Thomas Bernd Hildebrandt, Joseph Saragusty
Use of Ultrasonography in Wildlife Species
When a great new technology is invented, the inventors rarely imagine all the different uses that will be found for their invention in the years to come. The pioneers in the field of ultrasound applications, Sir Francis Galton who developed the ultrasonic whistle at the end of the 19th century or Paul Langevin who used it to detect icebergs in 1917, certainly did not anticipate the many industrial and medical uses of ultrasonography today. By definition, ultrasound is any sound with frequencies exceeding the upper limit of the human hearing range (maximum 20 kilohertz [kHz]). In the animal kingdom, the capacity for hearing, or the perception of sound, may be as high as about 200 kHz. Known examples are many nocturnal animals such as bats, moths, and other insects and many marine mammals that use sound to locate their prey.
Starting in the 1930s, the role of ultrasonography as a medical treatment tool was established and, in the 1940s, it was also recognized as a diagnostic tool. Gaining acceptance as a noninvasive imaging technology—and thanks to the many technological improvements that facilitated enhancements in sound waves production, reception, processing, and displaying—ultrasonography has become the second most used (after radiography) imaging technology in medicine today. Whereas radiography is known to have adverse effects resulting from radiation exposure, ultrasonography is deemed free of risks at the energies used for diagnostic purposes.51 Generally, the use of ultrasound in medical applications is a trade-off between penetration depth and resolution. The higher the frequency of the sound produced, the better is the resolution but the lower is the penetration depth, which makes visualization through thick body layers difficult or impossible. Imaging by ultrasonography is also restricted by its very limited penetration through bone structures or gas and the need for proper coupling of the transducer, as coupling may be limited by external structures such as feathers, scales, or fur.
Ultrasonography gained a foothold in veterinary medicine starting in the late 1950s when it was used to estimate fat and muscle thickness.71 The first peer reviewed report on such use of the technology appeared in 1961,70 and the first veterinary report appeared a few years later when the technology was used to evaluate pregnancy in ewes.41 The technology helps in visualizing shape, structure, and size, and identifies pathologic lesions in many body structures, including skin, muscles, tendons, and internal organs. Ultrasonography has many uses in veterinary medicine, ranging from assessment of body fat24 to searching for pathologies in soft tissues and tendons, but probably the leading application of this technique today is in reproductive medicine.
Ultrasonography was introduced to the field of reproduction management in humans in the late 1950s, thus opening the way to the exploration and characterization of morphologic, biologic, and pathologic processes and to fertility treatment and intervention under the guidance of ultrasound, thereby revolutionizing the entire field. Despite significant advances in ultrasonographic applications in human and veterinary medicine, adoption of this technology has been slow in zoo and wildlife medicine. Probably the first description of its use as a diagnostic tool in zoo animals was in 1978.56 For a long time, the use of ultrasonography for reproduction management in nondomestic species was sporadic, but over the years, a growing number of ultrasonographic descriptions of various species have been published. Today, this technique has become an indispensable imaging modality in university veterinary hospitals and private clinics, as well as in the veterinary clinics of many zoos.
Various reasons exist for the slow introduction of ultrasonography in the management of nondomestic animals. By definition, species are different from each other in many aspects, including their morphology and specialized structures. For example, significant differences exist in cardiac anatomy and blood flow among the various taxa. Without detailed knowledge of all species, interpretation of body structures seen in each new species may be challenging. Use of carcasses to conduct comparative studies with ultrasonography and conventional anatomic dissection may benefit all. Although some wild animals (e.g., elephants, rhinoceroses, marine mammals) may be trained to allow examination, in the majority of cases, physical restraint, sedation, or anesthesia are required to facilitate safe examination. Effective penetration of ultrasound is limited to certain depths, which makes it often challenging or practically impossible to visualize various internal structures in large-sized animals (e.g., elephants, whales).
In exotic animals, certain specialized structures or features such as the carapace and plastron in tortoises and turtles, feathers and air sacs in birds, scales on many fish and reptilian species, or exoskeleton in many invertebrates may all interfere with the ultrasonographic examination and may require innovative scanning techniques to overcome at least some of these limiting factors that are usually not encountered in human or mainstream veterinary medicine.24 The unique features that characterize different taxonomic groups with relation to ultrasonographic examination and the different ways this imaging technique may be used when working with zoo and wild animals belonging to these taxa will be discussed in this chapter. Recent developments in ultrasonography and modifications of commercial system configurations, the decrease in machine size that makes it more portable and affordable, and the new methods of examination will all facilitate increased use of this tool for health and reproduction management in wildlife species. Intraoperative ultrasonographic applications will not be discussed in this chapter, besides their value as a diagnostic tool to improve decision quality during surgery.
Fish
One of the main considerations in ultrasonography is the quality of coupling. When used in human or domestic species, for example, coupling gel is required to exclude air from the interface between the transducer and the body. If the fish remains in water (this is highly recommended to reduce the risk for trauma and stress), the water provides excellent coupling conditions, so coupling gel is not needed. Existence of a large body of water around the fish would also make it possible to scan its body without making actual contact with it, by holding the transducer at a distance of as much as 2 centimeters (cm), depending on the frequency used. Naturally, the transducer must be waterproof or otherwise wrapped in a way that will preclude the risk of exposure to water (e.g., by placing it inside a latex glove). Since electricity is involved, especially in devices that are connected to the electricity grid, every precaution should be taken to ensure safety. The need to keep the fish in water also precludes the use of radiography, which is yet another advantage of ultrasonography.
Small fish are normally sedated for the examination, and large ones may be physically restrained. For large fish, transducers of 2 to 5 megahertz (MHz) are usually chosen, and for small fish, 7.5 to 16 MHz would be more appropriate for better visualization of the small body structures. Some fish and elasmobranchs have scales or calcified integument on their body surface (see Figure 76-1, D), and these may interfere with the passage of sound waves, resulting in poor visualization. In many of these species, conducting the examination from the ventral surface may produce better results. When this is not sufficient, and when the animal is large enough, transintestinal ultrasonography may be considered. The tissues of fish (skin, muscle) have higher water content compared with tissues in mammals or birds, and this makes the tissues of fish look somewhat different for practitioners who usually work with mammals. It is also important to remember that the velocity of sound wave transmission through muscle decreases with temperature, whereas that in fat increases, so one may distinguish well between muscle and fat at room temperature, but at about 5° C to 11° C, they may look very similar.59
Ultrasonography has been used in the fish industry at least since the early 1980s. The main application of this technology has been for the determination of the sex and maturity status of juvenile and adult fish.55 This has been done in a wide range of fish species such as Coho salmon (Oncorhynchus kisutch), cod (Gadus morhua), Atlantic salmon (Salmo salar), and Pacific herring (Clupea harengus pallasi). Other areas in which ultrasonography has been found to be a useful tool include evaluation of ovarian activity and ovulation,47 health assessment and diagnosis of pathologies,65 anatomic studies,14 and, in aquaculture, muscle development evaluation4 (see Figure 76-1, A through D).
Assessment of reproductive potential, sexual maturity, and pregnancy has also been done in elasmobranchs (sharks and rays) such as nurse sharks (Ginglymostoma cirratum),7 thornback ray (Raja clavata) and small-spotted cat shark (Scyliorhinus canicula),74 and broadnose sevengill sharks (Notorynchus cepedianus).10 Furthermore, ultrasonography has been used for anatomic description and as a diagnostic tool in various species of sharks. Long-term investigations with ultrasonography may also help elucidate unusual phenomena such as intrauterine cannibalism occurring in lamnid sharks.
Amphibians
For at least three reasons, amphibians are well suited for ultrasonographic examination: (1) They do not have external structures such as fur, feathers, or scales that may impede imaging; (2) their coelom usually contains some fluid that helps enhance the quality of the images. This fluid may increase in volume in diseased animals (see Figure 76-1, G); and (3) to varying degrees, depending on the species, they dwell in water, making it possible to scan them while they are submerged in water, as is done in fish. Because of their body sizes, normally transducers ranging from 5 to 16 MHz are used and are placed directly on the animal’s skin, in the water without any direct contact with the animal (Figure 76-2, A), or in contact with the outside of a water-filled plastic container with the animal in it.68 When the transducer is applied directly to the skin or from outside the plastic container, coupling gel is required to enhance image quality. Restraining the animals may be achieved through sedation, direct manual restraint, or placement of the animal inside a water-filled plastic container that restricts its ability to move. If cold water is used, this will further reduce the mobility of the animal, but caution should be taken not to chill the animal too much.
When studying an amphibian by using ultrasonography, the amphibian heart, which is composed of two atria and a single ventricle, is a good point to start the examination. It is located at the ventral midline between the front limbs and may easily be identified because of its pumping activity. Often, the pericardial space contains a moderate amount of fluid, making visualization better. Amphibians lack a diaphragm, so the liver, which, in healthy animals, would have an appearance similar to that in mammals, could be located next to the heart. The gallbladder, which may be as large as the heart, would appear as an anechoic spherical structure near the heart and the liver. Other abdominal organs such as the stomach, gonads, and urinary bladder may also be visualized29,68 (see Figure 76-1, E and G). In both caudates and anurans, especially in those in good body condition, unique fat bodies may be visualized within the coelomic cavity. In anurans, these fat bodies appear as fingerlike projections originating from a common stalk stemming from the base of the gonads. These fat bodies would normally appear to be a bit hyperechoic compared with the liver. In salamanders, these fat bodies have a band shape and may be found between the gonads and the kidneys.
The main applications of ultrasonography in amphibians include assessment of the reproductive status,38 sex determination,29 evaluation of cardiac activity and blood flow (including the use of Doppler ultrasonography),8 and health assessment (e.g., parasitic and neoplastic status) and anatomic studies29,69 (see Figure 76-1, E through G).
Reptiles
Reptiles are good candidates for ultrasonography. It may be used either as a stand-alone modality or as a complement to radiography, which is used to clearly image the skeleton and the respiratory system, the two body components that ultrasonography cannot image properly. Most reptile species are relatively docile and may be imaged with minimal physical restraint. Some snakes and lizards, however, are less “cooperative” or are poisonous, and special care should be taken to protect the animal and the handler.
Transcutaneous ultrasonography may be problematic in some reptilian species because of the shell or scales that cover their bodies (e.g., Australian bobtail lizard, Tiliqua rugosa; or Komodo dragon, Varanus komodoensis). Data are scarce with regard to the normal anatomy and architecture of the coelomic cavity and visceral organs in many reptilian species, as well as the way these structures are viewed in ultrasonographic images, so special care should be taken not to mistake, for example, fat structures for other internal organs. This is especially true because many internal organs in reptiles look quite different from those in mammals. Furthermore, the sizes, shapes, and positions of various internal organs may change, depending on the size of the gastrointestinal and reproductive tracts. When possible, comparative studies between ultrasonography and necropsy are highly recommended. Generally, the lungs in reptiles are positioned dorsal to the other coelomic organs, so ultrasonography is best performed from the lateral or ventral aspect at the region where the lungs are located. In turtles, because of their shell, ultrasonography may be conducted from two restricted windows between the carapace and the plastron. From the front of the animal, between the head, front leg, and thorax, one may visualize the heart, liver, and gallbladder. Preferably a small probe (convex or sector scanner) should be used. From the rear of the animal, next to the pelvic region, the visceral organs, including the kidneys, gonads, urinary bladder, intestines, liver, spleen, and so on, may be viewed. The only exception is the pancake tortoise (Malacochersus tornieri), in which direct scanning through the soft plastron is possible. In snakes and lizards, especially those that are covered with thick hard scales, the best ultrasonographic visualization may be achieved from the ventral aspect of the animals. For this purpose, a platform or table with openings in it may be designed so that the animal may be placed over it. This will allow for extended duration of examination with minimal need for restraint of the animal.
Smaller animals are best imaged with 7.5 to 16 MHz transducers, and large ones may be better examined with 2.0 to 5.0 MHz probes. Coupling gel should be applied to achieve proper coupling. In some cases, when the ventral and lateral aspects of the animal are covered with very thick scales or scutes that partially or completely block ultrasound waves (e.g., Tiliqua spp., Corucia zebrata), coupling gel may be applied and left for 15 to 30 minutes so that the scales absorb some of the gel for better imaging. The alternative is to submerge the region of interest (excluding the head) in water to improve coupling. Placing the probe between the scales or from a more lateral position may also help. In larger reptiles, a good alternative would be to use specialized transducers that allow for transintestinal endosonography (see Figure 76-2, C). By inserting the transducer through the cloaca into the intestine, the entire urogenital tract, intestines, adrenal, and fat bodies may be visualized.27
The primary use of ultrasonography in reptiles is probably for the assessment of the reproductive tract and reproductive status (see Figure 76-2, B, C, and E). This was done in a wide variety of terrestrial, marine, and fresh-water reptilian species.39,60,62 Sex determination in sub-adult or monomorphic reptiles has also been done with the use of ultrasonography, for example, in komodo dragons (Varanus komodoensis), white-throated monitors (Varanus albigularis), Gila monsters (Heloderma suspectum), and beaded lizards (H. horridum).27,52 Ultrasonography has also proven useful for medical diagnosis 45 (see Figure 76-2, D), as well as for ultrasound-guided transcutaneous biopsy.34 Ultrasonography is very useful for anatomic, morphologic, and nutritional studies in reptiles.27,64
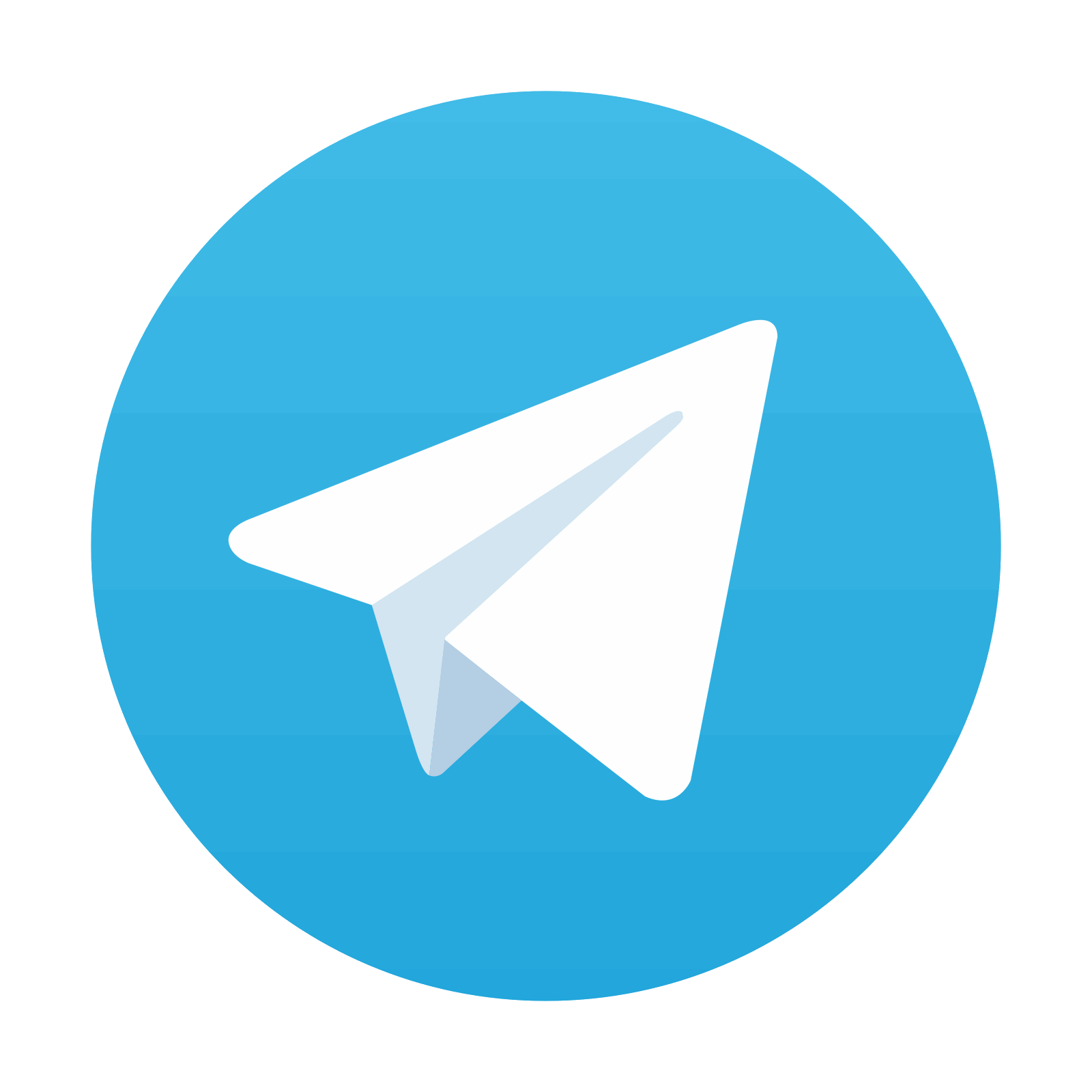
Stay updated, free articles. Join our Telegram channel

Full access? Get Clinical Tree
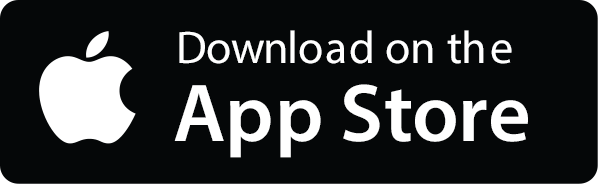
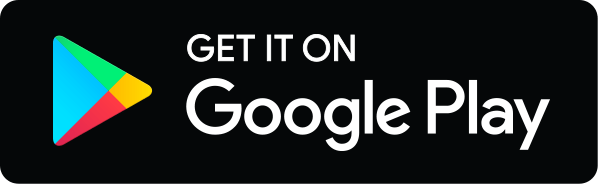