CHAPTER 16 Musculo-skeletal system
The musculoskeletal system consists of bones, cartilage, muscles, ligaments, and tendons. The main functions of the musculoskeletal system are support of the body, provision of motion, and protection of vital organs, especially the brain and thoracic and abdominal viscera. The skeletal system also serves as the main storage system for calcium and phosphorus.
THE DEVELOPMENT OF BONES (OSTEOGENESIS)
Despite this diversity, there are some fundamental embryological commonalities in the development of the skeleton. Skeletal tissue arises from cells with a mesenchymal morphology, but the origins of the mesenchyme vary in different regions of the body. In the trunk the mesenchyme that gives rise to the segmented axial skeleton (vertebral column, ribs and sternum) is derived from the sclerotomal portion of the somites (Figs 16-1, 16-2). The appendicular skeleton (the bones of the limbs and their respective girdles) originates from the mesenchyme of the lateral plate mesoderm. The origins of the head skeleton are more complex. Some cranial bones (e.g. those making up the roof and much of the base of the skull) are mesodermal in origin, but the facial bones and some of the bones covering the brain arise from mesenchyme derived from the ectodermal neural crest.
Intramembranous bone formation
Direct conversion of mesenchymal cells into bone is called intramembranous (or desmal ossification; Fig. 16-3). In the skull, neural-crest-derived mesenchymal cells proliferate and condense into compact aggregates. The condensation phase of bone formation is typically accompanied by the up-regulation of N-cadherin and N-CAM, molecules that mediate adhesion of the bone-forming cells and promote the establishment of a preskeletal condensation. Some of the mesenchymal cells change their shape to become osteoblasts that secrete osteoid, an extracellular matrix consisting of collagen and proteoglycans, which is able to bind calcium. Intramembranous bone formation involves bone morphogenetic proteins (especially BMP-2, BMP-4, and BMP-7) from the head epidermis that instruct the neural-crest-derived mesenchymal cells to become osteoblasts and to express a transcription factor CBFA1 (core binding factor 1), also called Runx2. CFBA1 activates the genes for osteocalcin, osteopontin and other bone-specific extracellular matrix proteins.
Endochondral bone formation
In endochondral ossification the mesenchymal cells first differentiate into cartilage, which is later replaced by bone tissue (Fig. 16-4). Endochondral ossification is seen predominantly in the vertebral column, ribs, pelvis and limbs.
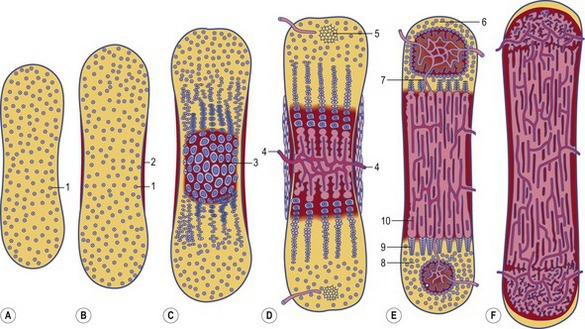
Fig. 16-4: Formation of a long bone on a model made by cartilage. A: Initially, a cartilage model (1) of the respective bone is formed. B: Around its middle part, a hollow bone cylinder is formed, the bone collar (2), which is produced by intramembranous ossification within the local perichondrium; 1: Hyaline cartilage; C: In the middle part of the cartilage model, the chondrocytes undergo a degenerative process with cell enlargement (hypertrophy) and matrix calcification (3), resulting in a 3-dimensional structure formed by the remnants of the calcified matrix. D: In the diaphysis of the cartilage model, blood vessels penetrate the bone collar (4), bringing osteoblasts and chondroclasts to this region. The chondroclasts degrade the calcified matrix and the osteoblasts produce a continuous layer of primary bone around the cartilaginous matrix remnants. In this way, the primary ossification centre is established. Secondary ossification centres (5) appear at the end of the cartilage model, the epiphyses. E: In the two epiphyses, cartilage remains in two regions: the articular cartilage (6), which persists through adult life, and the epiphyseal cartilage (7), which connects each epiphysis to the diaphysis. Histologically, the epiphyseal cartilage can be divided in 5 zones starting from the epiphyseal side of the cartilage:
Endochondral ossification of a long bone consists of a characteristic sequence of events that are more fully described in histology textbooks. Here, we want to give only a short overview. Initially, a cartilage model of the bone is formed. Then, around its middle part, a hollow bone cylinder is formed, the bone collar, which is produced by intramembranous ossification within the local perichondrium. In this part of the cartilage model, the chondrocytes undergo a degenerative process with cell enlargement (hypertrophy) and matrix calcification, resulting in a 3-dimensional structure formed by the remnants of the calcified matrix. This process starts in the middle part (diaphysis) of the cartilage model, where blood vessels penetrate the bone collar, bringing osteoblasts and chondroclasts to the region. The chondroclasts degrade the calcified matrix and the osteoblasts produce a continuous layer of primary bone around the cartilaginous matrix remnants. In this way, the primary ossification centre is established. Second ossification centres appear at the end of the cartilage model, the epiphyses. During the expansion and remodelling of the ossifying bone, the primary and secondary ossification centres produce cavities that are gradually filled with bone marrow.
Within the epiphyseal cartilage, the proliferation of chondrocytes continues until puberty. It is therefore responsible for the growth in length of the bone. The increase in sex hormone concentration at puberty causes the disappearance of the epiphyseal cartilage and the closure of the epiphyses.
AXIAL SKELETON
Vertebral column and ribs
Before the sclerotome cells form a vertebra, they split into two populations which fill the cranial and caudal segments of a presumptive vertebra. Due largely to differential growth rates, the caudal cells of each sclerotome become contiguous with the cranial population of the adjacent sclerotome. According to this traditional view of vertebral development, the densely packed caudal half of one sclerotome joins with the loosely packed cranial half of the next to form the centrum (body) of a vertebra (Fig. 16-5). This process is called resegmentation and explains why spinal ganglia and ventral roots of the spinal nerves are positioned between vertebrae, and the originally intersomitic arteries subsequently run between the pedicles of the vertebral arch. Due to resegmentation, each myotome then spans from one vertebra to another and bridges the intervertebral disc. This alteration gives the myotomes the capacity to move the spine. The caudal, dense population give rise primarily to the neural arch and related parts of each vertebra and also the intervertebral disc apart from its nucleus pulposus which is a remnant from the notochord. The original cranial, less dense population forms most of the body of the vertebra.
The number of somites is different in the various domestic species. In the dog, for instance, there are more than 40 somites. The first four are called occipital somites; they fuse with mesenchyme of the skull to form the occipital cartilages of the skull. The remaining somites take part in the formation of the vertebral column and the ribs. The development of sclerotomes proceeds in a craniocaudal sequence, as does chondrification of vertebrae. The pattern of ossification of vertebrae is less precise and thoracic vertebrae may begin to ossify before the cervical segments do. Vertebral ossification begins during the sixth week of gestation in the dog, and slightly later in the large domestic animals. Primary ossification centres are found in the middle of each vertebral body and laterally in the base of each neural arch. In altricial domestic animals, like the cat and dog, these ossification centres do not fuse dorsally before birth; secondary ossification centres appear during postnatal development in the periphery of the body to form epiphyses and distal parts of the transverse processes.
A typical vertebra consists of a vertebral body, a vertebral arch and foramen (through which the spinal cord passes), transverse processes and usually a spinous process, and it arises from the fusion of several cartilaginous primordia (Fig. 16-6). The body, which is derived from the ventromedial sclerotomal portions of the paired somites, surrounds the notochord and serves as a bony floor for the spinal cord. The neural arches, arising from lateral sclerotomal cells, fuse on both sides with the centrum, and along with other neural arches, they form a protective roof over the spinal cord. The costal process forms the true ribs at the level of the thoracic vertebrae. At other levels along the vertebral column the costal processes become incorporated into the vertebrae proper.
Development of ribs and sternum
The ribs arise from segmental sclerotome-derived condensation of mesenchymal cells lateral to the anlagen of the thoracic vertebrae, located between the developing myotomes (Figs 16-7, 16-8). The proximal part of a rib (head, neck, and tubercle) originates from the ventromedial sclerotome. Because of the resegmentation of the somites as they form the vertebrae, the distal part (shaft) of the rib is derived from the ventrolateral part of the adjacent cranial somite. By the time ossification begins in the vertebrae, the ribs separate from the vertebrae. Accessory ribs, especially in the upper lumbar and lower cervical levels, are common.
The distal ends of the first nine cartilaginous ribs grow towards the midline and make contact on each side with a longitudinal aggregation of somatic mesoderm called the sternal bar. The sternum arises from this pair of cartilaginous bands that converge at the ventral midline as the ventral body wall consolidates. The two bars fuse in the ventral midline and undergo a secondary subdivision into a series of sternebrae (Fig. 16-8). Usually a total of 8 sternebrae form, although it is not uncommon for the caudal sternebrae to remain paired. The sternebrae ultimately fuse as they ossify to form the common unpaired body of the sternum. Several common anomalies of the sternum, such as a split xiphoid process, are readily understood from its embryological development.
APPENDICULAR SKELETON
The appendicular skeleton consists of the bones of the limbs and limb girdles. There are fundamental differences in organization and developmental control between the axial and appendicular skeleton. The axial skeleton forms a protective casing around soft internal tissues like the brain, spinal cord, and pharynx, and it is these surrounded organs that induce the mesenchyme to form the bones. In contrast, the bones of the appendicular skeleton form a central supporting core of the limbs. Although interaction with an epithelium (the apical ectodermal ridge of the limb bud) is required for the formation of skeletal elements in the limb, morphogenetic control of the limb is inherent in the mesoderm, with the epithelium playing a stimulatory role. All components of the appendicular skeleton begin as cartilaginous models, which convert to true bone by endochondral ossification later during embryogenesis.
Development of the limbs
Forelimbs and hind limbs of the domestic mammals, as in all other terrestrial vertebrates, develop at defined positions in the cervicothoracic and lumbo-sacral regions of the body, respectively. Limb bud development begins towards the end of the third week of gestation in cats, sheep and pigs, and during the fourth week in dogs and cattle (Figs 16-9, 16-10). Development of the fore- and hind limbs is similar except that morphogenesis of the hind limbs is approximately 1 to 2 days behind that of the forelimbs.
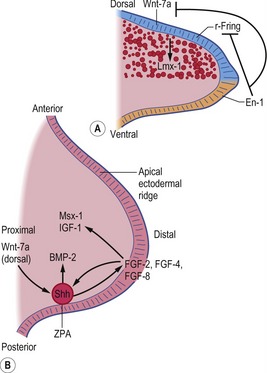
Fig. 16-9: Schematic diagram of molecular regulation of limb development (Carlson, 2004). A: Molecular control of the dorsoventral axis: En-1 inhibits both Wnt-7a and r-Fring. B: Molecular control along the anteroposterior and proximodistal axes. r-Fring: Radical fringe; ZPA: Zone of polarizing activity.
Limb bud
Each limb then develops from an encircled outgrowth of mesenchyme at the ventrolateral aspect of the embryo, which is covered with ectoderm. At the tip of the limb bud the ectoderm is thickened to the apical ectodermal ridge (AER), which plays a pivotal role in the organization of the limb during development (Fig. 16-9). In the earliest stages of limb development, the limb mesoderm is the prime mover. It secretes Fgf-10, which stimulates the overlying ectoderm to produce Fgf-8. An AER is found in all tetrapod vertebrates and its position corresponds exactly to the border between the dorsal ectoderm that expresses the signalling molecule radical fringe, and ventral ectoderm, which expresses the transcription factor Engrailed-1 (En-1). Experimental studies in mice have shown that removal of the AER results in arrest of limb development, leading to truncation of the limb.
Epithelio-mesenchymal interactions during limb morphogenesis
Extensive epithelio-mesenchymal interactions occur during limb development. The AER stimulates the outgrowth of the limb bud by promoting mitoses in the mesenchyme and by preventing differentiation. As the limb grows, the cells further away from the AER escape from its inductive influence, start to differentiate into cartilage and striated muscle, and limb development proceeds proximodistally. The existence of the AER is reciprocally controlled by signals from the mesenchyme of the limb bud.
Establishing of the limb axes
Limb buds display three obvious axes of asymmetry: proximodistal, craniocaudal, and dorsoventral (Figs 16-11, 16-12). At a molecular level, it is now possible to define the signals that control patterning of each of these axes, signals that must be integrated so that formation of the various limb elements, relative to the three axes, is coordinated.
Three key organizing centres produce primary signals to regulate patterning along the three axes of the embryonic limb. Proximodistal growth is regulated by the apical ectodermal ridge (AER), which produces proteins of the Fibroblast Growth Factor (FGF) family that act on the underlying limb mesenchyme. Craniocaudal patterning is controlled by a population of cells that secrete the Sonic hedgehog (Shh) protein in the posterior aspect of the limb mesenchyme (Fig. 16-9). Dorsoventral patterning requires localization of the WNT7a signalling protein to the dorsal limb ectoderm via repression by the Engrailed-1 (En1) transcription factor, localized in the ventral ectoderm.
Development of the basic limb structure
As the limb bud elongates, it becomes flattened in the dorsoventral plane of the embryo, and the distal part becomes paddle-shaped whereas the proximal part appears cylindrical (Fig. 16-13). As distal outgrowth continues, the limb bud bends ventrally and its originally ventral surface becomes the medial surface. Subsequently, the limbs rotate approximately 90° (clockwise in the left limb and counter clockwise in the right limb) along the proximodistal axis, which moves the cranial margins of the paddle-shaped distal parts of the limbs medially.
Hox genes regulate the type and shape of the bones of the limb. Hox gene expression is controlled by collective expression of Shh, Fgfs, and Wnt7a in phase and in places that correspond to the proximal, middle and distal parts of the limb. Genes of the Hoxa and Hoxd clusters are the primary determinants in the limb. Variations in their combined patterns of expression may cause some of the differences in forelimb and hind limb structures. As already described, other important factors in determining forelimb versus hind limb structure are the transcription factors TBX5 (forelimb) and TBX4 together with PITX1 (hind limbs).
Development of digits
The basic pattern of limb development is initially the same for all domestic mammals (Figs. 16-14, 16-15, 16-16) but becomes modified by species-specific changes during development. The standard pattern for the foot is the five-digit arrangement, characteristic for plantigrade animals.
< div class='tao-gold-member'>
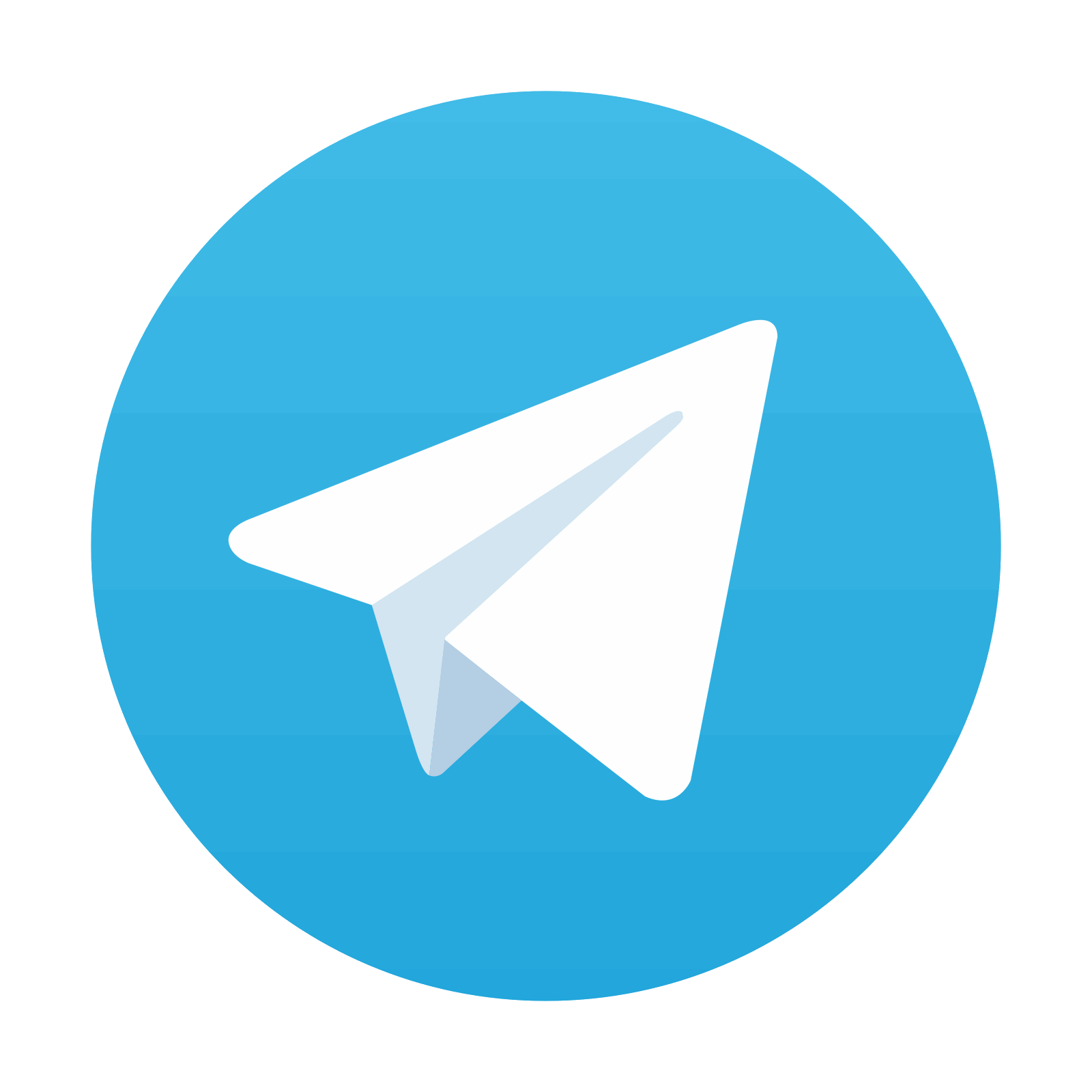
Stay updated, free articles. Join our Telegram channel

Full access? Get Clinical Tree
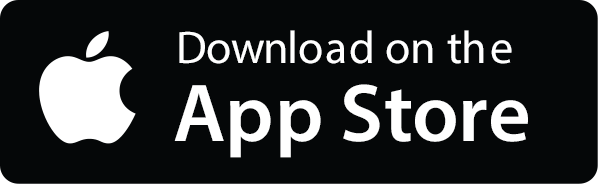
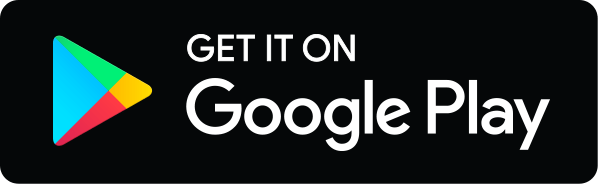