I. INTRODUCTION.
Pharmacology is the study of the properties of chemicals used as drugs for therapeutic purposes. It is divided into the study of pharmacokinetics and pharmacodynamics. Veterinary pharmacology focuses on drugs that are used in domestic animals. Pharmacokinetics is the study of drug absorption, distribution, biotransformation (metabolism), and excretion. Pharmacokinetic processes affect the route of administration, doses, dose intervals, and toxicities of drugs given to animals. Pharmacodynamics is the study of cell/tissue responses and selective receptor effects. In this chapter, we introduce standard concepts of pharmacokinetics and pharmacodynamics and comment on the need to be aware of species variation when considering principles of veterinary pharmacology.
II. DRUG ABSORPTION AND DISPOSITION
How do drugs reach their site of action? It is apparent from Figure 1-1 that a drug usually crosses several biological membranes from its locus of administration to reach its site of action and thereby produce the drug response. The manner by which drugs cross membranes are fundamental processes, which govern their absorption, distribution, and excretion from the animal.
If the drug is a weak acid use:

If the drug is a weak base use:

FIGURE 1-1. This diagram relates what may be expected to occur to a drug in the animal following its administration (IV, intravenous; IM, intramuscular; PO, per os or oral; IP, intraperitoneal; SC, subcutaneous; inhalation, dermal). (From Figure 1-1, NVMS Pharmacology.)

Examples: The macrocyclic lactones, ivermectin, and selamectin but less so with moxidectin, are excluded from the brain via P-glycoprotein. In some breeds of dog, particularly the Collies, P-glycoprotein is defective and ivermectin accumulates in the CNS, leading to toxicity.
Penicillin (a weak acid) concentrations in the CNS are kept low due to an active organic ion transporter system.
drug + protein (free) ⇋ Drug − protein (bound)
Acidic drugs are bound primary to albumin and basic drugs are bound primarily to α1-acid glycoprotein. Steroid hormones and thyroid hormones are bound by specific globulins, respectively, with high affinity.
FIGURE 1-2. Phases of biotransformation. (From Figure 1-2, NVMS Pharmacology.)

Example:
Acetylsalicylic acid | → | salicylate |
Inactive (aspirin) | active anti-inflammatory | |
febantel | → | fenbendazole/oxfendazole |
Inactive | active anthelmintic | |
Primidone | → | phenobarbital |
Inactive | active anticonvulsant | |
codeine | → | morphine |
active analgesic | more active analgesic |
A few chemicals are oxidized by cytosol or mitochondrial enzymes.
TABLE 1-1. Drug Conjugation Reactions
Conjugation Reaction | Drug Conjugated |
Glucuronidation | Aspirin, morphine, sulfadimethoxine, digitoxin, steroids, thyroxine, phenobarbital, phenytoin, chloramphenicol, phenylbutazone |
Acetylation | Sulfonamides, clonazepam, procainamide |
Glutathione formation | Ethacrynic acid |
Glycine formation | Salicylic acid, nicotinic acid |
Sulfate formation | Catecholamines, acetaminophen |
Methylation | Catecholamines, histamine |
FIGURE 1-3. Proximal renal tubule. Only drugs (D) which are free in the plasma are filtered. Once in the tubular lumen the drug may be passively reabsorbed. In the proximal renal tubule active transport mechanisms exist for secreting acid and base drugs (D) from the extracellular fluid into the renal tubule.

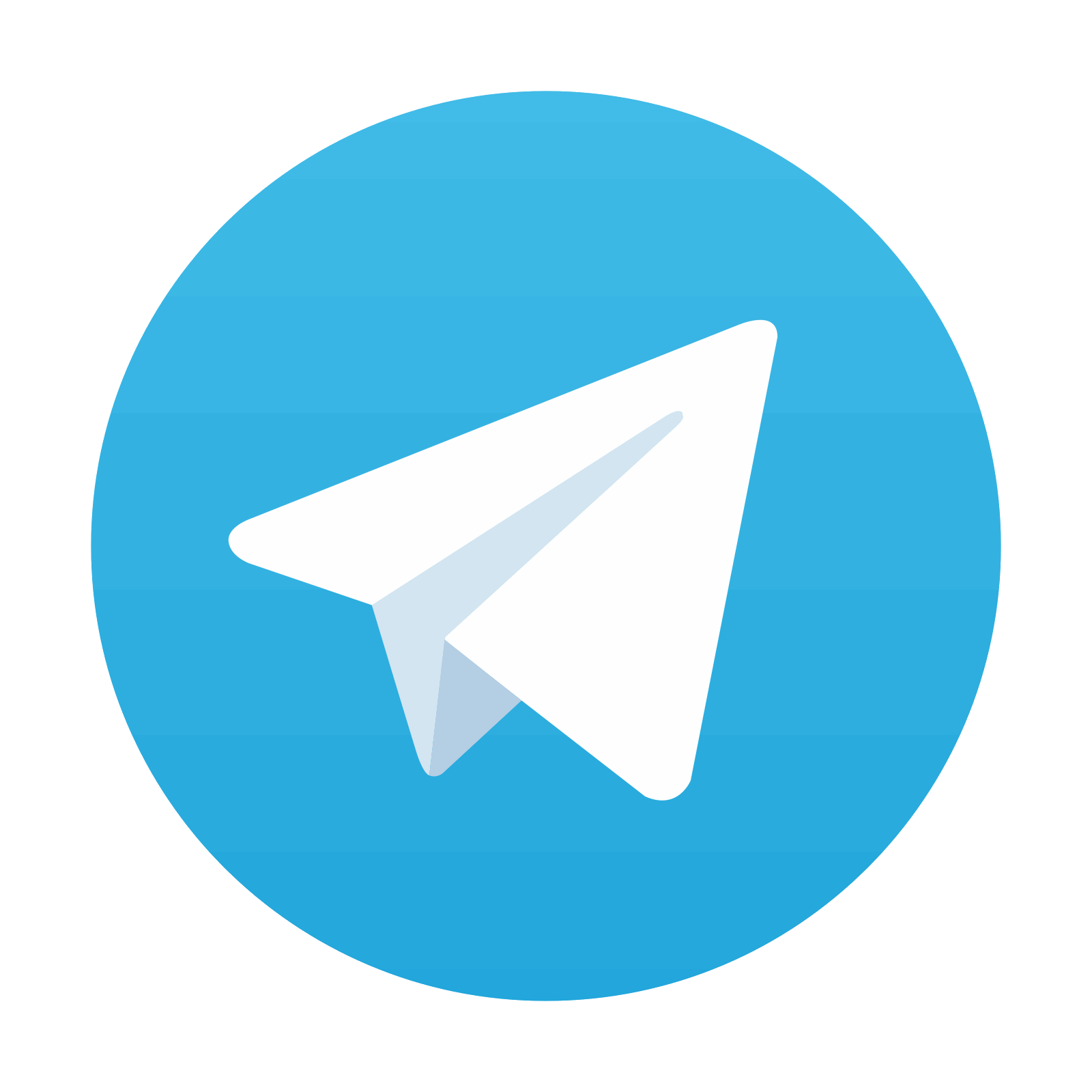
Stay updated, free articles. Join our Telegram channel

Full access? Get Clinical Tree
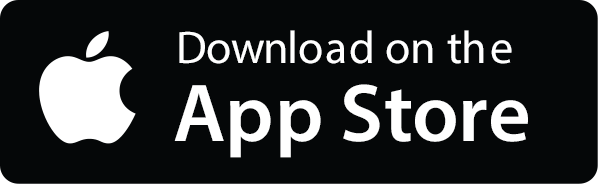
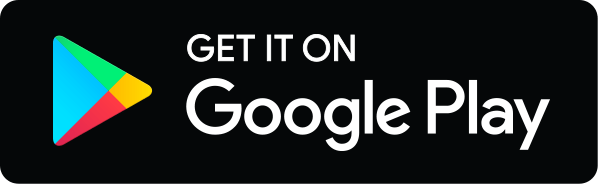