneumniae. B. trehalosi is a common inhabitant of the upper respiratory tract and tonsils of healthy ruminants. In sheep, a period of stress has been suggested to cause a corticosteroid‐dependent bacterial proliferation in the tonsillar region (Dyson et al. 1981) and ulceration of the alimentary tract (Suarez‐Guemes et al. 1985). These injured mucosal surfaces serve as portals of entry for B. trehalosi derived from the tonsils. After gaining access to the blood, the B. trehalosi cells gain access to the lungs and the hepatic sinusoids causing embolic lesions where further multiplication occurs and spread to other organs is possible (Dyson et al. 1981; Suarez‐Guemes et al. 1985). The Lkt toxin of B. trehalosi is cytolytic for all subsets of ruminant leukocytes (Subramaniam et al. 2014) and plays a key role in overcoming host defenses. The fibrinogen‐binding proteins produced by B. trehalosi may block phagocytosis (McNeil et al. 2002). Polymorphonuclear leukocytes (PMN) are the subset of leukocytes that are most susceptible to Lkt‐mediated lysis. The PMNs of bighorn sheep are three to seven times more susceptible to B. trehalosi Lkt‐induced cytolysis than domestic sheep (Silflow et al. 1993), possibly explaining the role of B. trehalosi in pneumonia in bighorn sheep. PMN lysis and degranulation has been suggested as the main cause of acute inflammation and lung damage in respiratory infections due to Lkt‐positive bacteria. The fibrinogen‐binding activity of B. trehalosi may initiate a clotting cascade and culminate in fibrin deposition (McNeil et al. 2002). Collagenase activity in B. trehalosi might be contributing to destruction of lung tissue allowing deeper colonization and enhanced inflammation (McNeil et al. 2003). In the septicemic disease seen in lambs, death is attributed to endotoxemia (Dyson et al. 1981; Suarez‐Guemes et al. 1985). As Lkt‐neutralizing antibodies protect against challenge from M. haemolytica, the same scenario has been assumed to apply for B. trehalosi. This is supported by the finding that a M. haemolytica toxoid (when used in a multivalent viral vaccine) provides significant protection against clinical signs, lesions, and mortality associated with a B. trehalosi challenge in cattle (Bowersock et al. 2014). The ability to produce neutralizing antibodies to the collagenase of B. trehalosi has been correlated with reduced level of pneumonic lung tissue in bighorn sheep (McNeil et al. 2003). In some regions, B. trehalosi‐based vaccines for sheep and goats are available, either as a stand‐alone vaccine or as part of clostridial disease vaccines. As noted above, in cattle, a five‐agent vaccine that contains a M. haemolytica toxoid provides significant protection against challenge with B. trehalosi. As stress can initiate disease, livestock management strategies that minimize stress from environment and nutrition changes are important. There is potential for movement of B. trehalosi from domestic livestock to wildlife ruminants so the mixing of these species should be avoided. Antimicrobial treatment is possible although in sheep the disease progresses so rapidly that animals are rarely seen in the early stage of the disease. Antimicrobial resistance genes linked to a plasmid have been reported in a calf isolate of B. trehalosi (Kehrenberg et al. 2006). Our understanding of the virulence factors of B. trehalosi has been hampered by early uncertainty in the identification of this species. In some reports, it was not clear if the study involved B. trehalosi as terms such as “P. haemolytica” and M. haemolytica were used. Now that rapid and specific assays have been developed to identify B. trehalosi this confusion should be at end, and it should be possible to, for example, clarify the role of Lkt‐positive B. trehalosi in diseases of cattle and bighorn sheep. The genus Gallibacterium is a member of the family Pasteurellaceae (Christensen et al. 2003) that is associated with a range of avian host species. One species, Gallibacterium anatis biovar haemolytica, is commonly associated lesions in the reproductive tract of farmed chickens around the world. The genus has four named species: G. anatis, Gallibacterium melopsittaci, Gallibacterium trehalosifermentans, and Gallibacterium salpingitidis, and three genomospecies (Bisgaard et al. 2009). G. anatis can be further subdivided into two phenotypically distinct biovars: biovar haemolytica and the non‐hemolytic biovar anatis. Colonies of Gallibacterium are 1–2 mm, grayish, smooth, semitransparent, slightly raised, and circular with an entire margin when incubated for 24 hours at 37°C on nutrient‐rich plates containing blood. G. anatis biovar haemolytica induces β‐hemolysis (1–2 mm) on agar plates with blood from calf, horse, swine, sheep, rabbit, or chicken. G. anatis is commonly isolated from healthy chickens but has also been reported from a wide range of both domestic and non‐domestic birds including turkeys, geese, ducks, pheasants, partridges, caged birds, and wild birds. In egg‐laying chickens it is among the most common causes of salpingitis and peritonitis. The significance of G. melopsittaci, G. trehalosifermentans, and G. salpingitidis in relation to disease is much less certain although all three species have been isolated from lesions in psittacine and web‐footed birds, respectively. In flocks of chickens colonized with G. anatis practically all individuals carry the bacterium in the upper respiratory tract and the lower reproductive tract. Only in premises observing very high levels of biosecurity are birds not colonized (Bojesen et al. 2003a). Horizontal transmission is the main mode of transmission within a flock; however, the initial establishment of infection appears less certain. Low frequency of vertical transfer between parent and offspring flocks followed by effective horizontal spreading within a flock has been proposed. The within‐flock genetic diversity is typically very low, corresponding to a presence of one to three genetically similar clonal lineages per epidemiological unit, which could indicate a very efficient within‐flock transmission following introduction (Bojesen et al. 2003b). In chickens, G. anatis frequently colonizes the upper respiratory tract and lower genital tract of healthy animals. In diseased chickens, it has been associated with a wide range of lesions including septicemia, pericarditis, hepatitis, oophoritis, follicle degeneration, enteritis, upper respiratory tract lesions, salpingitis, epididymitis, and peritonitis. Indeed, G. anatis is reported to be one of the most common bacterial species isolated from egg‐laying chickens with reproductive tract disorders (Mirle et al. 1991; Neubauer et al. 2009). In cockerels, G. anatis can colonize the testicle and epididymis and cause inflammation and lowered semen quality (Paudel et al. 2014a). One of the main characteristics of G. anatis biovar haemolytica is the β‐hemolytic zone around the colonies on blood agar plates. The protein responsible is the secreted toxin named GtxA (Gallibacterium toxin A; Kristensen et al. 2010; Tang et al. 2020). GtxA exhibits hemolytic activity against erythrocytes from a wide variety of hosts and leukotoxic activity against the chicken macrophage cell line, HD11 (Tang and Bojesen 2020). Immune cells are considered the main target of the toxin. RTX are pore‐forming exoproteins secreted via a type I secretion system (T1SS) in Gram‐negative bacteria. Common to these toxins is a series of Ca2+ binding glycine‐rich nonapeptide repeats (repeats in the structural toxin) in the carboxyl terminal of the protein. RTX are expressed by several members of the family Pasteurellaceae, where they may be responsible for the hemolytic and/or leukotoxic phenotype (Frey 2019). RTX toxins are usually transcribed from a four‐gene operon comprised of rtxC, rtxA, rtxB and rtxD (in order of transcription). The gtx locus, however, has an atypical genetic organization with gtxA and gtxC located together and the gtxB, gtxD and gtxE (a tolC homolog) genes located elsewhere in the genome. In G. anatis, gtxC encodes an activation protein that acetylates the effector (toxin), encoded by gtxA. GtxA is unusually large and, with a size of 2038 amino acids, it is almost twice as large as HlyA, the archetypal RTX expressed by some Escherichia coli. The gtxB and gtxD genes encode transport proteins, which together with the outer‐membrane protein (OMP) TolC, comprise the T1SS responsible for export of the activated GtxA toxin. GtxA has two domains: a C‐terminal domain homologous to that found in other RTXs, which is responsible for the hemolytic function, and an N‐terminal domain of approximately 950 amino acids, which is of unknown function and which has no obvious homologs. The N‐terminus is required for full hemolytic activity and the leukotoxic activity of the toxin (Kristensen et al. 2010). This part of GtxA shows some sequence similarity to the eukaryotic cytoskeletal proteins Talin‐A/B from the amoeba Dictyostelium discoideum and hence may have the capacity to bind to actin, vinculin, and/or integrins. A similar structure is seen in the 238 kDa RTX‐like toxin named AvxA in A. paragallinarum. In AvxA, only the C‐terminal part of the protein shows homology to other RTX toxins whereas the N‐terminal acts as a serine protease (Küng and Frey 2013). GtxA is thought to play a crucial role in pathogenesis and gtxA deletion mutants are severely attenuated both in vitro and in vivo (Kristensen et al. 2010; Tang et al. 2020). The hemolytic activity is seen against a wide range of blood cells, including erythrocytes of bovine, ovine, porcine, equine, leporine, and galliform origin. The N‐terminus of GtxA contains a domain with weak homology to Talin, a protein involved in the linkage of the cytoplasmic portion of integrins to the actin cytoskeleton by interaction with vinculin and alpha‐actinin. Actin has many physiologically important roles in the cell, including regulation of immune cell recognition and adherence, production and release of immune cell signaling molecules and phagocytosis. Several classes of bacterial toxins target the actin cytoskeleton of the host cells as an immune evasion strategy. Some high molecular weight toxins belonging to the RTX family, called MARTX (multifunctional autoprocessing RTX), have been shown to bind to and modulate actin. Based on the above it is likely that GtxA mediates immune evasion. An important initial step of the pathogenesis is the ability to adhere to and traverse a host barrier. G. anatis can adhere to chicken epithelial cells and surface expressed fimbria‐like structures have been observed (Bager et al. 2013a). In G. anatis, several F‐17 like fimbriae clusters have been identified (Kudirkiene et al. 2014). F‐17 like fimbriae belong to a group of fimbriae that bind N‐acetyl‐D‐glucosamine (Glc‐NAc) containing cell receptors on mucosal surfaces in the host. F‐17 type fimbria are expressed by several pathogenic strains of E. coli affecting poultry (Le Bouguénec and Bertin 1999). In G. anatis, F17‐like fimbria production is associated with a four‐gene cluster comprised of genes encoding a chaperone(flfD), an usher protein (flfC), an adhesion protein (flfG), and the structural subunit protein (flfA). Assembly and secretion of the structural protein is facilitated by the chaperone and the usher proteins. The adhesion, located at the tip of the fimbrial structure, is responsible for receptor recognition and binding. In the studies by Kudirkiene et al. (2014), fimbrial genes were detected in the genomes of 22 G. anatis strains. F17‐like fimbriae were very common and that most strains carried one to three different fimbrial clusters (Kudirkiene et al. 2014). The clusters grouped into five phylogenetic lineages (Flf, Flf1‐4), with Flf1 being the most common, present in 74% of the genomes investigated. The Flf1 type of fimbriae appeared only to be expressed in vivo, whereas the Flf cluster, represented by the 20.5 kDa protein FlfA, was encoded by 65% of the strains investigated and was expressed in vitro by 79% of these strains tested (Kudirkiene et al. 2014). The genetic variation among the structural fimbrial genes and the adhesion is considerable (Kudirkiene et al. 2014). Polyclonal antibodies raised against the three most common types of the structural subunit proteins do not cross‐react with the other Flf‐types. This is likely a result of selective pressure driven by immune recognition and could indicate the importance of fimbriae during the pathogenesis of G. anatis. FlfA is important for virulence in vivo, as a flfA deletion mutant was significantly attenuated in experimentally infected chickens (Bager et al. 2013a). The investigation also indicated that fimbrial expression may govern the tissue tropism observed for G. anatis. The presence of several fimbrial types could indicate an immune‐derived selection pressure toward increased affinity for different targets in the host tissue and/or represent a functional diversity, where different types of fimbriae play a role at different time points during the infection process. Some strains of G. anatis are capable of agglutinating avian erythrocytes (Zepeda et al. 2009; Ramirez‐Apolinar et al. 2012). Hemagglutination is linked with the expression of hemagglutinins or adhesins capable of binding receptors on the surface of red blood cells. Genome analyses indicate the presence of several putative hemagglutinins, one of which that has been localized in outer‐membrane vesicles (OMVs) released from G. anatis. No direct link has been established between presence of hemagglutinins and ability to induce hemagglutination, yet hemagglutinating activity have been shown to be important for other poultry pathogens, such as A. paragallinarum. Most Gram‐negative bacteria release OMVs in a process where the outer membrane detaches and eventually buds off leading to a release of spherical, bilayered membrane structures, which have been associated with a tremendous functional diversity. OMVs mainly consist of outer‐membrane components, such as membrane‐associated proteins and LPS, but can also contain periplasmic components and even compounds of cytoplasmic origin such as DNA. G. anatis produces OMVs with a protein content that varies according to growth condition and growth phase (Bager et al. 2013b). OMVs have been hypothesized involved in multiple processes including coping with changing environments (e.g. in the host). To study the formation of OMVs, an OMV‐overproducing mutant of G. anatis was created by deleting the tolR gene, which encodes a protein in the Tol‐Pal system that anchors the outer membrane to the inner membrane and thus, is important for membrane stability and integrity (Lloubès et al. 2001). The specific functions of Gallibacterium OMVs have not been determined. OMVs may facilitate elimination of misfolded or excess proteins or may enable replacement of lipids in the outer membrane during growth. The presence of specific contents, including proteins, lipids, or polysaccharides, depends on the growth conditions; however, indicating an active role of the OMVs. The hypervesiculating G. anatis ΔtolR mutant produces OMVs that are much more uniform and less affected by environmental changes compared to wild‐type OMVs (Antenucci et al. 2020). OMVs have also been suggested a role in preserving proteins sensitive to environmental degradation during delivery to the end destination. Transport of proteases and hemolysins by OMVs has been shown for the related Actinobacillus pleuropneumoniae and A. paragallinarum. However, vesicles from G. anatis do not seem to be involved in either hemolysis or proteolysis. A few proteins have been identified as being a part of the OMVs, including a possible hemagglutinin with sequence similarity to the filamentous hemagglutinin protein precursor FhaB from Bordetella pertussis, which is known to be important for colonization of the host mucosa. Another OMV associated protein in G. anatis is MDN1, an AAA ATPase containing a vWA domain, which has been suggested to be important for the bacterial stress response, cell adhesion, and/or biofilm formation in other species. G. anatis OMVs could indirectly act as virulence‐mediating factors important for survival in the host including modulation of the host immune response, acting as target for phages, or being involved in the binding and removal of anti‐bacterial substances including different antibiotics. As the production and content of OMVs seem affected by the surrounding environment, it is likely that additional host factors, such as those found in serum play a role in regulation and function of the OMVs. The number of OMVs produced as well as the protein profile dramatically changes when G. anatis is incubated in the presence of serum, supporting the hypothesis that the OMVs might play a role in the bacterium’s interaction with its natural host. Bacterial capsules composed of extracellular polysaccharides are expressed by a broad range of Gram‐negative and Gram‐positive pathogens. Capsule expression affects adhesion, cell–cell interactions, and immune evasion. The presence of a thin capsule on the surface of G. anatis was observed by transmission electron microscopy but it disappeared after subcultivation. The function of the capsule of Gallibacterium has not been determined in detail. Interestingly, a capsule‐knockout mutant (ΔgexD) proved to be more virulent than its wild‐type counterpart (Bojesen et al. 2011a). Although further studies are needed to understand that finding, removal of the capsule may have led to the exposure of virulence factors such as adhesins. Proteases are enzymes that catalyze the cleavage of peptide bonds in proteins or peptides. Proteases catalyze essential functions of pathogenic bacteria and metalloproteases are one class of proteases that play important functions in virulence during colonization, nutrient acquisition, immune evasion, and bacterial invasion into the systemic circulation. Modulation of the host immune response may be facilitated by enzymes acting on serum components such as Ig and proteins of the complement system. G. anatis expresses metalloproteases capable of degrading avian IgG (Garcia‐Gomez et al. 2005), which may promote immune evasion. The exact roles of IgG‐degrading metalloproteases are not known, but it has been speculated that these proteins could be responsible for the host‐specific pathogenicity observed for some strains. Several additional metalloproteases, including an extracellular protein with a metal‐dependent endonuclease domain, a zinc metalloprotease, and an ATP‐dependent metalloprotease have been identified in the genomes of G. anatis. Bacterial biofilms are structured cell communities embedded in a polymeric matrix that adhere to inert surfaces and live tissue. Clinically, biofilm formation is associated with persistent and chronic infections and increased resistance to antimicrobials. G. anatis is capable of binding to inert surfaces, which is regarded as a first step toward biofilm formation (Vaca et al. 2011). The biofilm forming ability varies between isolates of G. anatis. No clear correlation appears to exist between formation of biofilm and pathogenicity. With the GtxA toxin considered a major virulence factor the regulation of virulence has mainly focused on the regulation of expression of this protein. In in vitro experiments, GtxA was shown to be expressed in a growth phase dependent manner. The expression of the gtxA gene, as assessed by Northern blotting, was detected during the entire exponential growth and early stationary phases with a transcriptional peak during the late exponential phase. No expression was detected during the stationary phase. The amount of secreted toxin protein appeared to follow the transcription pattern closely. Secreted GtxA appeared to get degraded quickly and no accumulation was observed (Kristensen et al. 2010). A similar expression pattern was demonstrated in a collection of vastly different G. anatis strains (Kristensen et al. 2011). In healthy animals, G. anatis can colonize the upper respiratory tract and the lower reproductive tract without causing immediate lesions or clinical signs. In diseased birds, the organism causes severe lesions in the reproductive tract (Bojesen et al. 2004; Paudel et al. 2013, 2014b). In hens, this typically includes regression of the ovarian follicles some of which may develop a lightly yellow color and a shape like a prune (Figure 13.2). The GtxA toxin appears to be specifically involved in the ovarian disease process as hens infected with a gtxA deletion mutant only develop minute follicular changes compared with wild‐type infected birds. Also, the F17‐like fimbriae seem to take part in the ovarian tropism as an flfA deletion mutant induced far less lesion in the ovarian tissue than the wild type (Bager et al. 2013a
13
Other Pasteurellaceae: Avibacterium, Bibersteinia, Gallibacterium, Glaesserella, and Histophilus
Introduction
Avibacterium
Types of Disease and Pathologic Changes
Virulence Factors and Pathogenomics
Pathogenesis
Immunity
Control
Gaps in Knowledge and Anticipated Directions
Bibersteinia
Source of Infection
Types of Disease and Pathologic Changes
Virulence Factors and Pathogenomics
Pathogenesis
Multiplication
Evasion of Host Defenses
Damage
Immunity
Control
Gaps in Knowledge and Anticipated Directions
Gallibacterium
Pathogenic Species
Source of Infection: Ecology, Evolution, and Epidemiology
Types of Disease and Pathologic Changes
Virulence Factors and Pathogenomics
Repeats in the Structural Toxin‐like Toxin GtxA
Fimbriae
Hemagglutination
Outer‐Membrane Vesicles
Capsule
Metalloproteases
Biofilm Formation
Regulation of Virulence
Pathogenesis
Stay updated, free articles. Join our Telegram channel

Full access? Get Clinical Tree
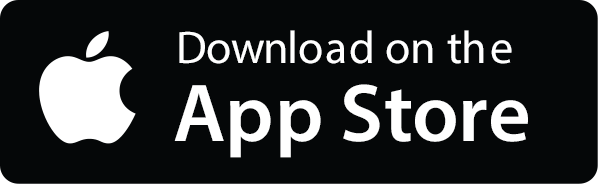
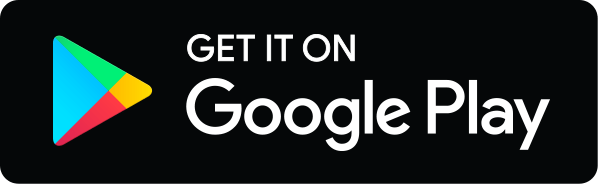