Chapter 1 Structure and Function of the Eye
Vision is a complex phenomenon in which light emanating from objects in the environment is captured by the eye and focused onto the retinal photoreceptors (Figures 1-1 and 1-2). Electrical signals originating from these cells pass through a number of cell types in the retina and throughout the central nervous system (CNS) before arriving at the visual cortex, where the sensation of vision occurs. Numerous species variations exist on this basic theme, each allowing the animal to exploit a particular ecologic niche. The basic similarities among all vertebrate eyes and how they respond to insult allow the comparative ophthalmologist to confidently treat a wide range of ocular conditions in a diverse array of species.
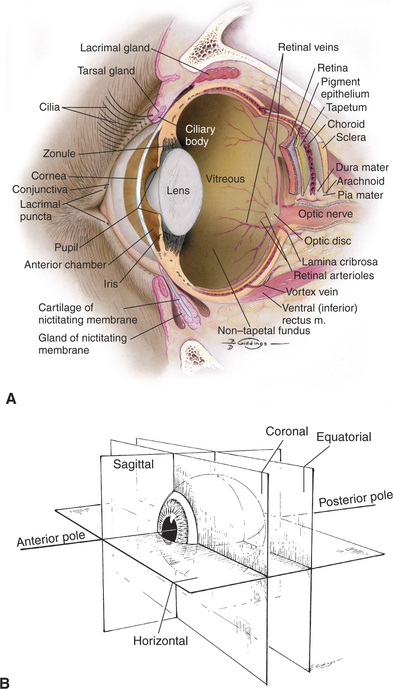
Figure 1-2 Internal structures of the canine eye (A). Also shown are the standard reference planes (B).
FUNDAMENTALS OF VISION
Sensitivity to Light
The visual system of most domestic mammals has evolved to improve performance under a wide range of lighting conditions so that they may exploit specific ecologic niches. Of domestic mammals, cats are probably the most efficiently adapted for nocturnal vision, with a minimum light detection threshold up to seven times lower than that in humans. Other adaptations that permit cats to function well in nocturnal conditions are a tapetum lucidum, which reflects 130 times more light than the human fundus; a vertical slit pupil, which produces a smaller aperture in bright light than what is possible with a circular pupil but also allows the pupil to dilate 6mm more than the human pupil; a large cornea, which permits more light to enter the eye; a relatively posteriorly located lens, which produces a smaller but brighter image on the fundus; and a retina rich in light-sensitive rod photoreceptors (Figure 1-3). Many of the other domestic mammals have similar but fewer extreme adaptations for vision in dim light, allowing them to exploit a photic environment that is not strictly diurnal or nocturnal.
The tapetum is cellular in dogs and cats and collagenous in horses and ruminants, suggesting that the visual advantages this structure offers are of sufficient magnitude that it has evolved separately at least twice in mammals (Figure 1-4). In both cases, the variety of tapetal colors seen during ophthalmoscopy results from the differential interaction of light with the tapetum’s physical structure rather than from the inherent spectral composition, or color, of its pigments. The dorsal location of the tapetum may enhance the view of the usually darker ground, and the ventrally located, usually darkly pigmented nontapetal region may reduce light scattering originating from the brighter sky. In cats, the tapetum may also absorb light in the shorter wavelengths and, via fluorescence, shift it to a longer wavelength that more closely approximates the maximal sensitivity of the photopigment, rhodopsin. This shift may brighten the appearance of a blue-black evening or night sky and enhance the contrast between other objects in the environment and the background sky.
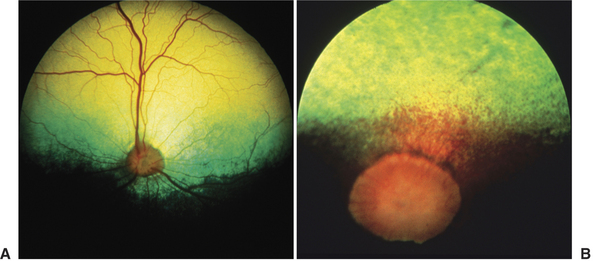
Figure 1-4 Cellular tapetum of a dog (A) and fibrous tapetum of a horse (B).
(B from Gilger B [2005]: Equine Ophthalmology. Saunders, St. Louis. A and B courtesy Dr. Christopher J. Murphy.)
The rhodopsin photopigment of dogs and cats is tuned to a slightly different wavelength of light from that in humans and, as is typical of species adapted to function well in dim light, takes longer to completely regenerate after extensive exposure to bright light. The ranges of wavelengths to which rhodopsin in dogs, cats, and humans is sensitive are similar, however, indicating that vision in dim light is not enhanced by expanding the range of detectable wavelengths. The slight wavelength shifts in the maximal sensitivity of rhodopsin across species suggests that domestic mammals and humans do not perceive the world in exactly the same way.
Sensitivity to Flickering Lights
Although not related to motion detection, the point at which rapidly flickering light fuses into a constantly illuminated light (flicker fusion) provides insight into the functional characteristics of rod and cone photoreceptors. The flicker frequency at which fusion occurs varies with the intensity and wavelength of the stimulating light. Because dogs can detect flicker at 70 to more than 80Hz, a television program in which the screen is updated 60 times/sec and appears to people as a fluidly moving story line may appear to dogs as rapidly flickering.
Visual Field of View
The extent of the visual field (i.e., the area that can be seen by an eye when it is fixed on one point) and the height of the eyes above the ground may vary greatly among breeds and species and has a major impact on the perception an animal has of its environment (Figure 1-5). For example, when the visual fields of its two eyes are combined, the horse has a total horizontal visual field of up to 350 degrees, with 55 to 65 degrees of binocular overlap and a virtually complete sphere of vision around its body (Figure 1-6). The length of the horse’s nose interferes with binocular vision, and so a horse views an object binocularly until it is about 1m away, at which point the horse must turn its head and observe with only one eye. In comparison, humans have a visual field of approximately 180 degrees (140 degrees of overlap), cats have a 200-degree field of view (140-degree overlap), and depending on breed, dogs have 250 degrees (30 to 60 degrees of binocular overlap) (Figure 1-7). The horse has only a few minor “blind spots,” which are located superior and perpendicular to the forehead, directly below the nose, in a small oval region in the superior visual field where light strikes the optic nerve itself, and the width of the head directly behind. Clearly, this extensive visual field makes it very difficult for a person or potential predator to “sneak up” on a horse.
Depth Perception
Depth perception is enhanced in those regions in which the visual fields of the two eyes overlap. Merely viewing an object with both eyes simultaneously does not guarantee improved perception of depth. Stereopsis (binocular depth perception) results when the two eyes view the object from slightly different vantage points and the resulting image is blended or fused into a single image. If the two images are not fused, double vision may result. (Such an alteration in vision may occur in animals with orbital diseases.) Although binocular depth perception is superior if the images can be blended into one, monocular depth perception is also possible. Horses make distance judgments on the basis of static monocular clues; these clues include relative brightness, contour, areas of light and shadows, object overlay, linear and aerial perspective, and density of optical texture. In addition, movement of the head results in an apparent change in the relative positions of the objects viewed (a phenomenon known as parallax) and produces the sensation that objects are moving at different speeds, allowing depth to be estimated (Figure 1-8).
Visual Acuity
Optical Factors in Visual Acuity
The optical media of the eye, namely the cornea, aqueous humor, lens, and vitreous humor, are responsible for creating a properly focused image on the retina. The cornea and, to a lesser extent, the lens are the principal refracting surfaces of the eye, and their ability to bend (refract) light is determined by their radii of curvature and the differences between their refractive index and that of the adjacent air or fluid. If the focal length of the focusing structures of the eye does not equal the length of the eye, a refractive error is present. In a normally focused (emmetropic) eye, parallel rays of light (effectively anything 20 feet or more away from the eye) are accurately focused on the retina. If parallel rays of light are focused in front the retina, myopia (nearsightedness) results. If they are focused behind the retina, hyperopia (farsightedness) results (Figure 1-9). Such errors in refraction are usually expressed in units of optical power called diopters (D). The extent of the error can be expressed by the formula D = 1/f, where f equals the focal length (in meters) of either the lens or the optical system as a whole. Therefore if an eye is 2D myopic at rest, it is focused at a plane located 0.5m in front of the eye. Similarly, an eye that is emmetropic at rest but can accommodate (change focus) 3D is capable of clearly imaging objects on the retina that range from as far away as the visual horizon (infinity) to as near as 0.33m in front of the eye.
In addition to myopia and hyperopia, other optical aberrations (e.g., astigmatism) may result from imperfections in the refractive media such as the cornea or lens and lead to degradation of the image formed on the retina. Astigmatism occurs when different regions of the optical system (especially cornea or lens) do not focus light in a uniform fashion, resulting in warping of the image, an extreme example of which can be found in the irregular mirrors found at carnivals. Spontaneous astigmatism is generally uncommon in dogs but has been observed in a variety of breeds. Astigmatism commonly accompanies corneal diseases that result in scarring and distortion of the corneal curvature (Figure 1-10).
Although visual acuity requires that optical portions of the eye be transparent and that optical blur from refractive errors or astigmatism be limited, an adjustable focusing (accommodative) mechanism is needed if objects at different distances are to be seen with equal clarity. Accommodation in dogs and cats may be brought about by altering the curvature of the lens surface or, more likely, by moving the lens anteriorly (Figure 1-11). The accommodative range for most domestic animals is quite limited and does not generally exceed 2 to 3 D for dogs, 4 D for cats, and less than 2 D for horses. This finding suggests that dogs are capable of accurately imaging objects on the retina that are within 50 to 33cm of their eyes but that objects nearer than this will be blurred. Hence, dogs use other senses, such as smell or taste, to augment vision in the investigation of very near objects. For comparison, young children are capable of accommodating approximately 14 D, or to about 7 cm.
Loss of the lens, as occurs after cataract surgery, results in severe hyperopia (farsightedness), with objects being focused approximately 14 D behind infinity, and a reduction in visual acuity to 20/800 or worse. This means that aphakic eyes are unable to image any object clearly, whether near or far away, and are unable to accommodate. Although the aphakic dog is extremely “farsighted,” it must be kept in mind that, for objects of similar size, objects that are closer to the dog will create a much larger image on the retina than objects that are located far away. Therefore the aphakic dog may be able to better visually orient to near objects despite being “farsighted.” Surprisingly, although this degree of hyperopia is markedly debilitating to some dogs, most dogs are still able to visually orient adequately in their environment without correction.
Retinal Factors in Visual Acuity
Domestic mammals lack the highly developed primate fovea but, instead, have a generally oval visual streak that contains the greatest density of photoreceptors, ganglion cells, and rhodopsin and thereby affords the greatest visual acuity. The visual streak, located in the tapetal region slightly superior and temporal to the optic nerve, has approximately linear, short temporal and longer nasal extensions (Figure 1-12). The oval temporal part of the visual streak is relatively free of blood vessels larger than capillaries, and nerve fibers take a curved course to the optic disc dorsal and ventral to the visual streak, presumably to avoid interfering with light reaching the photoreceptors. The temporal, oval portion of the streak may facilitate binocular vision, whereas the nasal, linear portion may be used to scan the horizon and better use the wider field of view available to the domestic mammals.
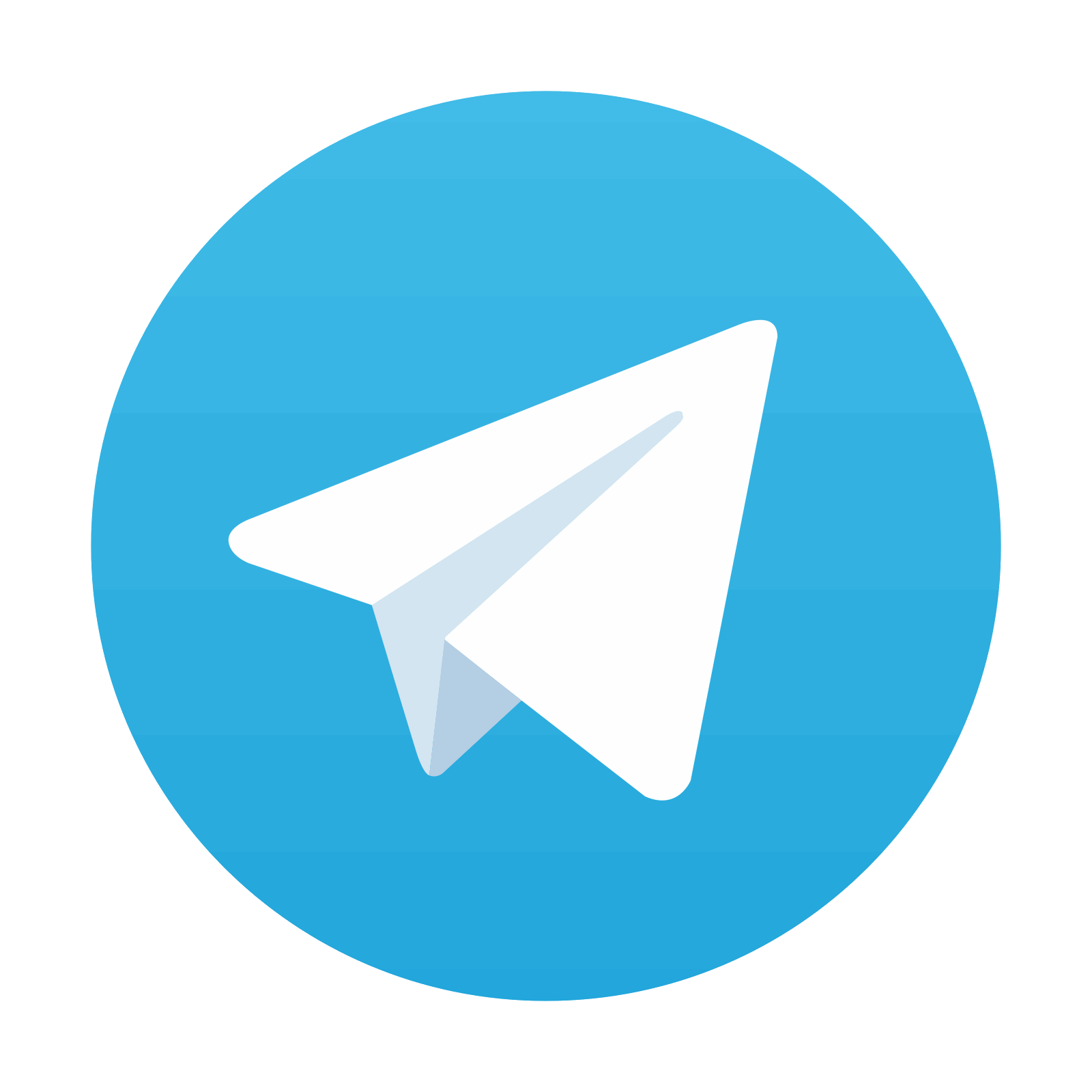
Stay updated, free articles. Join our Telegram channel

Full access? Get Clinical Tree
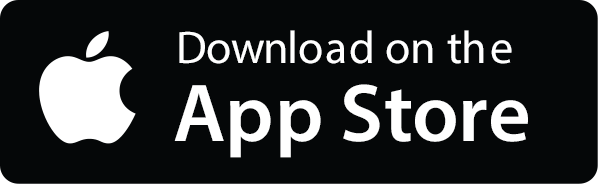
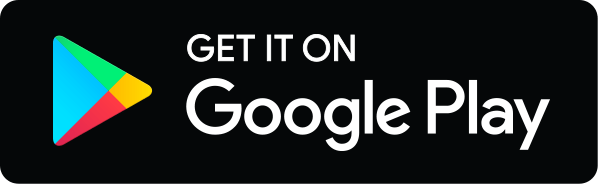