Chapter 25 Tumor Markers B. Hormones and Ectopic Hormones F. Miscellaneous Serum Tumor Markers IV. PROLIFERATION MARKERS/APOPTOSIS B. Thymidine Labeling Index and BrdU Incorporation C. Nucleolar Organizing Regions V. IMMUNOHISTOCHEMISTRY/IMMUNOCYTOCHEMISTRY IX. PROTEOMICS, GENOMICS, METABOLOMICS Biomarkers, particularly tumor markers, represent an exciting tool for the clinical discipline of oncology. However, as various markers of disease, including physiological, biochemical, and genetic changes, are identified, they may become more than useful diagnostic tests. These biomarkers may also play a role in drug discovery and development and become useful tools for predicting the response to therapy and prognosis. To help address the issues surrounding biomarkers and their application to various disciplines of clinical medicine and biomedical research, the National Institutes of Health (NIH) formed a working group on definitions to develop a set of preferred terms and descriptions, along with a conceptual model, that could be broadly applied to the increasing use of biomarkers. The working group defined a biomarker as an objectively measured and evaluated characteristic that is an indicator of a normal biological processes, a pathogenic process, or a response to a therapeutic intervention (Downing, 2000). The NIH Working Group on Definitions also characterized several important applications for biomarkers including their use for diagnosis, for staging disease, as indicators of disease risk, and as tools to predict and monitor clinical responses to therapy (Downing, 2000). This chapter focuses on how various biomarkers have an application to cancer management in veterinary medicine. Ideally, in addition to the attributes listed in the introduction, tumor markers should be both sensitive and specific for the detection of cancer, to minimize both false-positive and false-negative test findings, and they should use methodology that is minimally invasive to increase acceptance and compliance by animal owners. Tumor markers should also reflect the total tumor burden, identify tumor recurrence after treatment, and be unaffected by cancer treatment or adverse events associated with cancer treatment. Tumor markers should be reproducible among laboratories and have a well-defined reference range to distinguish between health and disease. In human medicine, testing for tumor markers is recommended only in situations where it is demonstrated to result in a better patient outcome, increased quality of life, or reduced overall cost of care (Duffy, 2004). The rapid technological advances in immunology, biochemistry, and cell and molecular biology will continue to offer new opportunities to evaluate candidate tumor markers. However, few tumor markers, including those that are commonly used in veterinary medicine, have been rigorously tested. To determine whether a candidate tumor marker has clinical utility, it is vital that it undergo critical evaluation. In evidence-based medicine, there are several criteria for interpreting the usefulness of a diagnostic test (Jaeschke et al., 2002). First, it should be useful for situations or cases where clinicians routinely face diagnostic uncertainty. For example, the diagnostic evaluation of hematuria in an older dog is one situation in which an effective tumor marker would be highly useful. Second, candidate tumor markers should be blindly evaluated against an independent “gold standard” diagnostic test in cancer patients, sick noncancer patients, and healthy patients. Only after the usefulness of a tumor marker has been established can clinicians begin to determine how effectively the test result and its interpretation will improve patient management. The likelihood ratio (Letelier et al., 2002) is one tool that can help clinicians interpret and apply test results, and it may be useful to apply to veterinary tumor markers. Briefly, the clinician must have an idea about the patient’s probability for having cancer based on signalment, history, physical findings, and other factors before tumor marker testing. Given knowledge of the likelihood of a high test value occurring in a patient with cancer compared to the likelihood of a high test value occurring in a patient suspected of cancer that was later ruled out, it is possible to calculate the posttest probability for the patient having cancer given a high, low, or intermediate test result. For the interested reader, the Evidence-Based Medicine Working Group has detailed the critical evaluation of diagnostic tests, results interpretation, and application to clinical patients (‘Users’ Guide to the Medical Literature: A Manual for Evidence-Based Clinical Practice, 2002). Historically, using a broad definition, veterinary tumor markers have included various molecules found in serum, flow cytometry, proliferation and apoptosis markers, immunohistochemistry, cytochemistry, and cytogeneticis. With the advent of new technologies, molecular markers of cancer will become more important in human and veterinary oncology. Likewise, the explosion of the “-omics,” including genomics, proteomics, and metabolomics, may also be important to the diagnosis and management of cancer in the future. Each of these issues will be considered in turn. Oncofetal proteins originate within tumor cells and enter the bloodstream either by secretion from the tumor or as a breakdown product of tumor cells. Normally oncofetal proteins are present during embryogenesis and may increase with certain cancers, making them potentially useful tumor markers. Carcinoembryonic antigen (CEA) and alpha-fetoprotein (AFP) are the most widely used oncofetal protein tumor markers (Garrett and Kurtz, 1986). CEA has been well studied in human medicine, and since the 1970s it has been recognized as a useful marker for cancers of the lung, colon-rectum, breast, ovary, and prostate gland (Go, 1976). CEA is the most useful tumor marker to distinguish benign from malignant pleural effusions (Shirit et al., 2005). Preoperative serum CEA levels may also predict survival in human colorectal cancer patients (Park et al., 2005). In veterinary medicine, CEA has received little attention, and its role as a tumor marker in domestic animal species remains undefined. In humans, AFP is commonly used to diagnose hepatocellular carcinoma and predict its prognosis (Zhou et al., 2006). AFP has been measured in the serum of cancer-bearing dogs (Hahn and Richardson, 1995). However, in this report, the mean serum concentration of AFP in dogs with various malignancies was not significantly different from the mean serum AFP concentration of the 16 dogs without cancer. A single dog with hepatic involvement with lymphoma had a serum AFP concentration >225 ng/ml, suggesting AFP may have a role for diagnosing primary or secondary hepatic cancer in the absence of other serum biochemical abnormalities. Indeed high serum concentrations (>250 ng/ml) of AFP have been detected in a small number of dogs with primary liver tumors (Lowseth et al., 1991). Because serum AFP concentration is higher in canine hepatocellular carcinoma compared to other hepatic diseases, it may be a useful tool for diagnosing hepatocellular carcinoma in dogs (Yamada et al., 1999). In a study of serum AFP concentrations in healthy dogs and dogs with multicentric lymphoma (Lechowski et al., 2002), the mean serum AFP concentration was higher in dogs with lymphoma compared to the healthy dogs. Serum AFP concentration was also found to increase with advancing clinical stage of lymphoma, and decrease to levels similar to normal dogs as the lymphoma went into remission with chemotherapy. These observations suggest serum AFP may be a useful biomarker for determining lymphoma remission in the dog and potentially an early indicator of relapse. Serum AFP has not been carefully evaluated as a tumor marker in other domestic species. Inhibin is a nonsteroidal hormone that is involved in the follicular phase of the human menstrual cycle (Groome et al., 1996). Inhibin has also been identified as a regulatory hormone in the follicular phase of the equine estrous cycle (Medan et al., 2004). Serum inhibin concentrations have been shown to be elevated in mares with granulosa theca cell tumors (GTCT) (Christman et al., 1999). Measuring inhibin concentrations can be helpful in diagnosing equine GTCT, especially if serum testosterone concentrations are not elevated, and distinguishing GTCT from other diseases of the ovary. Increased serum inhibin concentrations have also been identified in dogs with Sertoli cell tumors, making it a potentially useful marker for identifying testicular tumors (Grootenhuis et al., 1990). Increased serum parathyroid hormone (PTH) in the presence of hypercalcemia has been considered sufficient for the diagnosis of primary hyperparathyroidism. However, a retrospective case-control study suggests that normal PTH concentrations in the presence of hypercalcemia are an inappropriate physiological response by the parathyroid gland and are also consistent with primary hyperparathyroidism (Feldman et al., 2005). Although primary hyperparathyroidism is an uncommon disease of dogs and cats, both adenomas and carcinomas of the parathyroid glands are reported in the literature and should be considered among the differential diagnoses. Hypercalcemia of malignancy is a well-recognized paraneoplastic syndrome in animals. Hypercalcemia of malignancy may arise from local bone resorption stimulated by metastatic bone lesions or through endocrine factors that disrupt normal calcium homeostasis (Clines and Guise, 2005). Ectopic production of PTH by tumors is a rare phenomenon, and most humoral hypercalcemia of malignancy may be explained by the inappropriate production of parathyroid hormone-related protein (PTHrP) by a variety of tumors. Canine PTHrP is similar in structure and function to PTH (Rosol et al., 1995). In dogs, PTHrP has been implicated in hypercalcemia associated with apocrine gland adenocarcinoma of the anal sac and lymphoma (Rosol et al., 1992), melanoma (Pressler et al., 2002), thymoma (Foley et al., 2000), and poorly differentiated carcinoma. Increased serum PTHrP concentration has been identified in a small number of cats with humoral hypercalcemia of malignancy associated with a variety of carcinomas and lymphoma (Bolliger et al., 2002). Increased serum PTHrP has also been described in a horse with hypercalcemia and multiple myeloma (Barton et al., 2004). Estimates of the proportion of dogs with thyroid masses that are revealed to be carcinoma range from 51% to 100% (Scarlett, 1994). It is reported that 70% of dogs with thyroid carcinomas have increased concentrations of serum thyroglobulin, although these findings were not strongly correlated with serum thyroxine (T4) concentrations (Verschueren et al., 1991). It is generally accepted that most dogs with thyroid carcinomas are euthyroid, but the contemporary peer-reviewed literature is sparse with respect to the prevalence of functional thyroid tumors in dogs. In cats elevated serum total or free T4 is generally diagnostic for hyperthyroidism, although some cats with nonthyroidal disease may have elevated free T4 (Peterson et al., 2001). Cats with equivocal results may require additional evaluation with the triiodothyronine (T3) suppression test to confirm the diagnosis of hyperthyroidism (Graves and Peterson, 1994). Unlike dogs, most cats with hyperthyroidism have a functional adenoma or adenomatous hyperplasia rather than an underlying thyroid carcinoma. Serial T4 determinations are useful to monitor response to therapy and detect a relapse following radioiodine treatment (Peterson and Becker, 1995). Hyperfunctioning adrenocortical tumors can be diagnosed by measuring basal plasma cortisol concentration or changes in plasma cortisol concentration from a variety of provocative tests using natural or synthetic adrenocorticotropin hormone (ACTH) stimulation or dexamethasone suppression. The measurement of endogenous ACTH may help distinguish primary adrenal disorders from those secondary to pituitary gland dysfunction. The ratio of the precursor hormones pro-opiomelanocortin and proadrenocorticotropin has been recently correlated to the size of pituitary tumors in dogs with hyperadrenocorticism (Granger et al., 2005). Measurement of basal ACTH and the use of provocative testing with ACTH have been reported for the diagnosis of equine pituitary pars intermedia adenomas (van der Kolk et al., 1995). Measurement of plasma sex steroid hormones is useful for characterization of equine GTCT in mares with clinical signs attributable to high plasma concentrations of testosterone or estrogen (Meinecke and Gips, 1987; Stabenfeldt et al., 1979). Occasionally plasma progestins are elevated. Considerable variation in sex steroid hormone profiles exists among affected horses and may be due to the presence of abnormal ovarian follicles, defects in aromatase activity, or possible feedback inhibition affecting gonadotropin secretion (Hoque et al., 2003). Determination of plasma concentrations of androstenedione, 17-hydroxyprogesterone, and estradiol may be useful in diagnosing adrenocortical neoplasia in ferrets (Rosenthal and Peterson, 1996). Likewise, increased plasma concentrations of these adrenal sex hormones have been observed in dogs with adrenal gland adenocarcinoma with provocative testing using ACTH (Hill et al., 2005). Elevated plasma progesterone has been described in a cat with a well-differentiated adrenocortical adenocarcinoma (Boord and Griffin, 1999). Much literature exists surrounding the presence of estrogen and progesterone receptors in mammary gland tumors of the dog. Recent findings reveal that dogs with biologically aggressive inflammatory mammary carcinomas had increased serum and tissue concentrations of androgens when compared to dogs with noninflammatory malignant mammary tumors (Illera et al., 2006). The presence of progesterone receptors has been described in lymphomas of the horse (Henson et al., 2000). A case report describing the regression of a cutaneous lymphoma in a mare after removal of GTCT hints at the biological significance of progesterone receptors in equine lymphomas (Henson et al., 1998). Increased secretion of insulin from the beta cells of the pancreas is the hallmark of insulinoma (beta cell tumor, pancreatic islet cell tumor). Although several variations on the insulin-glucose ratio appear in the literature, documentation of the abnormal physiological condition of fasting hypoglycemia and measurable serum insulin is sufficient for the diagnosis of insulinoma. Measuring serum fructosamine and glycated hemoglobin may also be helpful in the diagnosis of insulinoma. In a few small series of dogs with insulinoma, decreased serum fructosamine concentrations (Loste et al., 2001; Mellanby and Herrtage, 2002) and decreased serum glycated hemoglobin concentrations (Elliott et al., 1997; Marca et al., 2000) have been observed. Low serum fructosamine may indicate the presence of insulinoma despite normoglycemia (Mellanby and Herrtage, 2002). Lactate dehydrogenase (LDH), an enzyme that catalyzes the interconversion of pyruvate and lactate, has been studied in humans with a variety of malignancies, but it is best validated as a marker for evaluating prognosis in patients with nonseminomatous germ cell tumors (Duffy, 2004). LDH activity, along with activity of several of its isoenzymes, is increased in dogs with lymphoma; dogs with lower serum LDH activity had overall longer survival times compared to dogs with increased LDH activity, suggesting this may be a useful marker for canine lymphoma (Zanatta et al., 2003). Increased plasma and urine LDH activity have been observed in cows with urinary bladder cancer (Dawra et al., 1991). Elevated serum alkaline phosphatase (ALP) activity is a prognostic factor in humans with a variety of advanced cancers (Hauser et al., 2006) and may predict outcome in a variety of cancers including prostate cancer (Cho et al., 2003) and advanced uveal melanoma (Eskelin et al., 2003). Serum ALP activity has apparently no value as a tumor marker for canine lymphoma or canine mammary tumors (Karayannopoulou et al., 2003; Wiedemann et al., 2005). Pretreatment elevated serum ALP activity and failure to return to normal ALP activity after treatment correlate with poor survival of dogs with appendicular osteosarcoma (Ehrhart et al., 1998; Garzotto et al., 2000). Prostatic acid phosphatase (PAP), a neutral protein tyrosine phosphatase, was widely used as a tumor marker before the discovery of prostate-specific antigen. Serum PAP activity is increased in men with prostate cancer and has important cellular functions in carcinogenesis of the prostate (Veeramani et al., 2005). However, this increase does not appear to occur in dogs with prostate cancer (Bell et al., 1995). Thymidine kinase (TK), a phosphotransferase enzyme found in most living cells, has been evaluated as a tumor marker in women with breast cancer, showing a decrease in serum activity after surgical treatment of the primary tumor (He et al., 2000). It may also provide prognostic information in humans with non-Hodgkin’s lymphoma and multiple myeloma (Diem et al., 1993; Hallek et al., 1992). In dogs with lymphoma, serum TK activity appears to be a predictor of survival (von Euler et al., 2004). TK can now be measured with nonradiometric methods, making it more appealing for routine laboratory use (von Euler et al., 2006). A long-standing use of a serum tumor marker in veterinary medicine is the measurement of serum immunoglobulins. In diseases of B cells, including multiple myeloma and Waldenström’s macroglobulinemia, hyperglobulinemia is often present. Electrophoresis of the serum proteins reveals a typical monoclonal gammopathy, and immunoelectrophoresis is used to further characterize the antibody class of the immunoglobulins. The hyperglobulinemia resolves with successful treatment and therefore makes a convenient measure to assess response and recurrence. IgM, IgG, and IgA gammopathies have been described in the dog (Giraudel et al., 2002; Lautzenhiser et al., 2003; Ramaiah et al., 2002). It should be noted that hypergammaglobulinemia does not occur with all cases of myeloma, and there may be hypogammaglobulinemia with a normal electrophoretic pattern. Canine ehrlichiosis infection can also cause a monoclonal gammopathy and should be considered among the differential diagnoses for increased serum immunoglobulins (Breitschwerdt et al., 1987). Cancer antigen 125 (CA-125) is a product of the MUC16 gene and has found use in human medicine as a tumor marker for epithelial ovarian cancers, although the sensitivity and specificity are poor (Moss et al., 2005). Serum CA-125 may be elevated in other cancers, such as endometrial cancer, cancer of the fallopian tubes, lung cancer, breast cancer, and cancer of the gastrointestinal tract and nonneoplastic diseases such as endometriosis. CA-125 has not found application in veterinary medicine. Prostate-specific antigen (PSA) is a tumor-associated antigen identifiable in the serum of men with prostate cancer, and it has become a widely used tumor marker for prostate cancer screening programs. Evidence suggests it may not adequately discriminate between cancer and benign enlargement of the prostate gland (Constantinou and Feneley, 2006). One study failed to identify PSA in canine serum, although weak immunoreactivity was noted on immunohistochemistry of canine prostatic adenocarcinoma specimens (Bell et al., 1995). Tumor-associated antigens, 1A10 and SB2, have been identified in the serum of dogs with a variety of cancers with the use of murine monoclonal antibodies developed against a canine mammary carcinoma cell line (Wang et al., 1995). However, the clinical importance of these antibodies has not been defined. Fibronectin (FN) is a large glycoprotein that is a component of the extracellular matrix and occurs in its soluble form in plasma. A study of plasma FN concentrations in dogs with malignancies documented both increased and decreased plasma FN (Feldman et al., 1988). A study of canine and feline pleural and abdominal effusions revealed no useful distinction in FN concentrations between malignant and nonmalignant effusions, although the FN/albumin ratio was higher in dogs with malignant effusions compared to those with congestive heart failure (Hirschberger and Pusch, 1996). Sialic acid describes derivatives of neuraminic acid, which are potentially useful tumor markers because of the aberrant glycosylation in cancer cell membranes. Although the cancer specificity of sialic acid is reported to be high, its sensitivity is low because sialic acid-rich glycoproteins are present in inflammatory diseases (Narayanan, 1994). Increased serum sialic acid concentration has been observed in cancer-bearing dogs (Poli et al., 1986); however, further studies suggest the increase is not specific for cancer (Thougaard et al., 1998). The acute-phase proteins (APP) are those plasma proteins, made primarily by hepatocytes, whose concentrations change after tissue injury or infection. Their relationship with cancer has long been recognized in human medicine (Cooper and Stone, 1979). An increase in APP at the time of diagnosis is a negative prognostic indicator in human cancer patients, and increases may also occur with advancing disease (Cooper, 1988). APP that may have use as tumor markers include ceruloplasmin (Cp), complement components C3 and C4, α1-acid glycoprotein (AGP), α1-proteinase inhibitor (α1-antitrypsin), α1-antichymotrypsin, haptoglobin (Hp), fibrinogen (Fbn), C-reactive protein (CRP), and serum amyloid A (SAA). As an example, increased serum CRP has been shown to be a poor prognostic indicator in people with multiple myeloma, melanoma, lymphoma, and ovarian, renal, pancreatic, and gastrointestinal tumors (Mahmoud and Rivera, 2002). Because interleukin 6 (Il-6) and tumor necrosis factor alpha (TNF-α) induce synthesis of CRP and potentially other APP, they are also receiving attention as potential biomarkers and therapeutic targets. APP have been studied in veterinary medicine. In a recent study of APP in diseased dogs, significant increases in Cp, Hp, and CRP were observed in dogs with cancer and other inflammatory diseases, and decreases with treatment were associated with more favorable outcomes (Tecles et al., 2005). Increased serum concentrations of AGP at diagnosis have been observed to decrease with cancer treatment and onset of clinical remission in dogs with lymphoma, suggesting its potential as a biomarker (Hahn et al., 1999). The flow cytometer is frequently employed to determine the DNA content of cancer cells in various veterinary tumors (Culmsee and Nolte, 2002). Most normal tissues, reactive tissues, and benign tumors have diploid (2N) DNA values. The different DNA content of tumor cells compared to normal tissues may be the result of abnormal DNA (aneuploidy) or due to increased DNA content with cell division. Although aneuploidy has been identified in a wide variety of veterinary tumors, it has not been studied carefully as a prognostic indicator. Aneuploidy may predict aggressive tumor behavior in canine hemangiopericytoma (Kang et al., 2006), canine mammary tumors (Hellmen et al., 1993), canine melanoma (Bolon et al., 1990), and possibly canine mast cell tumors (Ayl et al., 1992). However, aneuploidy does not seem to predict biological behavior or prognosis in equine melanomas (Roels et al., 2000a) or canine lymphomas (Teske et al., 1993). With the use of specific monoclonal antibodies, the flow cytometer can be used to characterize cell populations based on their cell surface determinants. Although particularly well suited for the study of leukemias, this technique may also be adapted to the study of solid tumors. Aberrant expression of cluster differentiation (CD) molecules has been identified as a common, and distinguishing, feature of canine lymphomas (Wilkerson et al., 2005). The flow cytometer also allows rapid immunophenotyping of lymphomas using an aspirate specimen (Culmsee et al., 2001). Further work is needed to determine how these findings may correlate with prognosis. Determining the frequency of mitotic figures visible in histological sections of biopsy specimens is a long-standing method for assessing cell proliferation. Cells in the mitotic phase are easily recognizable on routinely prepared specimens. There are various methods for reporting the mitotic activity, including number of mitoses visible per certain number of high, dry microscopic fields or the number of mitoses present in a certain number of cells (mitotic index). It has been shown that the mitotic index correlates better to other indices of proliferation and tumor grade compared to mitoses per area (Sarli et al., 1999). Although determining mitotic counts is technically simple, it is limited in its usefulness because of lack of reproducibility, and errors in interpretation may be introduced by delays in tissue fixation, variation in section thickness or size of microscopic field of view, and in difficulty recognizing mitoses. High mitotic index has been reported as a negative prognostic indicator of various tumors, including canine ocular melanoma (Wilcock and Peiffer, 1986), feline fibrosarcoma (Bostock and Dye, 1979), and canine soft tissue sarcomas (Kuntz et al., 1997). However, the mitotic index may have limited value for predicting the behavior of canine melanoma (Spangler and Kass, 2006), canine skin cancers (Martin De Las Mulas et al., 1999), or canine lymphoma (Kiupel et al., 1999). The synthesis of DNA occurring in the S phase of the cell cycle can be measured by labeled DNA precursor incorporation. Although there are radiometric methods using tritiated thymidine, bromodeoxyuridine (BrdU), a halogenated thymidine analogue, is more commonly used for direct estimation of DNA synthesis. The use of specific monoclonal antibodies to BrdU allows immunohistochemical detection in paraffin-embedded sections with simultaneous morphological examination of the tissues. The thymidine labeling index is defined as the ratio of the number of positively stained cells to the total number of cells. The S-phase fraction of the cell cycle can be determined by counting nuclei labeled with BrdU providing an accurate assessment of the proliferative capacity of the tumor. One drawback to this technique is that it requires infusing patients with BrdU before surgery to permit its incorporation into tumor DNA. BrdU incorporation and calculation of tumor potential doubling time has been associated with biological behavior and prognosis in canine chondrosarcoma, osteosarcoma, epulides, and lymphoma (Ohta et al., 2004; Schwyn et al., 1998; Vail et al., 1996; Yoshida et al., 1999). Quantifying the proteins associated with nucleolar organizing regions (NORs) of interphase chromosomes is another method to assess the proliferation rates of tumors. The NORs are visualized microscopically with a simple silver-staining method that is effective because of the argyrophilia of their nonhistone acidic proteins. The staining procedure can be done on both histological and cytological specimens. These argyrophilic nucleolar organizing regions (AgNORs) are representative of actual or potential transcriptional activity of ribosomal DNA and may be used as a marker for cell proliferation. AgNOR counts may be expressed as the mean number of AgNORs per nuclei or as the percentage of tumor cells with >5 AgNORs per nucleus. It is believed that the mean AgNOR count reflects DNA ploidy, whereas the percentage of tumor cells with >5 AgNORs per nucleus represents the proliferative activity. Increasing AgNOR scores have been shown to be predictive of poor outcome in canine soft tissue sarcoma (Ettinger et al., 2006), canine mast cell tumors (Bostock et al., 1989; Scase et al., 2006; Simoes et al., 1994), canine mammary gland tumors (Sarli et al., 2002), canine lymphoma (Kiupel et al., 1998, 1999; Vail et al., 1996), transmissible venereal tumors (Harmelin et al., 1995), and feline mammary carcinoma (Preziosi et al., 2002). However, AgNOR scores do not seem to be predictive of outcome in feline lymphoma (Rassnick et al., 1999; Vail et al., 1998), and other studies suggest that AgNOR scores do not predict outcome in canine mammary gland tumors (Lohr et al., 1997). Methods for detection of cell cycle-related antigens are described in Section V.D. Obtaining morphometric and densitometric measurements from individual nuclei and providing information about ploidy, S-phase fraction, and nuclear area, are possible with image analysis of cytocentrifuge preparations of Feulgen-stained nuclei extracted from formalin-fixed, paraffin-embedded tissues. Morphometric measurements can also be obtained from hematoxylin and eosin (H&E) and panoptic-stained cytology specimens. Nuclear morphometry and ploidy may aid in the classification of canine mast cell tumors (Strefezzi Rde et al., 2003), distinguish benign from malignant melanocytic tumors in dogs and cats (Roels et al., 2000b), and predict the outcome of feline mammary carcinomas (De Vico and Maiolino, 1997). Apoptosis is an energy-dependent process that may be considered a “programmed” form of cell death, distinct from the “accidental” cell death of necrosis. During apoptosis, there is activation of endogenous nucleases, which create oligonucleosomal fragments that have a characteristic orderly ladder appearance when separated by electrophoresis, compared with the smeared appearance of DNA degraded during necrosis. Cells undergoing apoptosis have characteristic morphological features including condensation of nuclear heterochromatin and resultant crescent apposed to the nuclear membrane, cell shrinkage, cytoplasmic condensation, and bud formation at the cell membrane, which may condense into “apoptotic bodies.” Another characteristic of apoptosis is the absence of inflammation, which is typically present with necrosis. Although features of apoptosis may be identified with light microscopy of H&E-stained cells, electron microscopy or fluorescence microscopy with dyes such as propidium iodine or acridine orange may also be useful. The apoptotic index predicts the initial relapse-free survival in dogs with lymphoma, but not its overall survival (Phillips et al., 2000). However, the apoptotic index does not appear to correlate with survival in feline mammary tumors (Sarli et al., 2003).
I. INTRODUCTION
II. SERUM TUMOR MARKERS
A. Oncofetal Proteins
B. Hormones and Ectopic Hormones
1. Inhibin
2. Serum Parathyroid Hormone
3. Parathyroid Hormone-Related Protein
4. Thyroxine/Thyroglobulin
5. Adrenocorticotropin Hormone/Cortisol
6. Sex Steroid Hormones
7. Insulin
C. Enzymes
1. Lactate Dehydrogenase, Serum Alkaline Phosphatase, Prostatic Acid Phosphatase, Thymidine Kinase
D. Immunoglobulins
E. Tumor-Associated Antigens
1. CA-125
2. Prostate-Specific Antigen
3. Other Tumor-Associated Antigens
F. Miscellaneous Serum Tumor Markers
1. Fibronectin
2. Sialic Acid
3. Acute-Phase Proteins
III. FLOW CYTOMETRY
A. DNA Ploidy
B. Cell Surface Determinants
IV. PROLIFERATION MARKERS/APOPTOSIS
A. Mitotic Counts
B. Thymidine-Labeling Index and BrdU Incorporation
C. Nucleolar Organizing Regions
D. Proliferation Markers
E. Image Analysis
F. Apoptosis
V. IMMUNOHISTOCHEMISTRY/IMMUNOCYTOCHEMISTRY
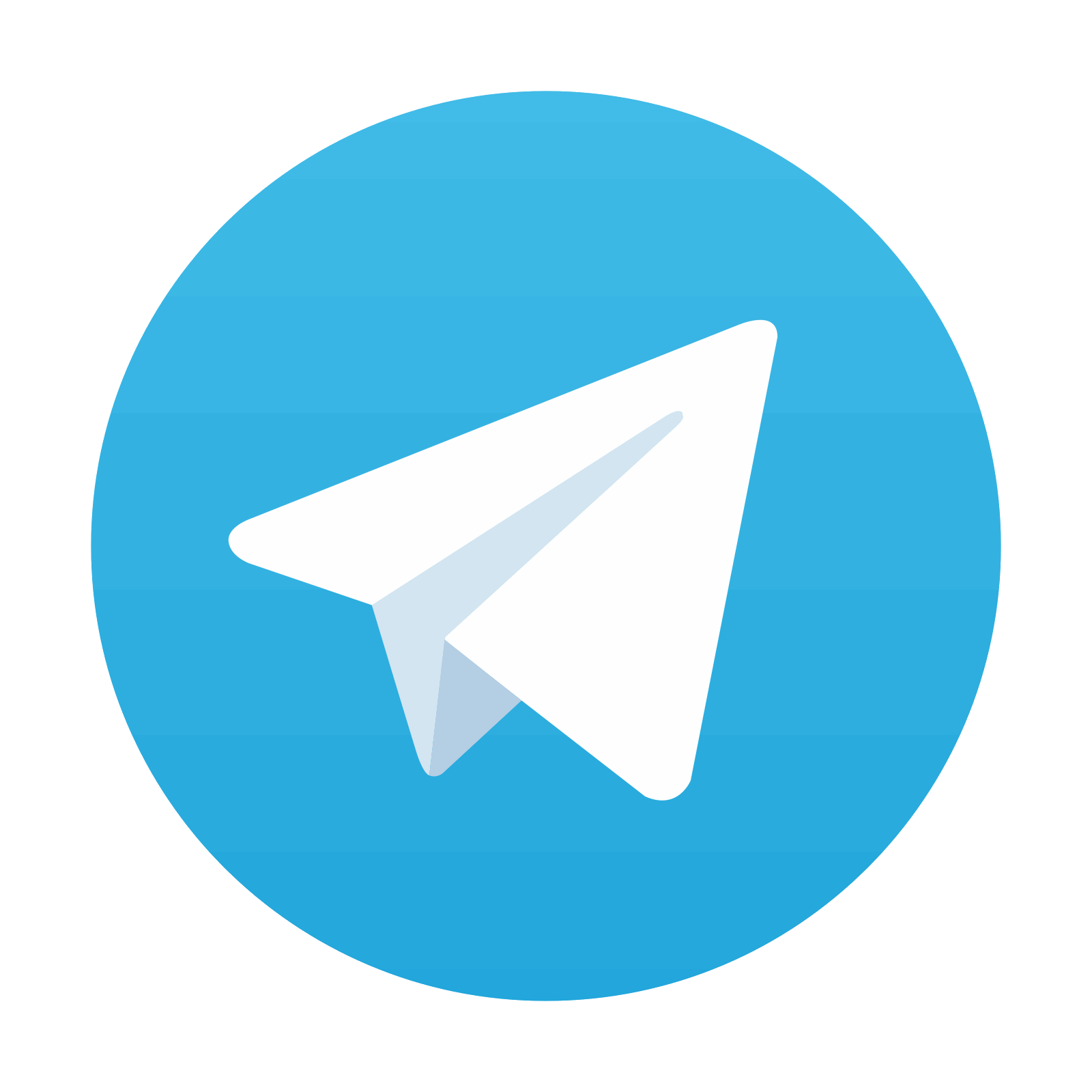
Stay updated, free articles. Join our Telegram channel

Full access? Get Clinical Tree
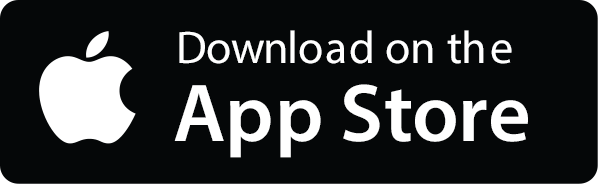
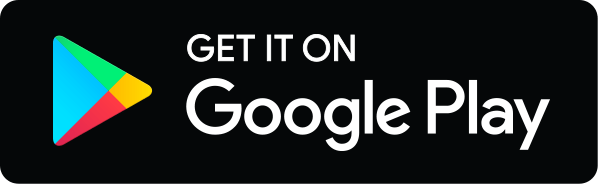