Linda J. Lowenstine, Iga M. Stasiak
Update on Iron Overload in Zoologic Species
Iron overload (IO), also called iron storage disease (ISD) or iron overload disorder, was considered rare in veterinary species until the 1970s and 1980s, after which it became increasingly recognized in many taxa of birds and mammals.37 The current understanding of molecular mechanisms of iron metabolism and of diseases associated with excessive iron accumulation has greatly expanded as a result of research in humans, mice, birds, and bats.5,24,28,32,40,64 An international workshop highlighted the concern about the impact of ISD on captive management of black rhinoceroses and resulted in the publication of a special issue of Journal of Zoo and Wildlife Medicine.23
In this chapter, the term hemosiderosis refers to an accumulation (overload) of stainable iron without morphologic or biochemical evidence of toxicity, and the term “hemochromatosis” indicates a serious state of iron overload, in which organ damage occurs. In contrast, in human medicine, “hemochromatosis” often refers specifically to primary hereditary iron storage disease. The term “iron overload (IO)” connotes too much iron, whereas ISD connotes disease associated with IO. Hemosiderosis may progress to hemochromatosis. However, very high concentrations of iron may occur without evidence of molecular, cellular, or structural damage if sequestration of toxic, labile free iron by ferritin and hemosiderin is adequate to prevent cell death.13,32
Iron overload may be primary (implying a constitutional, genetic propensity to accumulate iron, generally as a result of inability to control intestinal absorption, as in human hereditary hemochromatosis) or secondary to a variety of causes, including excessive enteral or parenteral (e.g., transfusions) intake, diseases of the erythron, liver disease (including intoxications and infections), systemic inflammatory processes (with iron sequestration as an antibacterial defense), stress, chronic renal disease, and malnutrition.1,61
The majority of iron in the body is present in hemoglobin in the red blood cells (RBCs) and is recycled from senescent erythrocytes by macrophages in the spleen, bone marrow, and liver.1,24 Most of the remainder is stored in the cytosol of cells as ferritin, from which it may be readily accessed, or as hemosiderin, which is a less active, aggregated form. Hemosiderin appears as golden brown granules in hematoxylin and eosin (H&E)–stained sections and is confirmed to be iron by blue precipitates formed by Prussian blue techniques (e.g., Perl). Ferritin is not visible with H&E staining but appears as a faint blue “wash” in Prussian blue stains.3 Hepatocytes are the major site of iron storage in overload states. The shift from ferritin to hemosiderin in hepatocytes may be caused by increasing iron concentrations or by hepatocellular injury, atrophy, or autophagy; thus, increases in stainable granular iron may reflect a relative, rather than absolute, increase in intracellular iron concentration.
Review of Iron Absorption and Transport and Liver Handling of Iron
Iron is essential for almost all life forms from prokaryotes to vertebrates, but it is also a toxin. Organisms have thus evolved complex regulatory mechanisms for ensuring enough iron to meet metabolic needs while preventing excessive uptake. Since “off-loading” of body iron is limited, control is primarily at the level of intestinal uptake.
Dietary iron is present in two main forms: heme and non-heme.61 Heme-iron is highly bioavailable; it is released by proteolytic digestion of myoglobin and hemoglobin in the small intestine and is the major source of iron in carnivores. Non-heme iron is complexed with organic acids or peptides and is the predominant form of dietary iron available to omnivorous, frugivorous, folivorous, granivorous, and other plant-eating species.32 In humans, non-heme iron in the oxidized state is reduced and then taken up by the cellular iron importer divalent metal transporter-1 (DMT-1).23,61 Gastric acid enhances the solubility and absorption of non-heme iron complexes, and dietary vitamin C increases iron absorption by forming highly bioavailable, soluble iron complexes.1 Iron bioavailability is decreased by iron chelating compounds in the diet.
Within the enterocyte, depending on the metabolic demand, iron is either bound to the iron storage protein, ferritin, in the cytosol or is exported into plasma via ferroportin. Ferroportin, a transmembrane protein and the most important iron exporter, is also expressed in macrophages, hepatocytes, and placental trophoblasts.5,23,32 Iron not transferred to plasma is sloughed off with exfoliating enterocytes and eliminated through feces. Oxidized iron is taken up by plasma transferrin for distribution throughout the body, and a majority is carried to erythropoeitic cells.
Hepcidin, the key iron regulatory hormone, expressed primarily in the liver, is induced when tissue and plasma iron levels are high.24 Hepcidin induction results in the internalization and degradation of ferroportin, thus downregulating enteric iron absorption and iron mobilization from cellular stores and causing depletion of the plasma iron pool. Hepcidin production is also induced in inflammatory states and appears to be a key mediator in anemia of chronic disease. Hepcidin is strongly suppressed in states requiring accelerated erythropoiesis.
The active hepcidin peptide appears to be highly conserved among vertebrate taxa with homologous sequences in over 51 different highly diverse species, including fish, amphibians, reptiles, and mammals.27 However, hepcidin has not been confirmed in birds (pigeons and chickens).
In humans, in a majority of cases, hereditary hemochromatosis is associated with a deficiency in hepcidin because of mutations in the hepcidin (HAMP) gene itself or its regulators.23,61 Investigations of the role of hepcidin in the regulation of iron balance in susceptible exotic species have included genetic studies in rhinoceroses and red deer (Cervus elaphus) and experimental studies in bats.6,23,50,64 Since hepcidin may not be present in birds, other iron-regulatory molecules may be of primary importance. Those identified include DMT1 in chickens and mynahs; ferroportin (Ireg1) in chickens and mynahs; intestinal cytochrome B reductase, serum and ovo-transferrins in chickens; and ferritin in several species.2,42,43,65
In mammals, iron crosses the placenta via placental DMT-1, ferroportin, and transferrins, mostly in the late stages of pregnancy.36 Unless born to an iron-deficient dam, infants are endowed with sufficient iron to carry them through early postnatal life to compensate for the relatively low concentration of iron in milk. This may account for the common finding of hemosiderosis in the livers of neonates of several species of mammals.37,62 Transfer of iron to the embryos of birds and presumably fish, amphibians, and reptiles occurs through the yolk.
Consequences of Iron Overload
Unbound iron is cytotoxic and causes peroxidation of membranes, cell death, and organ dysfunction.3 Cirrhotic liver failure (“pigmentary cirrhosis”), hepatocellular carcinoma, type 2 diabetes (“bronze diabetes”), male sexual dysfunction, arthropathies, and heart disease (cardiomyopathy and fatal dysrhythmias) are consequences of untreated ISD in humans.3,5,32,61
IO increases susceptibility to bacterial and fungal infections through direct effects on immune system function and by providing iron for microbial growth.5,23,67 This is highlighted by deaths caused by sepsis in human hemochromatosis, yersinosis in birds with IO, and, possibly, cases of atypical mycobacteriosis and aspergillosis in black rhinos.5,13,30,53 Increasing evidence suggests that elevated total body iron stores may be related to the development of metabolic syndrome and type 2 diabetes in humans and, possibly, in bottlenose dolphins and black rhinoceroses, perhaps through endogenous corticosteroid production.26,37,46,66,75
Diagnosis
Early diagnosis of ISD poses a challenge, as clinical signs are not specific and often not apparent until disease is advanced. Clinical signs are typically associated with liver failure and ascites, hepatomegaly, weight loss, icterus, anorexia, vomiting (in lemurs), “malaise” (in dolphins) and, in birds, cardiomegaly, dyspnea, coughing, or wheezing.15,39,63 Liver enzymes are often elevated, and hypoproteinemia ensues as the disease progresses to liver failure. Signs in domestic cattle and red deer include poor hair coat, weight loss in the face of a good appetite, and poor bone quality leading to loss of incisors and fractures in young animals.31,50 Radiography is useful in identifying hepatomegaly and cardiomegaly in birds with ISD.30,40 Liver biopsy, for histologic grading of iron accumulation and liver damage, and chemical analysis of iron concentration remain the gold standard for diagnosis of ISD in exotic species.40,62,71 Because of the invasiveness of liver biopsy, serum “iron analytes” (iron, total iron binding capacity, % transferrin saturation, and ferritin) have been used in humans and mammalian species and have proven useful in the diagnosis, determination of prognosis, and treatment of ISD.5,14,29,39,53,61,62,70
Serum ferritin (SF) is considered the best test for noninvasive measurement of body iron status in mammalian species.12,21,62 In humans, SF level vary with age and gender (males greater than females) and increases in inflammatory processes and conditions such as hyperthyroidism, chronic hepatitis, alcohol-induced liver disease, and neoplasia, which limits its usefulness as a stand-alone test.5,32,61 In humans, normal values are less than 200 microgram per liter (µg/L) in women and <300 µg/L in men, whereas SF >1000 indicates IO and >5000 indicates ISD.32 Variation in the molecular structure of ferritin requires species-specific radioimmunoassays, only available for humans, dogs, cats, cattle, horses (used for bats), pigs, rhinoceroses (used for tapirs), lemurs (used for callitrichids), dolphins, and fur seals (Kansas State Veterinary Diagnostic Laboratory, Comparative Hematology, Manhattan, KS).21,51,62,70
Serum iron (SI) levels reflect the total amount of iron in blood, including transferrin-bound and non–transferrin-bound iron. In mammalian species and birds, diurnal and seasonal variations exist in SI.7 Variations occur among closely related taxa, necessitating evaluation of the usefulness of serum iron analyte testing on a species-by-species basis.70 Total iron binding capacity (TIBC) and the calculated percentage transferrin saturation (%TS) are also used as indicators of body iron stores, with %TS considered more reliable than SI, TIBC, or both. In humans and many mammalian species, transferrin is normally about 30% saturated. TS persistently over 45% strongly suggests hereditary hemochromatosis in humans.61 A panel consisting of SF, SI, and %TS is recommended for assessment of body iron levels in mammals.
In birds, SI analytes seem to be of little diagnostic value, although relative changes in SI and %TS may be used to monitor treatment.27,40,69 SF assays are not yet commercially available for avian species, and liver biopsy remains the only reliable antemortem diagnostic test.13,30,37,40 The use of magnetic resonance imaging (MRI) to assess hepatic and cardiac iron stores is an accepted modality in humans; however, limitations exist.32,61 In pigeons with experimentally induced IO and hornbills with spontaneous ISD, MRI signal intensity corresponded well with hepatic iron concentration.38,56
Liver iron concentration (LIC) may be assessed histologically or analytically. Semi-quantitative assessment of Prussian blue–stained liver sections, using scoring schemes or morphometric techniques, approximates analytic quantification for comparisons of iron levels and assessing response to treatment.7,8,13,18,54,62,69 Analytic determination of LIC may be performed on fresh, frozen or formalin fixed biopsies or from deparaffinized samples retrieved from tissue blocks. Small volumes of tissue are best measured using atomic absorption spectrophotometry (AAS) or inductively coupled plasma mass spectroscopy (ICP-MS). Iron concentration is expressed as millimoles per gram (mmol/g), micrograms per gram (µg/g), grams per kilogram (g/kg) or milligrams per gram (mg/g) (parts per million [ppm]) on either a wet weight (ww) or dry weight (dw) basis (Box 69-1).
Normal reference ranges for LIC are lacking for most species in zoologic medicine. In humans, the normal is 400 to 2000 µg/g dw in men and 100 to 1600 in women; mild to moderate IO is 3000 to 10,000, and greater than 10,000 indicates potential toxicity. Fibrosis is always present at 22,000 µg/g.3 The interpretation of LIC in animals is complicated by seasonal variation associated with life history events, for example, reproduction and molting in birds and migration in reindeer.7,30
Iron Overload Syndromes in Zoologic Species
Amphibians and Reptiles
Hepatocellular hemosiderosis, often with hepatic lipidosis, is not uncommon in amphibians and reptiles and is likely secondary to systemic inflammation or inanition, not caused by a primary susceptibility to ISD.37 Liver iron reference values are generally lacking, but mean LIC in Chinese alligators (Alligator sinensus) was 380 ppm dw.73
Birds
Both primary ISD and secondary ISD occur in avian species, with toucans, birds of paradise, mynahs, and starlings considered “susceptible” or “iron sensitive” taxa (Box 69-2).13,30,37,40 Liver iron concentrations of 6683 to 23,078 µg/g dw, found in clinically normal mynahs, are well above the 487 to 701 in adult chickens and within the range of IO and ISD in humans.43 A primary genetic basis for iron overload in mynahs (Gracula religiosa and Acridotheres tristis) is likely. Compared with collared doves (Streptopelia decaocto), mynahs have much higher uptake and retention of dietary iron and demonstrate high levels of expression of the intestinal iron transporters DMT-1 and intestinal Ireg1 (ferroportin).41,42 This supports a working hypothesis that natural diets for which “sensitive” species have evolved are low in bioavailable iron leading to selection for enhanced iron uptake and storage.60 However, diets in captivity that provide too much available iron may exacerbate primary ISD, as shown in starlings.16
Reports of ISD in psittacines are interesting because this diverse order contains both generalist and specialized feeders. Species that have evolved to use low iron diets would be expected to be more “iron-sensitive” compared with other species. This seems to be the case for lorids, adapted to nectivorous and palynivorous (pollen) diets, and the red-spectacled Amazon (Amazona pretrei), adapted to a pine nut diet.54,68 Liver iron concentration in affected lories was 3500 to 7600 ppm ww (about 11,550 to 25,000 µg/g dw), comparable with LIC in mynahs. A report of two diabetic macaws with ISD parallels the frequent occurrence of diabetes in human hemochromatosis.22,61
In flamingos, moderate to marked hepatocellular and Kupffer cell hemosiderosis increases with age and is associated with hepatitis, cirrhosis, and hepatocellular carcinoma in older birds. Concurrent diseases that could drive ISD (e.g. pododermatitis, aspergillosis, and amyloidosis) are present in some, but not all, birds. This species warrants further investigation to determine whether an evolutionary predisposition exists or if ISD is secondary to management.
Hemosiderosis in other orders of birds such as the anseriforms appears to be secondary to intoxications (e.g., lead), infections, captive diets, or seasonal life history events such as molt and reproduction.30,37 Studies in Eider ducks have indicated that inanition increases LIC; however, in wild black-necked swans (Cygnus melanocoryphus), elevated SI in the face of starvation was considered to indicate mobilization and reduction of tissue iron storage.7,48 In the latter study, serum iron, TIBC, and %TS were used as surrogates for body iron stores but may not accurately reflect LIC.
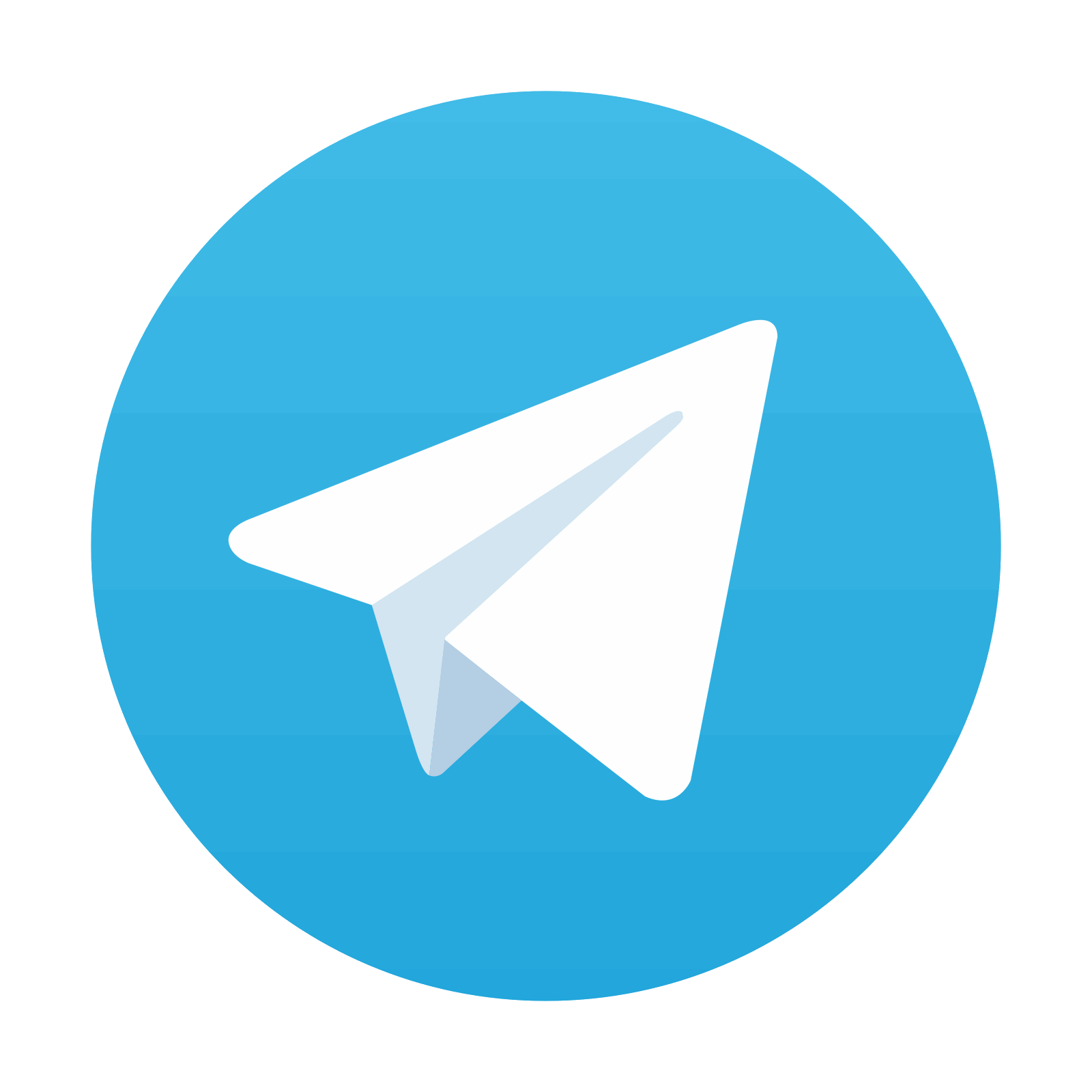
Stay updated, free articles. Join our Telegram channel

Full access? Get Clinical Tree
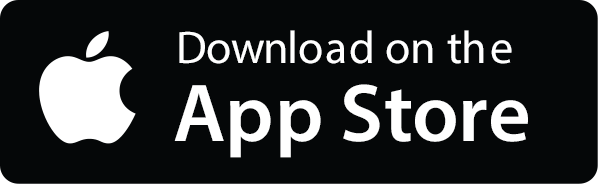
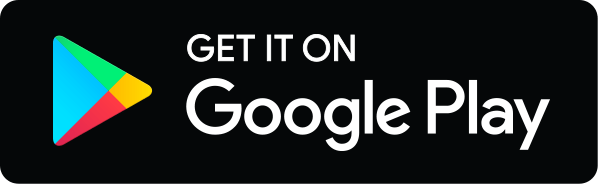