John F. Prescott The speed of progress in understanding how bacteria cause disease is providing novel insights and perspectives on pathogens and the pathogenesis of bacterial infections at an almost overwhelming rate. As the tsunami of antimicrobial resistance threatens our long‐standing expectation that we can successfully treat bacterial infections with existing antimicrobial drugs, understanding how bacterial pathogens of animals cause disease is of fundamental value in designing new and better ways to counter infections. Combined with rapid diagnosis of specific infections, novel antimicrobial treatments based on understanding the unique weaknesses of pathogens, such as those discussed in Chapter 6, can be targeted in ways that could overcome the inherently highly untargeted and resistance‐enhancing nature of most current antimicrobial therapies. Although an overview of the basic themes in bacterial pathogenesis provides a conceptual skeleton for the extensive details of individual pathogens and their interaction with the host given in later chapters, understanding of virulence and pathogenicity is changing rapidly. The fundamental concepts have withstood the test of time, but new knowledge has brought the complexities of host–pathogen interactions into sharper focus and has identified both important broad new topics as well as nuances not recognized previously. Although more is understood about bacteria, especially through the application of genome sequencing and related technologies such as RNAseq (Chapters 3, 4), bacterial infections seem to be increasing and changing, especially those associated with increased antibiotic resistance, driven both by exposure to increasingly powerful antibiotics and by changes in affected patient populations. Numerous anthropogenic activities, including antibiotic use at both therapeutic and subtherapeutic concentrations, may be driving bacterial evolution and the selection of pathogens adapted to changed circumstances (Chapter 2). Against the background of stunning advances in technologies, there is increasing recognition of the poor general application of well‐established simple infection control techniques, such as hand washing to reduce the transmission of infection in people and in animals in clinical settings. The fight against bacterial infections requires the disciplined use of hard‐earned knowledge, not simply the development and application of new technology. The basic elements in the establishment of infection by a bacterial pathogen (Figure 1.1) are well established. These are: Figure 1.1 Basic processes in the pathogenesis of bacterial infections. The processes are more stages than steps, occurring often simultaneously, but also progressively and dynamically, integrated by regulatory processes responsive to signals provided by the host and environment. “Regulation” is shown in the sea of blue in which the processes are embedded. For highly virulent, destructive pathogens, “subversion of host cellular processes” does not occur, whereas it is critical for intracellular pathogens. As would be expected for carefully regulated systems, the infection process is a dynamic continuum rather than a clear series of steps, but breaking it down into progressive steps allows ease of understanding. Successful colonization of the skin or a mucosal surface of the host is usually the first prerequisite of the infectious process. Some organisms need to employ motility and chemotaxis as well as resistance to acid and bile to reach their target host cells. Initial contact between bacterial pathogen and host cell is usually mediated by fimbrial or non‐fimbrial adhesins on the bacterial surface. Binding may result either in extracellular colonization or in internalization of the pathogen. The adhesins bind to specific host cell surface receptors, and both host and organ specificity of infection are determined by differences among animals in cellular receptors for the bacterial adhesins. For example, the Listeria monocytogenes adhesion molecule internalin A (InlA) promotes uptake of the bacterium into intestinal epithelial cells by binding to E‐cadherin. InlA binds to human and rabbit E‐cadherin and causes disease in these species; however, it fails to bind to mouse E‐cadherin and so does not readily cause disease in mice. Interestingly, Wollert et al. (2007) showed that by making two substitutions in InlA, they could increase the binding affinity to mouse E‐cadherin by 10 000‐fold and thereby establish experimental infection in mice. New host adaptations of different infections arise by similar naturally occurring mutations. As many receptors are developmentally regulated, age specificity may also be determined by the receptor that a pathogen binds to. Well‐established examples are known in K99 (F5) pili of porcine and bovine enterotoxigenic Escherichia coli (ETEC), which bind to the intestinal epithelium of neonatal animals, and in F18 pili of porcine ETEC, which bind to the intestinal epithelium of recently weaned pigs. Bacterial pathogens, including those associated with wound infections, may bind to extracellular matrix molecules such as fibronectin, collagen, laminin, or other proteins possessing RGD (Arg‐Gly‐Asp, arginine‐glycine‐aspartic acid) sequences for binding of eukaryotic cell membrane integrins. Bacteria may use “invasins” to mediate their uptake into non‐professional phagocytic host cells after attaching to molecules on the cell surface and activating host cell signaling to facilitate their entry, often through host cell cytoskeletal rearrangement. An excellent example of this is found in the adherence to and invasion of M cells by Yersinia enterocolitica and Yersinia pseudotuberculosis. The outer membrane protein invasin produced by these bacteria binds to β1 integrin on the surface of M cells and triggers uptake of the bacteria in a zipper‐like internalization process (Hauck 2002). This entry provides the bacteria with access to the lymphoid tissue, and to draining lymph nodes, in which the bacteria are well equipped to multiply. Facultative intracellular pathogens may deliberately target macrophages, for example by entering through complement or other lectin‐binding receptors and thus avoiding the oxidative burst that might otherwise kill them. Remarkably, the safest place in the body for these organisms is a macrophage, since these pathogens subsequently interfere with phagosome maturation. After initial association with the host, bacterial pathogens need to evade host defenses and to multiply to numbers sufficient for the infection to be self‐sustaining rather than to be aborted by the host response. The “defensins” or “protectins” involved in the evasion–multiplication process can be divided into those involved in defense against innate immune mechanisms and those involved in defense against specific immune mechanisms. Innate immunity can be overcome in a wide variety of ways (discussed throughout the book, in particular Chapter 5). The lack of available iron that restricts the growth of many bacteria within the body is an important defense mechanism because iron is critical for iron‐containing cofactors for enzymes required for primary and secondary bacterial metabolism. This limitation is often overcome by the iron‐acquisition systems of pathogens. Many organisms, particularly those that cause septicemia and pneumonia, have prominent, usually carbohydrate, capsules that help the organism resist phagocytosis in the absence of antibodies. Some capsules mimic host matrices so that the organisms are unrecognized by phagocytes. The lipopolysaccharide (LPS) molecules of some Gram‐negative bacteria can protect them from the membrane attack complex of complement or from the insertion of antimicrobial peptides. Some bacteria, such as streptococci, can break down complement components through C5a peptidase or other proteases. Other bacteria may destroy or impair phagocytic cells through their leukocidins such as the RTX (repeats in the structural toxin) toxins or enable bacteria to survive inside phagocytes through enzymes such as superoxide dismutases or catalases. Acquired immunity can be overcome in numerous ways (Chapter 5). These include the ability to degrade immunoglobulins with enzymes such as the immunoglobulin A (IgA) proteases, or the ability to alter the antigenicity of cell surface components such as fimbriae or outer membrane proteins. Bacterial superantigens can dramatically upregulate certain T cell subsets with specific Vβ regions, which may result not only in a cytokine storm, which confuses the immune system, but also in the deletion of these cells from the immune repertoire. Bacterial damage to the host is usually essential for immediate or long‐term acquisition of the nutrients that the bacterium needs to thrive and to continue its pathogenic lifestyle. Infection does not always lead to disease, which is only one of the possible outcomes of bacteria–host interaction. Other outcomes include commensalism, latency, or quiet parasitism. Among the wide variety of “offensins” produced by bacteria are many different types of toxins. Toxins can be classified in different though not fully satisfactory ways, with that based on activity the most logical. Type I toxins, the membrane‐acting toxins, bind to cell surface receptors to transduce a signal that results in the activation of host cell pathways, leading to aberrant cell metabolism. Examples in E. coli include the heat‐stable enterotoxin, STa, which binds to the receptor for guanylyl cyclase, resulting in hypersecretion due to excessive levels of cyclic guanosine monophosphate (cGMP), and the cytotoxic necrotizing factor toxins, which activate Rho guanosine triphosphatases (GTPases), resulting in cytoskeletal rearrangements. The superantigens fall into this class. Type II toxins, the membrane‐damaging toxins, include the membrane channel‐forming toxins using the β‐barrel structure (e.g. Staphylococcus aureus α‐toxin), channel‐forming toxins involving α‐helix formation, the large range of thiol‐activated cholesterol‐binding cytolysins, and the RTX toxins. Type II toxins that damage membranes enzymatically also include the phospholipases of many bacteria. Type III toxins, the intracellular toxins, are toxins that enter and are active within the cell. These are often active‐binding two‐component toxin molecules. Examples include the adenosine diphosphate (ADP)‐ribosyl transferases (e.g. the E. coli heat‐labile enterotoxin, ), the N‐glycosidases (e.g. the Shiga toxins), the adenylate cyclases (e.g. the Bordetella bronchiseptica adenylate cyclase toxin), and the metalloendoproteases of the clostridial neurotoxins. Tissue damage and impairment of host function is often due to the inflammatory response mounted by the host in response to infection with a bacterial pathogen, recognition of different pathogen‐associated molecular patterns (PAMPs) and activation of the host’s pattern‐recognition receptors (PRRs). Sepsis represents an extreme case in which hyperresponsiveness to LPS and/or other host signaling molecules unleashes an excessive inflammatory response, resulting in vascular damage, hypotension, and multiple organ damage. The inflammatory response mounted by the host may also provide a point of entry for certain invasive enteric pathogens. Although not often considered in a discussion of bacterial pathogenesis, a crucial feature of bacterial pathogens is their ability to use their pathogenic nature to ensure transmission from the host, either back into their environmental reservoir or directly to other susceptible hosts. Depending on the infection, further transmission to animals may be immediate or involve many years. An important aspect of transmission involves bacterial infections of animals, which are important primarily because of the transmission of organisms from animals to humans. In some cases, as with Shiga toxin‐producing E. coli O157:H7, the bacteria are normal flora in the intestine of ruminants, in which they do not cause disease; however, they do induce severe disease following transmission to humans. A similar situation exists for Campylobacter jejuni and most serotypes of Salmonella in poultry. Efficient transfer from their reservoir hosts to accidental host occurs directly through contamination of foods of animal origin and indirectly through fecal contamination of water and the environment. The outcome of infection is dependent on complex multistep processes involving the host, pathogen, environment, and their interactions. Although much is known about virulence and virulence‐associated genes in bacterial pathogens, and about the diseases they cause, how bacteria orchestrate the process at a regulatory level in terms of timing, response to different host signals, environments, and structures, is an area for considerable further investigation. Bacteria have an astounding ability to sense and to respond rapidly to their environment. Bacteria–host–environment communication systems important in pathogenesis may involve combinations of bacterial type III secretion systems (T3SS), type IV secretion systems, host cell cytoskeletal rearrangement, quorum sensing, two‐component regulatory systems, stress responses and regulatory RNAs. Studies of T3SS have identified a conservation of the secretion apparatus and a remarkable diversity in the effector functions mediated by the systems in extensively investigated bacterial pathogens such as Salmonella, enteropathogenic E. coli (EPEC), enterohemorrhagic E. coli (EHEC), and Yersinia, among others. The effectors of the T3SS are virulence factors that interact with specific host cell structures and factors that set off complex host cell pathways. Pathogenic bacteria need to be aware of their environment to know when to deploy their virulence genes. Cues to bacterial location are as diverse as temperature, pH, growth phase, nutrient availability, oxygen levels, ion concentrations, and quorum sensing molecules, or often combinations of these cues. Depending on the environment, some virulence genes may be upregulated while others are downregulated, only to reverse as the environment changes. The regulation of virulence genes is highly complex, with several regulators controlling the expression of a particular virulence gene and with coordinated and dynamic regulation of genes whose products are required under the same conditions (Bervoets and Charlier 2019; Barrientos et al. 2021; Felden and Augagneur 2021; Ishii and Eguchi 2021) although obtaining an integrated view of these interactions is in its infancy (Huang et al. 2019). How bacteria have evolved to cause disease in animals is truly remarkable in variety and complexity. Given the wide range of bacterial pathogens in different animal species, veterinary microbiologists have insights into this variety, dating back to the start of the microbiology revolution. The astounding ways in which such tiny packages cause so many different diseases is the subject of this book. Understanding infection and the definitions of virulence and pathogenicity has a long and fascinating history. Earlier definitions of virulence and pathogenicity derived from historic studies of classic bacterial pathogens (“Koch’s postulates”), many of which have been successfully controlled by immunization, hygiene, or antimicrobial drugs. Koch’s postulates, developed to define causation in infectious disease, ruled for 100 years (Wassenaar and Gaastra 2001). Early understanding of virulence and pathogenicity was primarily pathogen‐centered and often does not work as well for opportunist pathogens, where host factors are more important than pathogen factors, as it does for primary pathogens. A pathogen is a microbe capable of causing host damage, pathogenicity is the capacity of a microbe to cause damage in a host, and virulence is the relative capacity of a microbe to cause such damage (Casadevall and Pirofski 1999). A virulence factor (virulence determinant) was defined by Casadevall and Pirofski (1999) as a component of a pathogen that damages the host. Pathogenesis is the process through which bacteria cause disease. Detailed understanding of virulence and specifically its genetic basis was importantly updated by Falkow (1988
1
Themes in Bacterial Pathogenesis
Introduction
The Basic Elements of Bacterial Pathogenesis
Pathogen Association with the Host
Pathogen Damage to the Host
Pathogen Transmission from the Host
Regulation is Critical in Orchestrating Pathogenesis
Concepts of Bacterial Virulence are Being Refined
Understanding Virulence and Pathogenicity
Stay updated, free articles. Join our Telegram channel

Full access? Get Clinical Tree
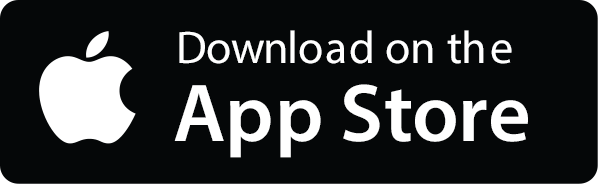
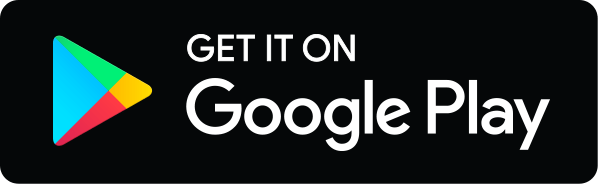