Erin L. Westman Véronique L. Taylor and Joseph S. Lam Pseudomonas is a diverse genus of rod‐shaped bacteria with broad metabolic diversity that is found ubiquitously in the environment. Many pseudomonads are non‐pathogenic, but P. aeruginosa is an important opportunistic pathogen. As an opportunist, it generally requires a defect or alteration in the normal host defenses to establish an infection. Injury, trauma, surgery, burns, or indwelling devices such as intravenous lines or urinary catheters make the host susceptible to P. aeruginosa colonization. One example of how the physiological versatility of P. aeruginosa contributes to this pathogen being an “opportunist” is clearly demonstrated in a study by Daly et al. (1999), who identified P. aeruginosa to be the causative agent of a severe outbreak of mastitis among 11 Irish dairy herds (Daly et al. 1999). It was a remarkable revelation that the teat wipes used on these dairy herds were contaminated with P. aeruginosa, even though the wipes were stored in 70% alcohol. These wipes were meant to sterilize the teats before injecting antibiotics into the mammary glands, but instead, this provided a direct inoculation of P. aeruginosa onto the tissues. P. aeruginosa pathogenesis has been best studied in human forms of the disease, but the three distinct stages of infection also hold true in animals. These stages are adherence and colonization, which is generally modulated by cell‐surface components of the bacterium including flagella, pili, and lipopolysaccharide (LPS). Next, growth and multiplication of Pseudomonas involves genetic regulatory systems upregulating the expression of virulence factors including extracellular (secreted) products, type‐III secretion system (T3SS), change to the biofilm mode of growth, all in the presence of intrinsic multidrug resistance conferred by low outer‐membrane permeability and numerous efflux pumps. In fact, P. aeruginosa exhibits broad antibiotic resistance, including to commonly used biocides like triclosan (commonly found in antibacterial hand soap). This multifactorial resistance frequently makes infections difficult to manage, and careful selection of therapeutic agent is necessary. Resistance may also emerge very quickly. Careful surveillance of treatment outcomes is wise, with repeated culture susceptibility testing if the infection is not resolved. Pseudomonas are Gram‐negative straight or slightly curved rod‐shaped eubacteria. The cells are around 0.5–1.0 × 1.5–5.0 μm in size and are motile by a single flagellum or multiple polar flagella. Part of the γ‐proteobacteria, Pseudomonas are aerobic chemoheterotrophs, and have remarkable nutritional diversity, using a wide variety of organic compounds as carbon sources and electron donors for energy production. As a result of this robust metabolism, Pseudomonas are important to the breakdown of organic materials to inorganic substances (mineralization) in nature. In addition, pseudomonads such as P. fluorescens grow at 4 °C and are important in the spoilage of refrigerated milk, meat, eggs, seafood, and even blood products. Some pseudomonads including P. aeruginosa, P. fluorescens, P. putida, and P. syringae produce a diffusible, water‐soluble, yellow‐green pigment called pyoverdine that fluoresces under ultraviolet light. Pyoverdine is produced under conditions of iron limitation and can be useful diagnostically in identifying these pseudomonads. When iron is limited, Pseudomonas aeruginosa also produces a blue‐green phenazine pigment called pyocyanin. Infections caused by P. aeruginosa are suppurative with a characteristic blue‐green color caused by the secretion of this pigment. Both molecules are siderophores, which are iron (III) chelators that are secreted to the environment to scavenge iron. High‐affinity receptors on the bacterial cell‐surface uptake the siderophore, thus improving the growth of Pseudomonas under iron‐limiting conditions like those encountered during infection of animals. The most significant Pseudomonas species include P. aeruginosa, P. fluorescens, P. putida, and P. syringae. Among these, P. putida and P. syringae are associated with infections of plants. P. fluorescens is non‐pathogenic, since they grow best at cooler temperatures (around 4°C). P. aeruginosa is the predominant species associated with disease in both humans and animals, its role as an opportunistic pathogen is the focus of this chapter. P. aeruginosa cells are around 0.3–0.5 μm × 1–2 μm in size and secrete the iron‐sequestering siderophores pyoverdine and pyocyanin when under low‐iron stress; these give mature cultures a characteristic fluorescent‐green or blue‐green color, respectively (Figure 14.1). The optimal growth temperature is 37°C, but these bacteria can thrive at temperatures between 10°C and 40°C. In humans, P. aeruginosa causes infections mainly of the blood, lungs, or other parts of the body after surgery. The Centers for Disease Control and Prevention (2019) report on antibiotic resistance threats in the United States lists multidrug‐resistant P. aeruginosa as a serious threat, the second most significant tier. Each year, multidrug‐resistant P. aeruginosa is estimated to cause 32 600 hospitalized cases and 2700 human deaths (Centers for Disease Control and Prevention 2019). Similarly, P. aeruginosa is reported to be an emerging nosocomial infection of veterinary significance (Bernal‐Rosas et al. 2015). Figure 14.1 P. aeruginosa. (Left) Transmission electron micrograph of negatively stained P. aeruginosa cells. Black arrow points to the single polar flagellum, and the dashed arrow indicates pili. Bar: 1 μm. (Right) Stationary‐phase culture normally exhibits the characteristic blue‐green color due to siderophore secretion. The white arrow points to the pellicle, a biofilm at the liquid/air interface. P. aeruginosa is ubiquitous and can be isolated from most natural habitats including water, soil, plants, and sewage. An opportunistic pathogen, it is often found in the hospital setting, including respiratory equipment, cleaning solutions, medicines, disinfectants, sinks, and floors, and accounts for about 10% of human nosocomial infections and between 0.8% and 18% of veterinary nosocomial infections (Nelson 2011). Transmission of P. aeruginosa is usually through indirect contact via contaminated tools such as thermometers, drinking bowls, bedding, or surgical implements. Owing to its ubiquity in the environment, it is readily reintroduced into the clinic from human and animal sources. Differentiation of clinical isolates is important for epidemiological studies and is facilitated by numerous typing schemes. Although no system is universally employed, serotyping is a commonly used serological method in clinical settings to classify P. aeruginosa based on differences in the O antigen of the LPS present on the cell surface of these bacteria. Two forms of O‐antigen polysaccharides are produced by P. aeruginosa: the homopolymeric common polysaccharide antigen, which is common to most strains, and the heteropolymeric O‐specific antigen (OSA). Based on the saccharide composition and linkages of the OSA O antigen, the International Antigenic Typing Scheme (IATS) recognizes 20 serotypes of P. aeruginosa. Although the IATS is the most commonly used, other typing schemes have been employed in the past and include the Fisher immunotypes, and the Lanyi and Bergan O serogroups. Other typing schemes for the classification of P. aeruginosa include biotyping, antibiogram analysis, pyocin typing, and bacteriophage typing, as well as more accurate and sensitive genotyping/DNA fingerprinting methods including pulsed‐field gel electrophoresis, ribotyping, and polymerase chain reaction (PCR)‐based techniques, such as amplified fragment length polymorphism analysis and random amplified polymorphic DNA analysis. P. aeruginosa causes a variety of diseases in animals, including wound infections in all species, urinary tract infections and chronic purulent otitis externa in dogs, ulcerative keratitis in dogs and horses, and dermatitis (fleece rot) in sheep (Table 14.1). P. aeruginosa infections in which there is no obvious breach in host defenses include mastitis in cattle, genital tract infections in horses, and rhinitis or otitis in sheep. In one case, several ewes developed severe purulent rhinitis and otitis externa/media after being showered with a wash that had previously been used on another group of ewes with dermatitis (Watson et al. 2003). Sporadic outbreaks of septicemic disease occur in poultry, mink, and chinchillas. Mink in particular are highly susceptible to P. aeruginosa infection, as shown by an LD50 of 103–104 colony forming units observed in an intratracheal challenge, compared with 106–109 in other species (Long et al. 1980). A vaccine has been developed to address severe hemorrhagic pneumonia in mink (Aoi et al. 1979). However, this vaccine has a limited protection in other animals and is not effective outside mink and chinchillas. Table 14.1 Diseases in animals caused by Pseudomonas aeruginosa. Source: Adapted from Gyles (1993). The most commonly encountered Pseudomonas infection in animals is otitis. In dogs, it is typically characterized by head shaking, ear scratching, purulent exudates, malodor, as well as swelling, inflammation, and pain. Whenever cytology laboratory tests reveal rod‐shaped bacteria, Pseudomonas should be suspected and treatment is warranted (Pye 2018). Otitis externa and otitis media are often diagnosed together, even if the tympanic membrane is intact, and different bacterial species and/or different trends in antibiotic susceptibility may be found in the external and middle ears (Cole et al. 1998). In some otitis cases, initial therapy may fail, or the otitis may reoccur rapidly following seemingly successful treatment. In these cases, culture and susceptibility testing are invaluable for selecting an appropriate antibiotic. P. aeruginosa may also cause urinary tract infections, and the high intrinsic antibiotic resistance of the organism makes it difficult to treat. Infections present with typical lower urinary tract signs such as voiding or obstructive symptoms, potentially including hesitancy, poor and/or intermittent stream, straining, dribbling, as well as irritative symptoms such as frequency and urge incontinence. Furthermore, evidence of bacterial cystitis including hematuria, pyuria, or cytologically evident bacteriuria are common. Aerobic bacterial urine culture is preferred to detect P. aeruginosa as the causative agent. For management of extended spectrum beta‐lactamase‐producing P. aeruginosa in urinary tract infections in dogs and cats, a carbapenem, especially imipenem administered with cilastatin (to slow the metabolism of imipenem) or a fluoroquinolone, such as marbofloxacin is suggested (Weese et al. 2019). Generally, swift and decisive therapy is required to resolve the infection without promoting resistance to the antibiotic agent. Any animal suffering from a wound or trauma is a potential candidate for infection by P. aeruginosa, and other predisposing factors include chronic debilitating illnesses, prolonged hospitalization, prior therapy with antimicrobial drugs, and impaired host immunity (cellular and/or humoral) owing to a primary disease or as a consequence of immunosuppressive or cytotoxic therapy. The success of P. aeruginosa as a pathogen is largely due to its ability to survive in a wide variety of environments and its arsenal of virulence factors (Table 14.2). P. aeruginosa virulence factors can be divided into four groups: cell‐surface components, extracellular (secreted) products, a, and genetic regulatory systems. Since this bacterial species is an important opportunistic pathogen of humans, our understanding of the pathogenic mechanisms contributed by virulence factors of P. aeruginosa is derived mostly from studies on animal models of human diseases. Table 14.2 Cell‐associated virulence factors produced by Pseudomonas aeruginosa. The four groups of virulence factors differ in the role played in the environmental life of the organism, the stage of pathogenesis influenced, and the target for manipulation by the factor. The cell‐associated factors are generally thought to be essential for the viability of the organism even outside the host, and include key structures in motility, adhesion, and the cell wall, as well as physiological states. The extracellular products are produced to gain nutrients or protect the cell from the immune response in the host, while the T3SS is a targeted mechanism for the delivery of several exotoxins directly into host cells. Finally, the regulatory systems enable P. aeruginosa to sense and react to environmental stimuli, such as the presence of other P. aeruginosa cells. In brief, the cell‐surface components of P. aeruginosa include flagella, pili, and LPS (Table 14.2). P. aeruginosa produces a single polar flagellum that is required for swimming motility and is important for initial colonization of damaged or altered epithelium. This organelle allows the bacterium to propel itself and also functions as an adhesin to anchor the cell to host epithelium. Using a neonatal mouse model of pneumonia, Feldman et al. (1998) demonstrated that fliC mutants of P. aeruginosa have decreased virulence. Histopathological examinations showed that the fliC mutant only caused focal inflammation, which is in contrast to the spread of inflammatory lesions throughout the lungs caused by the parent wildtype strain (Feldman et al. 1998). Flagella mutants were less invasive than the motile wild‐type strains in a burned‐mouse infection model and the addition of antiflagellum antibody had a significant protective effect (Montie et al. 1987). Pili are filamentous, polar cell‐surface structures of P. aeruginosa that are responsible for a form of flagella‐independent motility called twitching motility, which occurs over moist or semi‐solid surfaces. Pili are important for host colonization because they are thought to be the dominant adhesins of P. aeruginosa. The pili of P. aeruginosa are the prototypes of the Type 4 family of pili, also known as N‐methylphenylalanine pili. Cycles of extension, tethering, and retraction of the pili tow the bacterial cell forward, as if the cells were pulled along by a set of grappling hooks. Twitching motility is itself important for virulence. Strains that are piliated but not able to twitch (due to genetic deletions) are attenuated in virulence despite having the ability to associate with corneal epithelial cells as effectively as wild‐type bacteria (Zolfaghar et al. 2003). Pili also mediate adherence to DNA and abiotic surfaces such as stainless steel, polystyrene, and polyvinylchloride. Pilus‐deficient strains are significantly reduced in their ability to cause epithelial cell damage, to persist at the site of infection, and to disseminate throughout the host (Sato et al. 1988). LPS of P. aeruginosa consists of a hydrophobic lipid A component that anchors the LPS in the outer membrane, a core oligosaccharide that contains highly conserved sugar residues, and the long‐chain polysaccharide O antigen (also called O polysaccharide). LPS contributes significantly to virulence throughout the infection process, from initial attachment and colonization to avoidance of bacterial clearance during tissue invasion and dissemination in both acute and chronic infections. Lipid A is composed of a phosphorylated diglucosamine backbone substituted with fatty acids and is the endotoxic moiety that mediates inflammation and tissue damage associated with Gram‐negative septicemia. The core oligosaccharide of P. aeruginosa is produced in two forms: an “uncapped” core oligosaccharide (lacking attached O antigen) referred to as “rough LPS” and a “capped” form with attached O antigen that is referred to as “smooth” LPS (Sadovskaya et al. 1998, 2000). Bacteria possessing the rough form of LPS are particularly effective in adherence to, and invasion of, corneal epithelial cells in a cell culture assay. Similarly, rough LPS of P. aeruginosa is important in mouse infection models for the invasion of murine eyes (Zaidi et al. 1996) and airway respiratory epithelial cells (Pier et al. 1996). These data are consistent with the notion that P. aeruginosa LPS mediates internalization into epithelial cells. This is followed by replication so that a reservoir of intracellular bacteria is established and serves as a source to cause further spread of the infection. The O antigens that are attached to the capped form of core oligosaccharide are subdivided into two types: the common O polysaccharide, a repeating homopolymer composed of D‐rhamnose; and the heteropolymeric OSA, which is composed of di‐ to pentasaccharide‐repeating units of a variety of unusual sugars that define the serotype. The O polysaccharides are antiphagocytic and confer resistance to complement‐mediated killing. The O polysaccharide is required for virulence as shown in a burned‐mouse model study where the LD50 for the O‐antigen deficient mutant was at least 1000‐fold higher than that of the wild‐type strain (Cryz et al. 1984). In a separate study, Tang et al. (1995) used a neonatal mouse model and demonstrated that an LPS mutant was unable to initiate a respiratory tract infection whereas the wild‐type strain caused acute pneumonia, bacteremia and death (Tang et al. 1995). Extracellular products of P. aeruginosa also contribute to survival and resistance to antibiotic treatment (Table 14.2). In particular, P. aeruginosa is known for its biofilm mode of growth. Biofilms are communities of surface‐attached microorganisms enclosed in a matrix which can play a significant role in infectious disease (Costerton et al. 1999). Biofilms are very resistant to the killing effect of whole blood and serum and affect the pathogenesis of P. aeruginosa infections. The biofilm mode of growth also provides a two to three order of magnitude decrease in susceptibility to antimicrobial agents in comparison to planktonic (or free‐swimming) cells. Interestingly, bacterial cells within a biofilm show reduced metabolic activities, making them less susceptible particularly to those antibiotics that target primarily metabolically active cells. The matrix, or extracellular polymeric substance, that holds the biofilm together can contain protein, polysaccharide, and nucleic acid. The polysaccharide components of Pseudomonas biofilm extracellular polymeric substances (EPS) include alginate, Psl, and Pel (reviewed by Ryder et al. 2007). The T3SS and its secreted products are also key to the virulence of P. aeruginosa (Table 14.3). The T3SS is a specialized protein complex that acts as a “molecular syringe” to deliver cytotoxins directly from the bacterium into the cytoplasm of eukaryotic cells. Over 20 genes are required for secretion, translocation, and regulation of this system. The secretion of four cytotoxic proteins, ExoS, ExoT, ExoU, and ExoY, is induced by direct contact between the bacteria and the host cells. These proteins are postulated to promote epithelial cell injury, inhibit the phagocytic response to infection, and allow bacterial replication. The PcrV protein is part of a channel‐forming complex that is required for translocation of the effector proteins into the host cells. PcrV is required for virulence, lung injury and cytotoxicity in an acute lung infection model (Sawa et al. 1999). The last group of virulence factors are regulatory networks that coordinate these responses to the environment in which this opportunistic pathogen finds itself. Quorum sensing in bacteria is a cell‐to‐cell signaling system that microorganisms use to communicate with each other in a cell‐density dependent manner. It is used to modulate a variety of physiological processes including the production of many virulence factors (reviewed in Parsek and Greenberg 2000). P. aeruginosa has two quorum‐sensing systems, lasR‐lasI and rhlR‐rhlI (Figure 14.2). Each system is composed of two components: the transcriptional activator (LasR and RhlR, respectively) and an autoinducer synthase (LasI and RhlI, respectively). LasI and RhlI are involved in the synthesis of the cell‐to‐cell signaling autoinducer molecules 3‐oxo‐C12‐HSL (N‐[3‐oxododecanoyl]‐L‐homoserine lactone) and C4‐HSL (N‐butyrylhomoserine lactone), respectively (Parsek and Greenberg 2000). As a population of bacteria grows, the cell density increases and synthesis of the autoinducer molecules is upregulated. The autoinducers are released into the immediate area and move into surrounding bacterial cells. At a certain threshold concentration, the autoinducers bind to their respective transcriptional activator proteins, LasR and/or RhlR. The autoinducer/transcriptional activator complex binds to conserved DNA sequences upstream of target genes, thereby upregulating their expression. As an opportunistic pathogen, P. aeruginosa is adapted to survival both in the environment and in the host. The extensive cross‐talk and interconnections between production of seemingly disparate virulence factors probably reflects this diverse lifestyle. Some virulence factors are related through common biosynthesis steps, while others are coordinately or negatively regulated. At the top of the regulatory hierarchy are the global regulators, GacA and Vfr (Figure 14.2). GacA is a transcriptional activator and Vfr is a cyclic adenosine monophosphate receptor protein. Although the environmental stimuli that these global regulators respond to is not known, GacA and Vfr control quorum sensing at the level of transcription of the las and rhl systems. The las system is required for normal biofilm formation, and controls expression of the virulence factor genes responsible for the production of proteases (LasB and LasA elastase, and alkaline phosphatase) and exotoxin A, plus activates the expression of the rhl system. The rhl system regulates production of rhamnolipid and the stress response regulator RpoS, and is also required for optimal expression of LasB, LasA, hydrogen cyanide, alaline protease, and the siderophore pyocyanin. Table 14.3 The secreted arsenal of Pseudomonas aeruginosa. ExoA is secreted in response to iron starvation and is under the regulatory control of the lasR‐lasI quorum‐sensing system The clinical importance of P. aeruginosa requires rapid classification and identification of clones of concern. The typing of isolates has taken on many forms throughout the years; the earliest were enzyme‐linked immunosorbent assay and agglutination with antibodies specific to the OSA. These methods classify P. aeruginosa according to the IATS. This method allows for rapid classification of P. aeruginosa based on their OSA structures, which have been shown to be associated with specific ecological niches. Over time, the identification of specific resistance genes, or virulence factors has given rise to more focused PCR and restriction digestion‐based typing schemes such as pulse‐field gel electrophoresis (PFGE) and multilocus sequencing typing, in which a specific gene or region of the genome are amplified and sequenced. PFGE identifies patterns in restriction digested genomes of isolates and compare the “fingerprint” to establish relatedness, such as in an equine endometritis outbreak (Allen et al. 2011). With the advances of whole‐genome sequencing, typing can be done within a hospital and epidemiological studies of trends across the years can be done. By typing Pseudomonas in a holistic manner, overall Pseudomonas genome plasticity such as inversions and serotype‐switching, demonstrating the selective advantage of certain OSA structures and antibiotic resistance genes can be observed. An analysis of globally diverse P. aeruginosa strains revealed that around 88% of O12 serotype clones contained an island containing the biosynthesis cluster for the O12 serotype and a significant gyrase mutation attributed to ciprofloxacin resistance along with several efflux pumps. This “serotype‐switched” clone was identified from clinical specimens obtained throughout the globe (Thrane et al. 2015). Taking serotyping to the modern era, whole‐genome sequencing data can be mined to determine serotypes of any particular clinical isolate through in silico (online‐based) analysis (Thrane et al. 2016). Whole‐genome sequencing also helps to identify lineages of problematic clones and has observed certain clones have the possibility of zoonotic transmission. Nowadays, we have reached an era in which predictive computer algorithms in combination with whole‐genome sequencing can be used to track down the source of outbreaks (Sundermann et al. 2020). Figure 14.2 Quorum‐sensing systems of P. aeruginosa. The cascade of events begins with the transcription of lasR, which regulates PQS production and the Rhl system and ends with the activation of RhlR/C4‐HSL controlled genes. Arrowheads indicate positive regulation whereas a short parallel line indicates negative regulation. PQS 2‐heptyl‐3‐hydroxy‐4‐quinolone; 3‐oxo‐C12‐HSL N‐[3‐oxododecanoyyl]‐L‐homoserine lactone; C4‐HSL N‐butyrylhomoserine lactone. Source: Adapted from Venturi (2006) and Van Delden and Iglewski (1998). The pathogenesis of P. aeruginosa infections can be divided into three distinct stages: bacterial colonization, multiplication, and systemic disease (Figure 14.3). The first step in establishing an infection is colonization of host tissues. For an animal host, the first line of defense against bacterial invasion is the skin and the mucous membranes. However, if these tissues are damaged by injury or compromised by the insertion of a urinary catheter or intravenous line, bacteria will be able to attach to the epithelial cells, predominantly by the cell‐associated structures such as pili, flagella, and LPS. Interestingly, damaged and regenerating respiratory epithelium express greater amounts of receptors on their cell surface for P. aeruginosa
14
Pseudomonas
Introduction
Characteristics of the Organism
Pathogenic Species
Sources of Infection: Ecology, Evolution, and Epidemiology
Types of Disease and Pathologic Changes
Animal species
Diseases
All species
Wound infection
Dogs, cats
Otitis externa, urinary tract infection, ulcerative keratitis, pneumonia
Birds
Respiratory infections
Cattle
Respiratory infections, mastitis, enteritis
Horses
Genital tract infection, abortion, ulcerative keratitis
Swine
Respiratory infections
Poultry
Septicemia, keratitis
Sheep
Pneumonia, mastitis, fleece rot, rhinitis, otitis
Mink
Hemorrhagic pneumonia, septicemia
Chinchillas
Pneumonia, septicemia
Laboratory rodents
Septicemia, enteritis
Virulence Factors and Pathogenomics
Virulence factor
Proposed biologic effects
Notes
Alginate/biofilm
Antiphagocytic, adherence to epithelium, antibiotic resistance
Alginate important but not essential for biofilm formation (Wozniak et al. 2003)
Flagellum
Chemotaxis, mediates motility for invasion, adherence to mucin
Non‐flagellated mutants are shown to have decreased virulence (Feldman et al. 1998; Montie et al. 1987)
Lipid A
Endotoxic properties
Binding to receptors on macrophages induces the production of inflammatory mediators including tumor necrosis factor α, interleukin (IL)‐1, IL‐6, IL‐8, and IL‐10 (Lynn and Golenbock 1992)
Lipopolysaccharide (LPS)
Adherence and invasion of epithelial cells
Two forms: capped/smooth with O antigen, and uncapped/rough without O antigen (Sadovskaya et al. 1998); uncapped/rough LPS of P. aeruginosa was important in mouse infection models for invasion of eyes (Zaidi et al. 1996) and airway respiratory epithelial cells (Pier et al. 1996)
O antigen
Serum resistance, resistance to phagocytosis
LD50 for O antigen‐deficient mutant was at least 1000‐fold higher than that of the wild‐type strain in a mouse burned‐wound model (Cryz et al. 1984). In neonatal mice, an LPS mutant was unable to initiate a respiratory tract infection whereas the wild‐type strain caused acute pneumonia, bacteremia and death (Tang et al. 1995)
Pili
Adherence to epithelial cells, mucin, colonization of tissues
Twitching motility is itself important for virulence; strains that have pili but not able to twitch were attenuated in virulence despite having the ability to associate with corneal epithelial cells as effectively as wildtype bacteria (Zolfaghar et al. 2003)
Virulence factor
Proposed biologic effects
Notes
Exotoxin A
(ADP)‐ribosyl transferase enzyme (like diphtheria toxin), cytotoxic, tissue invasion, cellular damage
LD50 of ExoA in mice is 0.2 μg/animal by the intraperitoneal route (Liu 1973) and 0.062 μg by the intravenous route (Callahan 1976)
Phospholipase C
Destruction of pulmonary surfactant, generation of inflammatory mediators
Toxic to animals at microgram amounts; causes vascular permeability, hepatonecrosis, renal tubular necrosis, end organ damage, and death in mice at high doses (Berk et al. 1987); (Meyers et al. 1992). Intradermal injection of sheep with PlcH causes superficial inflammatory lesions characteristic of fleece rot (Chin and Watts 1988)
Proteases: LasB and LasA elastase alkaline phosphatase
Damage to lung tissue, blood vessels; tissue damage, increased vascular permeability, disruption of respiratory cilia
Subcutaneous and intramuscular injections of LasB into mice cause severe hemorrhage and leakage of plasma components, including red and white blood cells into the extravascular tissue (Komori et al. 2001)
Rhamnolipid
Ciliostatic, impairs mucociliary clearance
Impair normal host respiratory clearance mechanisms by slowing down mucociliary clearance (Read et al. 1992)
Siderophores (pyochelin, pyocyanin, pyoverdin)
Acquisition of iron, enhances survival in low‐iron environments such as host tissues
Blue‐green of pyoverdin is responsible for characteristic “blue pus” of P. aeruginosa infected wounds (Meyer et al. 1996)
Type III secretion system
Cytotoxic, tissue damage and invasion, acute epithelial cell injury
Delivery system for several exoenzymes
Exoenzyme S (ExoS)
Inactivates GTP‐binding proteins of the H‐ and K‐Ras family and activates small‐molecular weight GTPases of the Rho subfamily and stimulates actin reorganization that results in disruption of actin microfilaments (Goehring et al. 1999)
ExoS causes cell injury and reduces proliferation and viability of host cells in vitro and is important for tissue damage and bacterial dissemination in animal studies (Kudoh et al. 1994; Nicas and Iglewski 1985)
Exoenzyme T (ExoT)
GTPase‐activating protein for Rho GTPase proteins and interferes with Rho transduction pathways which regulate actin organization, exocytosis, phagocytosis, and cell‐cycle progression (Krall et al. 2000; Sundin et al. 2001)
Recently, ExoT was shown to be necessary and sufficient to induce apoptosis in epithelial cells (Shafikhani et al. 2008)
Exoenzyme U (ExoU)
Lipase that requires activation or modification by eukaryotic factors (Sato et al. 2003)
ExoU and ExoS are apparently mutually exclusive, as no strains expressing both have been isolated, and those strains that do express ExoU are cytotoxic to a variety of mammalian cell types in vitro (Hauser et al. 1998)
Exoenzyme Y (ExoY)
Adenylate cyclase which induces elevation of intracellular cyclic adenosine monophosphate in vitro and in vivo (Yahr et al. 1998)
May provide a minor contribution to systemic spread in vivo (Vance et al. 2005)
Type V secretion system
Lipases that destroy of eukaryotic signaling lipids and lung surfactants; esterases that stimulate adhesion and biofilm formation (da Mata Madeira et al. 2016; Filloux 2011)
PlpD, a highly conserved type V secretion system toxin, is predicted to make an attractive vaccine target against P. aeruginosa (Chirani et al. 2018)
Type VI secretion system
Toxins which disrupt the cytoskeleton and promote non‐phagocytic internalization; endoplasmic reticulum attack stimulates host stress response and autophagy (Monjarás Feria and Valvano 2020)
Genetic analysis of the T6SS effector PldA suggests that the toxin was acquired horizontally from a eukaryotic source (Wilderman et al. 2001)
Pathogenesis
Stay updated, free articles. Join our Telegram channel

Full access? Get Clinical Tree
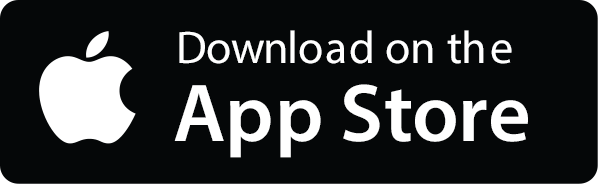
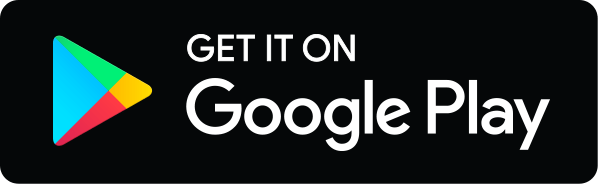