© Springer Science+Business Media New York 2015
Mónica V. Cunha and João Inácio (eds.)Veterinary Infection Biology: Molecular Diagnostics and High-Throughput StrategiesMethods in Molecular BiologyMethods and Protocols124710.1007/978-1-4939-2004-4_22. Significance and Integration of Molecular Diagnostics in the Framework of Veterinary Practice
(1)
Departamento de Sanidad Animal, Facultad de Veterinaria, Universidad Complutense de Madrid, 28040 Madrid, Spain
Abstract
The field of molecular diagnostics in veterinary practice is rapidly evolving. An array of molecular techniques of different complexity is available to facilitate the fast and specific diagnosis of animal diseases. The choice for the adequate technique is dependent on the mission and attributions of the laboratory and requires both a knowledge of the molecular biology basis and of its limitations.
The ability to quickly detect pathogens and their characteristics would allow for precise decision-making and target measures such as prophylaxis, appropriate therapy, and biosafety plans to control disease outbreaks. In practice, taking benefit of the huge amount of data that can be obtained using molecular techniques highlights the need of collaboration between veterinarians in the laboratory and practitioners.
Key words
Molecular diagnosticsPCRRT-PCRVeterinary clinical microbiologyPathogens1 Introduction
Molecular techniques, fueled by polymerase chain reaction (PCR), have revolutionized the diagnostics in veterinary practice. Molecular approaches are now widely accepted for identification of etiological agents and in many cases have replaced traditional systems based on the more cumbersome phenotypic identification. Several assays are currently described for the detection and identification of many pathogens of the OIE–listed diseases and other diseases of importance to international trade. However, compared to serological techniques, molecular assays are still officially recommended as prescribed tests only for a limited number of diseases, when concerning the international trade and movement of animals and animal products (http://www.oie.int/en/international-standard-setting/terrestrial-manual).
The development of molecular methods has provided new tools for enhanced surveillance and outbreak detection, and this may result in better implementation of infection control programs. Benefits of using molecular diagnostics are clear in terms of time to result and accuracy; however, laboratories also face several challenges when implementing molecular diagnostic assays, related with throughput, quality control, direct use on clinical samples, or difficulties for the definition of gold standards to assess the techniques. The aim of this chapter is to give an overview of the general uses of molecular techniques in routine work of veterinary diagnostics and to discuss some of their limitations.
2 Basic Approaches in the Laboratory
In the past 20 years, international research efforts have provided an exponential knowledge on pathogen genomic sequences and the availability of molecular technologies of different complexity. This knowledge has resulted in powerful tools that enable the rapid and specific diagnosis of animal diseases [1, 2]. The practical relevance of these techniques is directly related to the problems that the pathogens pose in the laboratory; the more fastidious the pathogen, or noncultivable, the more useful will be the molecular technique. However, for some fast-growing pathogens, biochemical characterization using commercial automated systems is still a valuable tool. Accordingly, molecular techniques are of paramount importance for diagnosis of viral diseases and for some serious bacterial pathogens, such as brucellae, mycobacteria, and rickettsiae, which take considerable time to grow in vitro and/or require specific culture media or cell culture systems, and are not adequate for wide-scale culture-based diagnosis. Moreover, additional time is required for the biochemical or serological identification of the isolates. It is also known that false-negative culture results may occur. To overcome these limitations, efforts have focus on the PCR amplification of nucleic acids directly extracted from clinical specimens or environmental samples. Extensive research and use of these techniques have increased our knowledge of pathogens and epidemiology of the diseases.
Molecular diagnosis of diseases caused by main pathogens of veterinary interest can be applied following two main approaches:
1.
Coupled to conventional microbiological culture to identify bacterial or fungal isolates, or cell culture in the case of viruses, replacing the more cumbersome biochemical or serological identification. This requires only a step to inactivate the pathogen and to prepare the nucleic acids that will work as template for the amplification reactions. In many instances, the suspension of the colonies in distilled sterile water and simple boiling is enough for DNA extraction, being suited for laboratories even with minimum resources.
2.
Direct detection of the pathogen’s nucleic acids in clinical specimens (e.g., tissues, feces, body fluids, and swabs). In this case, the direct detection presents the advantage of rapidity (which can mean saving up to several months) and the possibility to work with inactivated material, so reducing the risk of laboratory hazards (important when pathogens are also zoonotic). However, the direct detection of pathogen’s nucleic acids in clinical samples requires the use of improved DNA/RNA extraction methods. Although amplification-based assays are extremely sensitive when used on microbial cultures, its sensitivity may drop when directly applied to samples eventually containing inhibitors. Protocols requiring many steps involving mechanical, chemical, and enzymatic disruption of microbial cells, or involving commercially available DNA/RNA extraction and purification kits, may be useful and efficient for research activities but may not be adequate or be cost-prohibitive when handling a large number of samples with routine diagnostic purposes, as during disease outbreaks (high pressure to analyze many samples in the minimum period of time) or surveillance (high number of samples during an extended period of time). Furthermore, the use of automated work stations that handle several samples at a time may not be available in resource-limited laboratories. The effectiveness of every nucleic acid extraction protocol depends on the matrix (i.e., type of clinical sample) and the pathogen and needs to be adjusted for each specific combination.
3 Level of Information
Most molecular diagnosis approaches currently used in the routine veterinary laboratory rely in the PCR and its variants, such as nested-PCR and real-time PCR. The performance of these assays mainly depends upon the primers used, which are usually a pair of short oligonucleotide fragments complementary to flanking regions of the target sequence and that will specifically anneal to the microbial genetic material present in the sample. The laboratory can design in–house PCRs based on previously well-characterized and validated genomic targets and primers sequences available in scientific publications and online databases. Otherwise, the laboratory staff would need to select novel targets and to design appropriate primers (free software can be downloaded and used for this purpose). Ideally, the primers should be 100 % specific and, at the same time, should detect all variants that are being “hunted”; this may require a balance between the use of conserved and of highly variable genetic regions as complementary targets for those primers. Depending on the primer design strategy, molecular tests can allow the identification of the etiological agents further than the species level, such as distinct groups (lineages) with a characteristic geographic spread, for instance, the Mycobacterium bovis clonal complexes [3] or the Brucella melitensis groups [4]. The possibility of designing own primers allows to focus diagnosis on local needs and priorities but will require further experimental optimization of the PCR-based reactions in every laboratory. Nevertheless, an increasing number of commercial kits to detect pathogens have become available; some diagnostic kits are certified by the OIE as fit for purpose.
Regarding the number of distinct sequence targets that are detected per amplification reaction, there are two strategies: the use of a single target (singleplex) or of several targets (multiplex). The latter strategy involves the use of multiple different pairs of primers in the same PCR, usually yielding amplification products with distinct lengths. Multiplexing allows for an increased workflow and reduced cost and has been used mainly with the two following objectives:
1.
To identify pathogens to the species, or even to the strain level using, e.g., specific genes, genetic regions, or insertion sequences, or specific nucleotide polymorphisms, as complementary targets. Examples are the tests to distinguish the classical Brucella species and vaccine strains [5] and to identify the biovars of B. suis [6], using standard gel electrophoresis to assess the amplification results, or the test for ruminant Mycoplasma spp. using a bead-based liquid suspension array [7].
2.
To allow the simultaneous detection of multiple pathogens in a same specimen. This option may be used in a syndromic approach, i.e., to identify the etiology of diseases caused by different pathogens but with similar clinical signs or pathological findings, such as the simultaneous detection of Chlamydia spp., Coxiella burnetii, and Neospora caninum in abortion material from ruminants [8]; the detection of C. burnetii and N. caninum in equine-aborted fetuses and neonates [9]; and the diagnosis of Brucella ovis, Actinobacillus seminis, and Histophilus somni infection in ram semen [10, 11], among many other examples. In combination with reverse transcription PCR (RT-PCR), this multiplex strategy is being used to detect endemic and different transboundary porcine viruses [12–14]. There is a trend to multiplexing, aiming to develop syndrome-specific assays targeting multiple candidate agents, but the design of the assays needs to be evaluated experimentally in order to check that test sensitivities do not significantly decrease when compared to singleplex testing.
Molecular techniques can be also applied to identify “clones.” PCR-based techniques such as multilocus variable-number tandem repeat analysis (MLVA) are less demanding and less time-consuming than other previously used methods, such as the analysis of restriction fragment length polymorphisms (RFLP) or pulse-field gel electrophoresis (PFGE), and provide results that can easily be compared between laboratories. Results obtained with the implementation of these techniques (called molecular epidemiology) may be combined with classical epidemiology to understand the dynamics of an infection and to design control measures.
These results can be worked out with phylogenetic purposes as well. A variety of PCR-based methods have been used, involving, e.g., the study of the presence or absence of large pieces of DNA, called regions of difference (RD) or large sequence polymorphisms (LSPs), which are expected to represent unidirectional evolution events, and of single nucleotide polymorphisms (SNPs), which are fast to test and less prone to homoplasy. As an example, these genomic targets have been used to delineate the phylogeny of the members of the Mycobacterium tuberculosis complex [15, 16].
Whole-genome sequencing is becoming affordable and applied to accumulate information on the biology of known pathogens or to disclose novel emerging pathogens. Analysis of complete genomes, or representative parts of the genome, can reveal the probable chain of transmission events and demonstrate the value of such techniques in providing information useful to control programs, as it has been done with the genetic analysis of the complete genome of the foot-and-mouth virus in the last outbreak in the UK [17]. In a near future, application of whole-genome sequencing analysis will offer definitive information about each pathogen.
The detection of unknown pathogens has been possible using broad-based primers with degenerate sequences. A most recent approach consists of a combination of nonspecific amplification and extensive sequencing. This approach requires a sound knowledge of taxonomy and genomics [18]. Furthermore, these new technological trends provide with an enormous amount of data that must be mined by bioinformatics. As an example, the use of a metagenomics approach allowed to identify the Schmallenberg virus, a novel orthobunyavirus that affected cattle in Europe in 2011 [19].
4 The Technique That Is Best Suited for the Laboratory
The choices for the most adequate molecular techniques and equipments are dependent on the mission and attributions of the laboratory. Several methods of nucleic acid amplification have been developed, and some of them are now common in veterinary diagnostics. Thermal cyclers to perform basic PCRs have become compact, easy to use, and (relatively) affordable equipments present in almost every diagnostic infrastructure. PCRs are frequently used in combination with an electrophoretic transfer cell to analyze the molecular weight of the amplified products. Additionally, laboratories may have an imaging system and software for gel documentation.
The success of PCR-based assays relies on its performance and versatility but also in the ease of use and affordability. Standard PCR requires the use of crucial reagents including dNTPs, a thermostable DNA polymerase and the respective buffer, and a pair of well-designed primers to make millions of copies of the deoxyribonucleic acid target in a couple of hours. In theory, the number of copies could reach 2 n , where n is the number of rounds of denaturing-annealing-extension steps. The amplified product (amplicon) can be detected by simple electrophoresis on an agarose gel and staining with a fluorescent dye (e.g., SYBR® Green) that preferentially binds to double-stranded DNA. An internal molecular weight ladder is run at the same time in the gel to estimate the size of the amplicons. The presence of the amplicon of the defined size (in base pairs) indicates the presence of the target DNA and, therefore, of the pathogen. An alternative system of detection consists on reverse hybridization where amplicons are denatured and allowed to hybridize with specific probes (single-stranded DNA) that are immobilized on a membrane strip. Subsequently, bound products may be detected, e.g., using an alkaline phosphatase reaction and a colorimetric detection assay. This system can detect sequences and specific point mutations in a gene and is commonly used to detect genotypes or pathogens (species identification, presence of toxin genes and of other virulence factors), as well as resistances to drugs.
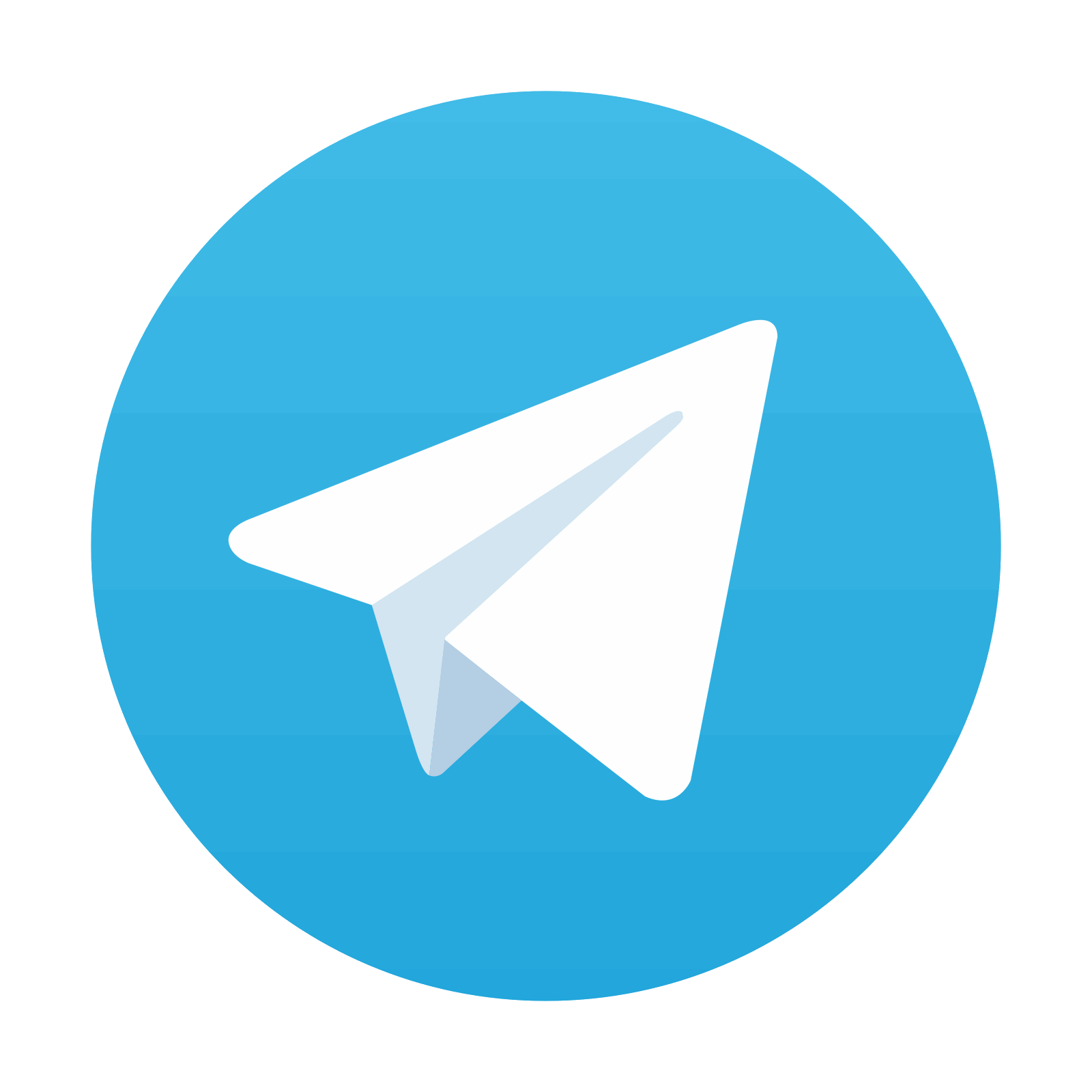
Stay updated, free articles. Join our Telegram channel

Full access? Get Clinical Tree
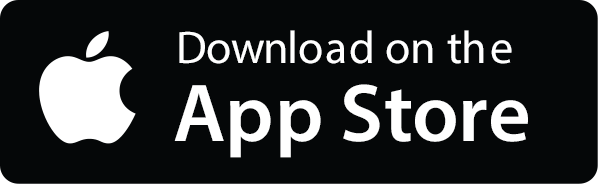
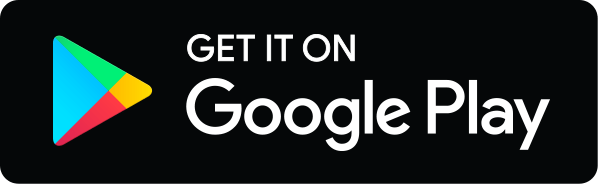