Chapter 20 Thyroid Function V. FUNCTIONS OF THE THYROID GLAND VI. TRANSPORT OF HORMONE: PROTEIN BINDING VII. MECHANISM OF THYROID HORMONE ACTION A. General Effects of Thyroid Hormones B. Molecular Basis of Thyroid Hormone Action VIII. CATABOLISM AND EXCRETION OF THYROID HORMONES IX. REGULATION OF THE THYROID GLAND A. Production and Regulation of Thyroid-Stimulating Hormone B. Action of the Thyroid-Stimulating Hormone on the Thyroid Gland C. Action of the Thyroid-Stimulating Hormone on Hormonogenesis D. Long-Acting Thyroid Stimulators A. Indirect Tests of Thyroid Function B. Direct Tests of Thyroid Function E. Trophic Hormone Response Tests D. Tumors of the Thyroid Gland Disorders of the thyroid gland are the most common endocrine disorders in humans, and extensive historical and scientific literature is available. Among the domestic animals, thyroid function and its diseases are well known in companion animals but less so in other animals, domestic or wild. In livestock, nutritional iodine deficiencies have been of greater importance than thyroid gland diseases, particularly in the iodine-deficient areas of the world. The importance of thyroid function and its diseases has also become progressively more important as the longevity of companion animals such as the dog and the cat has increased. Advances in thyroid physiology, pathogenesis of its diseases, and the continued development and refinement of methods of testing thyroid function have added impetus to the study of thyroid disease in all animals. This chapter reviews the anatomy and physiology of the thyroid gland and its diseases as a corollary to the understanding of the pathophysiology of the thyroid gland in disease. Emphasis is placed on the physiological bases of a variety of thyroid function tests, most of which are now readily available to the veterinary clinician. The thyroid gland of animals is a bilobed structure that overlays the trachea at a point just below the larynx. Anatomical variations of the gland are marked between species and, to some extent, within a given species. The isthmus connecting the two lobes of the thyroid is the region that varies most markedly between species. Humans and pig have a large discrete isthmus, which forms a pyramidal lobe connecting the two lateral lobes. The cow has a fairly wide band of glandular tissue, which forms the connecting isthmus. In the horse, sheep, goat, cat, and dog, the isthmus is a narrow remnant of tissue and may be nonexistent. The size of the gland relative to body weight is extremely small in all animals, approximating 0.20% of body weight. The size of the gland varies and may be enlarged when iodine deficiency, ingestion of goitrogenic toxins, tumors, or hyperactivity is present. In primary hypothyroidism, the gland may be reduced to fibrotic and inactive remnants of thyroid tissue. Accessory or extrathyroidal tissue is commonly observed in the dog, particularly near the thoracic inlet though they may be found anywhere along the esophagus. This accessory tissue is fully functional physiologically, synthesizes hormone, and can be located by its uptake of radionuclides. This is a particularly important consideration for the dog when thinking about surgical intervention for treatment of hyperthyroidism resulting from a thyroid tumor. The thyroid gland is a highly vascularized tissue with a large blood flow. The functional unit of the thyroid gland is the thyroid follicle, which can be envisioned as a spherical structure composed of an outer monolayer of follicular cells surrounding an inner core of colloid. Colloid is a thyroglobulin-hormone complex that is the storage reservoir of thyroid hormone in the thyroid gland. The colloid stored in the lumen is a clear, viscus fluid. The individual follicular cells vary from 5 to 10 μm in height, and the entire follicle may vary from 25 to 250 μm in diameter. The size of the follicles and the height of their follicular cells vary according to the functional state of the gland. The cells may vary from an inactive squamous cell to the highly active, tall columnar cell. Interspersed between the follicles are the thyroid “C” cells, the source of calcitonin, the hypocalcemic hormone associated with calcium metabolism. A third type of hormonal tissue, the parathyroid, is imbedded within the thyroid or located in close proximity to it. The parathyroids are the source of parathormone, the hypercalcemic hormone. Removal of the parathyroids is virtually unavoidable during surgical thyroidectomies so that post-surgical hypocalcemias are important consequences to be considered. The thyroid gland is unique among the endocrine glands in that an integral part of its hormone, L-thyroxine (T4), is a trace mineral, iodine, which is available to the animal in only limited amounts. Marine plants are known to be good sources of iodine, but on land, iodine is limited and many regions of the world are known to be iodine deficient. The recommended daily requirement is 35 μg/kg bw (276 nmol/kg bw) for the adult dog and 70 μg/kg bw (551.6 nmol/kg bw) for the growing puppy. For most animals, nutrient requirements are given in mg/Kg dry diet or ppm and range from 0.1 to 1.0 mg, including cats and horses. Milk is a poor source of hormonal iodide contributing only about 4% to 7% of the maintenance requirements for hormone (Akasha and Anderson, 1984). The small requirements are compensated for by an efficient intestinal absorption mechanism and conservation and recycling of internal iodine. Little iodine is lost from the body by the various excretory routes such as urine, saliva, tears, milk, sweat, and feces. Also, whereas most endocrine glands store little of their hormones, the thyroid manages to store large quantities of hormone sufficient for 1 to 3 weeks depending on the species. The thyroid gland contains about 20% of the total body iodine. Its iodine content and size vary with iodine intake and the state of thyroid function, but it usually contains 10 to 40 mg (78.8 to 315.2 μmol) iodine/100 gm tissue or 4 to 16 mg (31.5 to 126.1 μmol) iodine in a 20-kg dog. Iodine can be absorbed in any of its soluble chemical forms, but in the intestines it is usually absorbed in the form of iodides (I–), iodates (IO4–), or as the hormonal forms. Iodides may be absorbed from any moist body surface, including the mucus membranes, and it is absorbed easily through broken epithelia. Normally, the chief route of entry into the general circulation is by absorption through the mucosal cells of the small intestine. The I– in the circulation is trapped almost exclusively by the thyroid gland, with small amounts being trapped by the salivary gland and minimal amounts by the gastric mucosa, placenta, and mammary gland. In the ruminant, 70% to 80% of an oral dose is absorbed in the rumen and 10% in the omasum. The main route of excretion of I– is by the kidneys through which almost all the I– that was not trapped by the thyroid is lost in the urine. A small but significant amount is lost in the saliva, and minimal amounts are lost in tears, feces, sweat, and milk. A minute amount of free hormone, that fraction not bound to serum proteins, is also lost in the urine. These routes of excretion are especially important considerations when patients are being treated with radioiodine. The main functions of the thyroid gland are the trapping of I– and the synthesis, storage, and release of thyroid hormones (Fig. 20-1), and these activities are under the control of the thyrotropin or the thyroid-stimulating hormone (TSH). Although TSH stimulates all steps in hormonogenesis, the trapping of I– and the release of hormone are the two major sites of its action. FIGURE 20-1 Pathways of iodine metabolism and thyroid hormone synthesis. Goitrogenic blocking agents are shown in brackets. The principal thyroid hormones elaborated by the thyroid are the two active hormones, 3,5,39,59-tetraiodothyronine (T4) and 3,5,39-triiodothyronine (T3), and the inactive hormone, 3,39,59-triiodothyronine (reverse T3 or rT3). The rT3 is the inner deiodination product of the T4. The structures of the individual hormones are given in Figure 20-2. The T4 molecule contains 65.3% iodine, and the T3 molecule contains 58.5% iodine. The T3 is the active hormone in the target cell. The T4 functions as the transport form and as the feedback regulator of the thyroid gland. The I–in the general circulation is taken up by the thyroid follicular cells by a highly efficient trapping and concentrating mechanism. It does this against a large concentration gradient, which can be from 1:20- to 1:500-fold across the thyroid cell membrane and is stimulated by TSH. The trapping process is catalyzed by a trapping enzyme, requires oxygen, and is an active transport or “pump” mechanism catalyzed by a Na+K+-ATPase and dependent on ATP. The transport process is mediated by a sodium iodide symporter (NIS) protein located on the plasma membrane (Riesco-Eizaguirre and Santisteban, 2006). It is the high efficiency of this trapping system that concentrates virtually all of the body iodine in the thyroid gland. It also accounts for the microgram nutrient requirement for iodine. In addition, the thyroid follicular cells have a high capability for compensatory hypertrophy when there is a scarcity of iodine, hence the development of iodine deficiency goiters. The efficiency of I– trapping is also the basis for the radioactive iodine uptake (131I Uptake) test of thyroid function, 131I thyroid imaging, and 131I thyroid therapy. The trapping of I– is stimulated by TSH and blocked by the goitrogenic agents such as thiocyanate (SCN-), perchlorate ( FIGURE 20-2 Chemical structures of the major iodinated compounds of the thyroid gland. After trapping, there is an oxidation of I–catalyzed by a peroxidase and the product is a highly active form of iodine, most likely a free radical, I*. This reaction is inhibited by thyrotoxic agents such as the thiouracils or thioureas and stimulated by TSH. Propylthiouracil is commonly used in the treatment of hyperthyroidism. The I* almost instantaneously binds to the phenyl groups of the tyrosine moieties of thyroglobulin at the 3 or 5 position to form a monoiodotyrosine (MIT) or a diiodotyrosine (DIT). This iodination occurs while the tyrosines remain in polypeptide linkage within the thyroglobulin molecule. Next, the iodinated phenyl groups of the tyrosines are coupled by the oxidative condensation of an iodinated phenyl group of one DIT to another DIT to form T4 or of an MIT group to a DIT to form T3. These iodination reactions occur mainly at the follicular cell membrane-colloid interface. These iodination reactions are energy requiring and sensitive to blocking by sulfa drugs, thioureas, and paraaminobenzoic acid (PABA). Thyroglobulin is the thyroidal glycoprotein of high molecular weight (660,000 daltons) synthesized exclusively by the thyroidal follicular cells. After synthesis, it moves to the cell membrane where the iodinations occur and the iodinated thyroglobulin, or colloid, is released into the lumen of the follicle where it is stored. The amount stored can be quite large as evidenced by the mass of protein contained within a normal follicle. TSH stimulation of the release of hormones is the second of its two principal sites of action. Within a few minutes after giving TSH, small packets of colloid are taken up by the follicular cell membrane, and vesicles are formed and taken into the follicular cell by endocytosis. The vesicles merge with lysosomes within the cell, and the lysosomal proteases hydrolyze the colloid and release their MIT, DIT, T3, and T4. The released MIT and DIT are enzymatically degraded by microsomal tyrosine deiodinases, and their iodine is recycled within the follicular cell. The T4 and T3 are released into the circulation by a simple diffusion process. Of the total hormone released, about 90% is T4 and 10% is T3. Within the gland, there is also some deiodination of the T4 of the inner phenyl group to form rT3, but most of this deiodination occurs in the peripheral tissues. This rT3 is the inactive form of thyroid hormone and is on a degradation pathway. The thyroid hormones in the circulation are T4, T3, and rT3. Immediately upon entering the circulation, these hormones are bound to transport proteins, mainly to thyroxine-binding globulin (TBG), and with lesser amounts to thyroxine binding prealbumin (TBPA) and to albumin. There is a wide spectrum of species variation in hormone binding by serum proteins (Table 20-1). TBPA is present in all the species in contrast to earlier reports indicating that TBPA was present only in humans, rhesus monkey, horse, cat, rabbit, pigeon, and chicken. The early work was based on electrophoretic migration, but later work based on chemical properties identified TBPA or its analogues at different migration sites (Larsson et al., 1985). The differences in migration are likely due to differences in the amino acid composition of these proteins among the various species. Using chemical criteria, all species have TBPA. TABLE 20-1 Thyroxine-Binding Proteins of Various Species, Their Electrophoretic Migration, and Their Relative Thyroxine Bindinga a TBG, thyroxine-binding globulin; ALB, albumin; TBPA, thyroxine-binding prealbumin; α-G, α-globulin: positioned as shown relative to the albumin position in each species. Numbers in parentheses specify the percentage of thyroxine binding. Constructed from the data of Tanabe et al. (1969), Refetoff et al. (1970), and Larsson et al. (1985). The * indicates approximations. In the cat, rabbit, rat, mouse, guinea pig, pigeon, or chicken, TBG is absent and most of the hormone is transported by albumin. The albumin binding constant is about 105 liter/mole for T4 or T3 but with an unlimited binding capacity. In these species without TBG, albumin transports between 50% and 80% of the hormones. T3 (and likely rT3) appears to bind to these transport proteins in parallel with T4 binding. Protein binding has several functions. Protein binding solubilizes these lipid soluble hormones for transport in the aqueous plasma. The bound forms also do not readily pass through the renal glomerular membrane, so they minimize urinary loss of hormones, whereas the free hormones pass and are lost. The bound forms also serve as a large and readily accessible reservoir of the active hormones for delivery to the target organs and cells. Finally, the protein binding equilibrium is the fundamental basis for protein or immunoprotein binding assays of hormone and the indirect assay of TBG. After the administration of thyroid extracts or T4, its first physiological effects are noted in 24 to 28 h and its maximal effects are noted in 7 to 10 days (Table 20-2). The T4 requirements for these effects vary. The requirement for T3 also varies, and less is required for equivalent activity and it acts more quickly. T3 is now recognized as the active form of the thyroid hormone within the target cell. The T4 that is transported into the target cell is rapidly deiodinated to the active T3. However, rT3, which is also produced in the cell in the deiodination process, is an inactive form of thyroid hormone and is simply degraded. The molecular basis of thyroid hormone action at the cellular level has been frequently reviewed, and a multifaceted concept of its action has evolved. For many years, the mitochondrion was considered to be the site of thyroid hormone action. Uncoupling of oxidative phosphorylation (ox-phos) in the mitochondria was a viable hypothesis for many years. Under normal conditions, 3 moles of ATP (P) are synthesized per atom of oxygen (½ 02 = 0) used in the cytochrome oxidase system; hence, P:0 ratio equals 3. If less than 3 moles of ATP are formed per unit 0 in a system in the presence of a compound such as the thyroid hormone, the system is said to be uncoupled (i.e., P:0 ratio is less than 3). In this event, more O2 would be needed to generate an equivalent amount of ATP, and O2 consumption would increase. T4 has repeatedly been shown to uncouple oxidative phosphorylation in in vitro systems, but it does so only in large, unphysiological amounts. These findings were extended to the whole animal to explain the increased oxygen consumption by T4. The large amounts of oxidative energy not incorporated into ATP increased body temperature and were dissipated as heat. Thus, T4 action was theorized to be the result of the uncoupling of oxidative phosphorylation. Another now well-known effect of thyroid hormone is the stimulation of cellular protein synthesis (Tapley, 1964; Tata et al., 1963), and this occurs during the latent period when the calorigenic effect of thyroid hormone occurs. T3 is now known to stimulate messenger RNA (mRNA) transcription, increasing translation and protein synthesis and accounting for the anabolic effects of thyroid hormones. This also means that the site of action is at the cell nucleus. Another action of thyroid hormone is to stimulate the “sodium pump” (Na-K-ATPase) at the cell membrane, an action that would increase O2 consumption (Edelman, 1974). Ouabain, an inhibitor of Na-K-ATPase, also inhibits the increased O2 consumption induced by T4 or T3. Thus, stimulation of the sodium pump is an important way in which thyroid hormones stimulate increased oxygen consumption and accounts for almost half of the increase.
I. INTRODUCTION
II. ANATOMICAL CONSIDERATIONS
III. NUTRITIONAL REQUIREMENTS
IV. IODINE METABOLISM
V. FUNCTIONS OF THE THYROID GLAND
A. Thyroid Hormones
B. Hormonogenesis and Release
1. Trapping of Iodide
) and by large amounts of I–. Figure 20-1 also shows the sites of these and other blocks in the thyroxine biosynthetic pathway. Other compounds are trapped by the thyroid gland, and the most widely clinically used one is 99mTc-pertechnetate, which is used for thyroid tissue imaging.
2. Synthesis of Thyroid Hormones
3. Storage of Hormone
4. Release of Hormone
VI. TRANSPORT OF HORMONE: PROTEIN BINDING
VII. MECHANISM OF THYROID HORMONE ACTION
A. General Effects of Thyroid Hormones
B. Molecular Basis of Thyroid Hormone Action
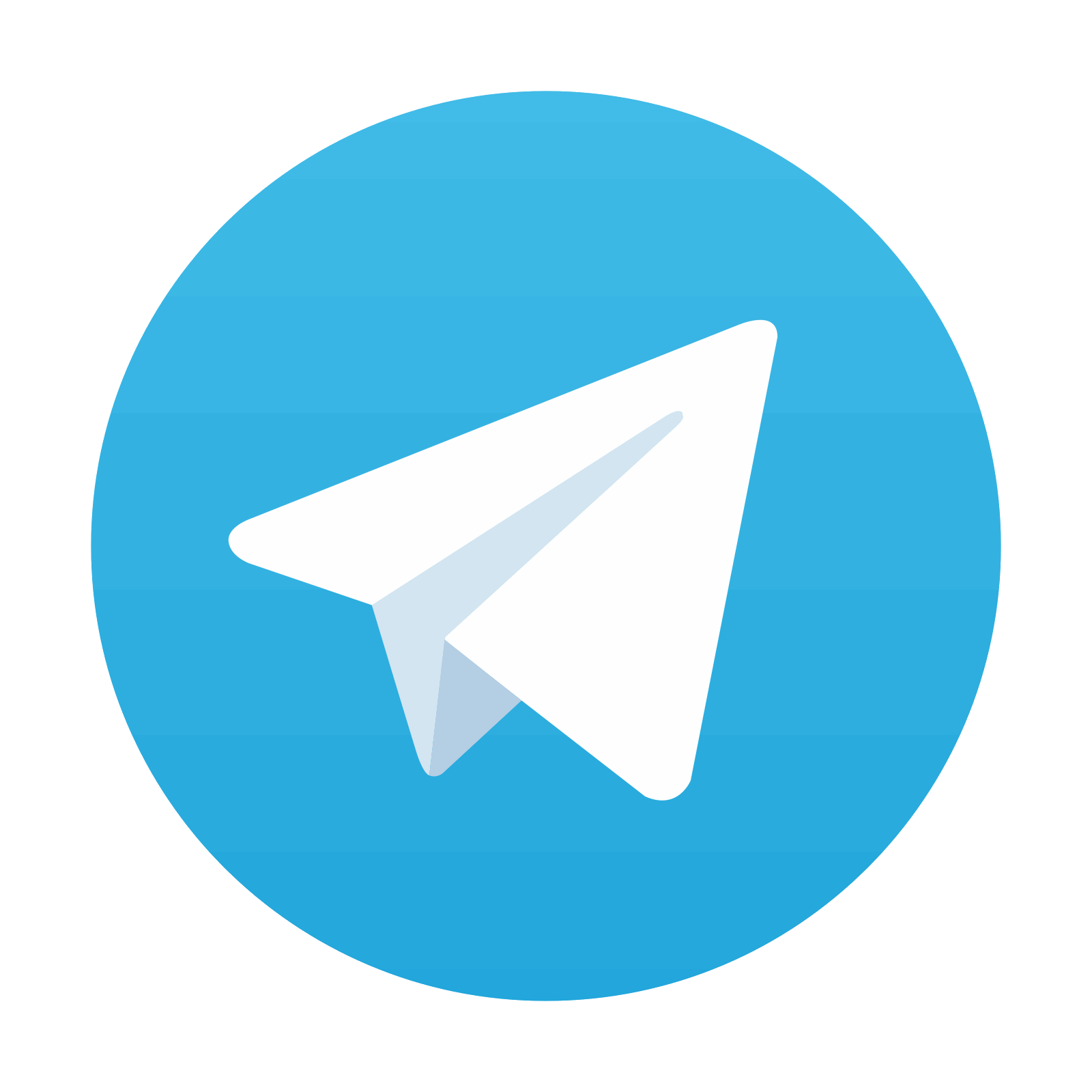
Stay updated, free articles. Join our Telegram channel

Full access? Get Clinical Tree
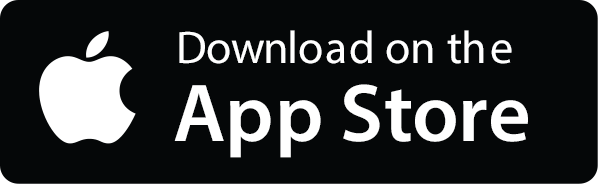
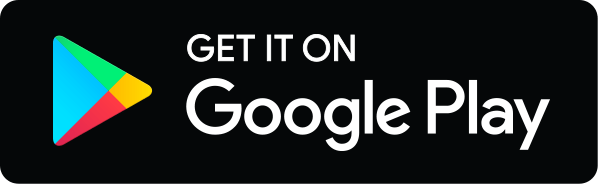