I. DRUGS USED IN HEART FAILURE THERAPY
A. Introduction
1. Heart failure occurs when abnormal cardiac function causes inadequate blood delivery to the tissues or adequate delivery only with elevated cardiac filling pressures. It can involve abnormalities of systolic or diastolic function, or both.
a. Congestive heart failure (CHF) is characterized by high cardiac filling pressure which leads to venous congestion (behind the affected side of the heart) and tissue fluid accumulation. It is a complex clinical syndrome rather than a specific etiologic diagnosis. Although poor contractility often underlies CHF, chronic cardiac overload (volume or pressure) or other injury can also stimulate a cascade of neurohormonal and cardiac responses that eventually lead to congestive signs and further deterioration in function.
(1) Left-sided congestive signs include pulmonary venous congestion and edema (resulting in cough, tachypnea, dyspnea, orthopnea, pulmonary crackles, tiring, hemoptysis, cyanosis). Chronic pulmonary congestion can lead to varying degrees of pulmonary hypertension and sometimes, right-sided CHF signs.
(2) Right-sided congestive signs include systemic venous hypertension, with resulting jugular venous distension, hepatic (and abdominal visceral) congestion, pleural effusion (resulting in dyspnea, orthopnea, cyanosis), ascites, small pericardial effusions, and sometimes, subcutaneous edema.
b. Forward (low output) failure causes tiring, exertional weakness, syncope, prerenal azotemia, and peripheral cyanosis (from poor cutaneous circulation). Cardiac arrhythmias frequently occur with all manifestations of heart failure. These signs can occur with either left or right heart disease.
2. Pathophysiology of heart failure
a. Cardiac remodeling involves changes in myocardial size, shape and stiffness that occur in response to various mechanical, biochemical, and molecular signals induced by the underlying injury or stress.
(1) Myocardial hypertrophy. Changes include myocardial cell hypertrophy, cardiac cell dropout (apoptosis), excess interstitial matrix formation, fibrosis, and abnormal collagen binding between individual myocytes. Increased mechanical forces (ventricular wall stress), as well as various neurohormones (e.g., angiotensin II, norepinephrine, endothelin, aldosterone) and cytokines (e.g., a tumor necrosis factor-α), are stimuli for cardiac remodeling. Myocardial hypertrophy helps normalize wall stress. Systolic pressure loads mainly cause “concentric” hypertrophy, with myocardial fiber thickening; volume loads mainly cause “eccentric” hypertrophy, with myocardial fiber elongation. Hypertrophy can interfere with diastolic function by making the ventricle “stiffer.”
(2) Frank–Starling mechanism. Acutely increased filling pressure and end diastolic volume (preload) induce greater force of contraction and help increase overall cardiac output. Valvular insufficiency, arterial hypertension, and ventricular outflow obstruction as well as volume retention lead to increased preload. Compensatory hypertrophy lessens the importance of the Frank– Starling mechanism in stable, chronic heart failure.
b. Neurohormonal mechanisms. Neurohormonal responses contribute to cardiac remodeling and have systemic effects. Excessive activation of neurohormonal “compensatory” mechanisms leads to the clinical syndrome of CHF.
(1) Increased sympathetic nervous tone. Short-term effects of increased contractility, heart rate, and venous return can increase cardiac output. But over time, increased afterload stress and myocardial oxygen requirement, contribute to cellular damage, myocardial fibrosis, and increased potential for cardiac arrhythmias.
(2) Activation of the renin–angiotensin–aldosterone system. Angiotensin II stimulates potent vasoconstriction and aldosterone secretion, and has other important effects. Aldosterone promotes Na+ and Cl− reabsorption in the kidney; it also contributes to pathologic remodeling and myocardial fibrosis.
(3) Antidiuretic hormone (vasopression). This hormone directly causes vasoconstriction and also promotes free water reabsorption.
(4) Other substances. Cytokines (e.g., TNF-α), endothelins, and other substances also play a role in abnormal myocardial hypertrophy and/or fibrosis in heart failure.
(5) Endogenous vasodilatory mechanisms. These oppose the vasoconstrictor responses in heart failure and include natriuretic peptides (atrial NP and brain NP, nitric oxide, and vasodilatory prostaglandins). Normally, a balance between vasodilator and vasoconstrictor effects maintains circulatory homeostasis. As heart failure progresses, the influence of the vasoconstrictor mechanisms predominates.
c. Pathophysiologic groups of heart failure. As an aid to choosing therapy, the causes of heart failure can be viewed according to major underlying pathophysiologic mechanism. Nevertheless, several pathophysiologic abnormalities usually coexist; both systolic and diastolic function abnormalities are common in advanced failure.
(1) Myocardial (systolic pump) failure. Dilated cardiomyopathy is the most common cause. Valvular insufficiency may or may not be present initially, but usually develops as the affected ventricle dilates.
(2) Volume overload. A leaky valve or abnormal systemic-to-pulmonary connections are common causes. Cardiac pump function is often maintained well initially, but myocardial contractility deteriorates over time.
(3) Systolic pressure overload. Congenital ventricular outflow obstruction, and systemic or pulmonary hypertension are common causes. Concentric hypertrophy increases ventricular wall thickness and stiffness, and predisposes to ischemia; eventually myocardial contractility declines.
(4) Reduced ventricular compliance with impaired filling (diastolic dysfunction). Examples include hypertrophic and restrictive myocardial disease and pericardial disease. Contractile ability is usually normal initially, but elevated filling pressure leads to congestion and may diminish cardiac output.
B. Overview of heart failure therapy
1. Treatment strategies are aimed at modifying either the results of neurohormonal activation (i.e., Na+ and water retention) or the activation process itself (e.g., angiotensin-converting enzyme inhibition). Goals are to control edema and effusions, improve cardiac output, reduce cardiac workload, support myocardial function, and manage concurrent arrhythmias. The clinical severity of heart failure influences the treatments used. Animals with heart failure caused by systolic dysfunction (e.g., dilated cardiomyopathy or advanced valvular insufficiency) benefit most from arteriolar vasodilation (reduced afterload) and also positive inotropic support.
a. Acute CHF. Acute CHF is characterized by severe cardiogenic pulmonary edema, with or without pleural and/or abdominal effusions or poor cardiac output. Therapy is aimed at rapidly clearing pulmonary edema, improving oxygenation, and optimizing cardiac output (see Table 8-1).
b. Chronic heart failure. Therapy is tailored to the individual’s needs by adjusting dosages, adding or substituting drugs, and modifying lifestyle or diet. Pleural effusion and large-volume ascites that accumulate despite medical therapy are drained to improve respiration. As heart disease progresses, more aggressive therapy is usually needed. Support of myocardial function with pimobendan (or digoxin) is helpful for many dogs, and some cats.
c. Treatment implications for diastolic dysfunction
(1) Hypertrophic cardiomyopathy (HCM). Disease such as HCM, which impairs ventricular filling, is treated with drugs that slow heart rate (to increase filling time and reduce ischemia). Improved cardiac relaxation is also a goal. Diltiazem (Ca2+ blocker), a β-adrenergic blocker and/or an angiotensin converting enzyme inhibitor (ACEI) are most commonly used.
(2) Cardiac tamponade. Impaired filling caused by cardiac tamponade or pericardial restriction is treated by pericardiocentesis or pericardiectomy rather than drugs.
C. Diuretics. Diuretic therapy is indicated to control edema and effusions that occur in CHF. Excessive use of diuretics in heart failure exacerbates neurohormonal activation. Diuretics are discussed in more detail in Chapter 9.
1. Furosemide. This loop-diuretic is used almost exclusively for animals with cardiogenic edema or effusion.
a. Acute CHF. Although aggressive furosemide therapy is indicated for acute, fulminant pulmonary edema, the smallest effective doses are used for chronic heart failure therapy.
b. Chronic heart failure. Furosemide should not be used as monotherapy for chronic heart failure management.
c. Adverse effects are usually related to excessive fluid and/or electrolyte losses.
2. Spironolactone. This K+-sparing diuretic may be a useful adjunct therapy for chronic refractory heart failure, although it appears to have little diuretic effect in normal dogs.
a. Aldosterone release can occur despite ACE inhibitor use. Spironolactone’s antialdosterone effect may mitigate aldosterone-induced cardiovascular remodeling.
b. Spironolactone must be used cautiously in patients receiving an ACEI or K+ supplement, and is absolutely contraindicated in hyperkalemic patients.
c. Adverse effects relate to excess K+ retention and GI disturbances. Spironolactone may decrease digoxin clearance.
3. Thiazide diuretics. Chlorothiazide or hydrochlorothiazide is occasionally used in combination with furosemide and other therapy for refractory CHF.
a. Thiazide diuretics decrease renal blood flow and should not be used in the presence of azotemia.
TABLE 8-1. Management of Acute Decompensated Congested Heart Failure*

D. Angiotensin converting enzyme inhibitors (ACEIs). This is a group of drugs that, by inhibiting the action of ACE, block the conversion of an inactive precursor peptide (angiotensin I) into the active angiotensin II. In this way, the effects of the renin– angiotensin–aldosterone cascade are opposed. ACE also degrades certain vasodilator kinins, including bradykinin. Most ACEIs (except captopril and lisinopril) are prodrugs which are converted to their active form in the liver. Severe liver dysfunction can interfere with this conversion.
1. Pharmacologic effects and mechanism of action
a. The main benefits of ACEIs arise from reducing the effects of neurohormonal activation (Figure 8-1).
b. Arteriolar and venous vasodilation
(1) Inhibition of locally produced ACE within vascular walls may produce a local vasodilatory response, even in the absence of high circulating renin levels.
(2) The vasodilating effects of ACEIs may be enhanced by vasodilatory kinins normally degraded by ACE.
c. Inhibition of angiotensin II production decreases aldosterone secretion, a hormone that promotes renal Na+ retention. ACEI’s diuretic effect is modest.
d. ACEIs may also (potentially) oppose abnormal CV remodeling changes.
2. Therapeutic uses and administration
a. An ACEI is indicated for the chronic management of most causes of chronic heart failure, especially dilated cardiomyopathy, chronic valvular insufficiency, and HCM.
b. An ACEI is considered the first choice agent in the management of systemic arterial hypertension in dogs. An ACEI may also be useful in cats with hypertension.
c. An ACEI may help slow progression of chronic renal failure in cats, and possibly dogs.
3. Adverse effects of ACEIs include hypotension, GI upset, renal function deterioration, and hyperkalemia (especially when used with a K+-sparing diuretic or K+ supplement).
a. Azotemia. If azotemia develops, the diuretic dosage is decreased first. If necessary, the ACEI dose is reduced or the drug discontinued.
4. ACEI used commonly for CHF management
a. Enalapril is the only ACEI licensed for veterinary use.
(1) Enalapril is well absorbed orally; bioavailability is not decreased by food.
(2) Enalapril is hydrolyzed in the liver to its most active form, enalaprilat.
(3) Peak ACE-inhibiting activity occurs within 4–6 hours in dogs. Duration of action is 12–14 hours; effects are minimal by 24 hours after once daily dosing.
(4) Maximal activity in cats occurs within 2–4 hours after an oral dose; some ACE inhibition (50% of control) persists for 2–3 days.
(5) Enalapril and its active metabolite are excreted in the urine.
(a) Significant adverse effects on renal function in dogs with advanced mitral regurgitation are generally not a concern.
(b) Renal failure and severe CHF prolong the drug’s elimination, so reduced dosage or benazepril are used in this situation.
b. Benazepril is often chosen in animals with preexisting renal disease. Benazepril may also slow renal function deterioration and partially mitigate hypertension in cats with renal disease.
(1) Oral administration is absorbed at rate of ~40%, which is not affected by feeding.
(2) Benazepril is metabolized to its active form (benazeprilat) in the liver.
(3) Peak ACE inhibition occurs within 2 hours of PO administration in dogs and cats. Complete ACE inhibition occurs in cats at doses of 0.25–0.5 mg/kg. There is >90% ACE inhibition at 24 hours.
(4) Repeated dosing moderately increases drug plasma concentrations.
(5) Benazepril is eliminated equally in urine and bile in dogs. In cats, ~85% is excreted in the feces and only 15% in the urine.
c. Other ACEIs sometimes used for CHF include captopril, lisinopril, ramipril, quinipril, fosinopril, and imidapril.
(1) Captopril is well absorbed orally (75% bioavailable), but feeding decreases bioavailability by 30–40%.
(a) Hemodynamic effects appear within 1 hour, peak in 1–2 hours, and last <4 hours in dogs.
(b) Captopril is excreted in the urine.
(2) Lisinopril is a lysine analog of enalaprilat with direct ACE-inhibiting effects.
(a) Lisinopril is 25–50% bioavailable; absorption is not affected by feeding.
(b) Time to peak effect is 6–8 hours. Once daily dosing appears to be effective.
FIGURE 8-1. The renin–angiotensin cascade and effects of angiotensin II. Angiotensin converting enzyme (ACE) catalyzes the conversion of angiotensin I to angiotensin II and degrades vasodilator kinins (e.g., bradykinin). Open arrows—enzymatic reaction.

E. Other vasodilators can further improve cardiac output and reduce edema and effusions in selected heart failure patients. Agents that reduce arteriolar resistance are also used in the treatment of hypertension. Vasodilators can affect arterioles, venous capacitance vessels, or both (“balanced” vasodilators).
1. Introduction
a. Arteriolar dilators decrease systemic vascular resistance, arterial blood pressure, and afterload on the heart by relaxing arteriolar smooth muscle. This helps improve forward cardiac output and can reduce (mitral) regurgitant flow, thereby decreasing left atrial pressure and pulmonary congestion. An arteriolar or mixed vasodilator drug should be initiated cautiously to avoid hypotension and reflex tachycardia.
b. Venodilators relax systemic veins, increase venous capacitance, decrease cardiac filling pressures (preload), and reduce pulmonary congestion. They are used mainly in treating acute CHF.
2. Hydralazine
a. Preparation and chemistry. Hydralazine (Apresoline®) is a phthalazine-derivative vasodilating agent.
b. Pharmacologic effects and mechanism of action. Hydralazine directly relaxes arteriolar smooth muscle with little effect on the venous system. Cerebral, coronary, splanchnic, and renal circulations are affected more than skeletal muscle or skin vasculature. Its effect is dependent on intact vascular endothelium.
c. Therapeutic uses and administration. Hydralazine, in combination with furosemide, is especially useful for dogs with mitral insufficiency and severe pulmonary edema by rapidly reducing arterial resistance (afterload). This helps reduce regurgitant volume and pulmonary venous pressure and increase forward cardiac output. Hydralazine has also been used in the treatment of myocardial failure and hypertension.
d. Pharmacokinetics. The drug is rapidly absorbed orally, with onset of action within 1 hour. Peak effect occurs within 3–5 hours and lasts up to 12 hours in dogs. There is extensive first-pass hepatic metabolism of this drug. However, in dogs, increased doses saturate this mechanism and increase bioavailability. A small amount of the drug is excreted unchanged in the urine. The t½ is 2–4 hours in people. The ClT is about 70 mL/min/kg and Vd is 9 L/kg; bioavailability is decreased by over 60% with food.
e. Adverse effects. Hypotension is most common. Hydralazine causes significant reflex tachycardia in some animals. The dose should be reduced if this occurs; sometimes adding digoxin or a β-blocker is also necessary. Hydralazine can exacerbate the increased neurohumoral response in heart failure, and enhance Na+ and water retention. GI upset may also occur, especially in cats.
3. Amlodipine
a. Preparation and chemistry. Amlodipine besylate (Norvasc®) is dihydropyridine Ca2+ channel blocker.
b. Pharmacologic effects and mechanism of action. Ca2+ channel blockers as a group block Ca2+ influx across cardiac and vascular smooth muscle cell membranes. Amlodipine mainly has vasodilating effects, with no appreciable cardiac effects.
c. Therapeutic uses and administration. Amlodipine is the drug of first choice for systemic arterial hypertension in cats. It can be a useful adjunct therapeutic agent in hypertensive dogs, and may be effective as a single agent in some. It also is used as adjunct therapy in dogs with refractory CHF (especially from mitral valve disease). It is administered PO.
d. Pharmacokinetics. Plasma t½ of amlodipine is ~30 hours in dogs, and maximal effects occur 4–7 days after initiating therapy. Oral bioavailability is high (88% in dogs) and peak plasma concentrations are reached 3–8 hours after oral administration; plasma concentrations increase with chronic therapy. The drug undergoes hepatic metabolism, but there is no extensive first-pass elimination; caution is warranted in animals with poor liver function. Excretion is through the urine and feces. Amlodipine’s effect on blood pressure lasts ≥24 hours in cats. Amlodipine generally does not have significant effects on serum creatinine concentration or body weight in cats with chronic renal failure.
e. Adverse effects. Hypotension is possible, but less likely than with hydralazine because of the slower onset of action. Infrequently, inappetence, azotemia, lethargy, hypokalemia, reflex tachycardia, or weight loss may occur.
4. Nitrates
a. Preparation and chemistry. Commonly used nitrates include sodium nitroprusside (for IV infusion), nitroglycerine topical ointment (2%), and oral isosorbide dinitrate.
b. Pharmacologic effects and mechanism of action. Nitrates cause peripheral vasodilation.
(1) Nitroprusside is a potent direct dilator of both arteriolar and venous smooth muscle; it reduces afterload as well as preload on the heart. The infused dosage should be titrated to maintain mean arterial pressure above 70 mm Hg.
(2) Nitroglycerin and isosorbide dinitrate act mainly on the venous system and reduce cardiac filling pressures (preload) by their venodilating effects. They are metabolized in vascular smooth muscle to produce nitric oxide, which indirectly mediates vasodilation via activation of guanylyl cyclase to produce cyclic GMP, which in turn activates protein kinase G to open K+ channels and close Na+ channels, thereby inducing hyperpolarization of the muscle.
c. Therapeutic uses and administration
(1) Sodium nitroprusside is mainly administered IV in the treatment of fulminant CHF; it is sometimes used for acute hypertensive crisis. This agent should only be used when arterial blood pressure and IV infusion rate can be constantly monitored.
(2) Nitroglycerin. The major indication for nitroglycerin is acute cardiogenic pulmonary edema. Nitroglycerin ointment (2%) is usually applied to the skin of the groin, axillary area, or ear pinna, although the efficacy of this in heart failure is unclear. An application paper or glove is used to avoid skin contact by the person applying the drug. Nitroglycerin ointment or isosorbide dinitrate are used occasionally in chronic CHF management, either combined with standard therapy for refractory CHF, or with hydralazine or amlodipine in animals that cannot tolerate ACEIs.
d. Pharmacokinetics
(1) Sodium nitroprusside’s effect on blood pressure last less than 10 minutes, so the drug must be given by intravenous infusion. Nitroprusside is metabolized to a cyanide radical, and then further metabolized in the liver; elimination is via the urine, feces, and exhaled air.
(2) Other nitrates. Because of extensive first-pass hepatic metabolism after oral administration, the transcutaneous route is used most often in animals for other nitrates, although nitroglycerine is also well absorbed sublingually. Nitroglycerine ointment (2%) is applied to the patient’s skin (usually the groin, axillary area, or ear pinna) every 4–6 hours using application papers or gloves. Onset of action is within 1 hour, with variable duration of effect (e.g., 2–12 hours). The t½ in dogs is unclear; it is <5 minutes in people, but metabolites have some activity. Dosage and absorption are variable. The self-adhesive, sustained-release preparations may be useful, but have not been systematically evaluated in small animals.
e. Adverse effects. Profound hypotension is the major side effect of sodium nitroprusside. Cyanide toxicity can result from excess or prolonged use (e.g., over 48 hours). Hypotension may result from excessive or inappropriate use of other nitrates as well. Chronic high dosages and frequent application or long acting formulations are most likely to be associated with the development of drug tolerance.
F. Positive inotropic drugs
1. Pimobendan (Vetmedin®)
a. Preparation and chemistry. Pimobendan is a benzimidazole–pyridazinone derivative, nonsympathomimetic, nonglycoside inotropic drug that also has vasodilating properties.
b. Pharmacologic effects and mechanism of action. This drug increases myocardial contractility by increasing myofilament sensitivity to Ca2+ and by inhibiting phosphodiesterase III (which breaks down cyclic AMP). The latter mechanism is responsible for pimobendan’s vasodilating properties. The drug also appears to have some anticytokine and antithrombotic properties.
c. Therapeutic uses and administration. Pimobendan is approved for use in dogs with CHF from dilated cardiomyopathy or chronic mitral valve disease. It is used in combination with diuretic and other therapy (sometimes including digoxin) as appropriate for the individual case. The total daily oral dose (0.5 mg/kg) is divided (not necessarily equally) and administered BID using a combination of whole and half chewable tablets (or capsules where available).
d. Pharmacokinetics. Pimobendan is metabolized in the liver to an active metabolite. Excretion is mainly through the feces. There is >90% protein binding of both drug and active metabolite. The elimination t½ for pimobendan and its metabolite are ~0.5 and 2 hours, respectively. There is a wide tissue distribution and there is a delay from the time of peak plasma concentrations to maximal effect on myocardial contractility (dP/dtmax). Increased dP/dtmax is observed for ≥8 hours after dosing. Inotropic effect may be attenuated by concurrent use of a β- or Ca2+-blocker.
e. Adverse effects. About a third of patients may experience reduced appetite, lethargy, diarrhea, and dyspnea, with fewer dogs exhibiting azotemia, weakness and other signs; however, these signs may be due to the underlying CHF. Sporadic mild increase in serum alkaline phosphatase has occurred, and hyperactivity, hemorrhage, drooling, constipation, and diabetes mellitus have been reported as suspected adverse reactions.
2. Digoxin
a. Preparation and chemistry. Digoxin is a cardiac glycoside. It is essentially the only digitalis glycoside still in clinical use.
b. Pharmacologic effects and mechanism of action
(1) Positive inotropic effect (Figure 8-2). Digoxin competitively binds to and inhibits Na+, K+-ATPase at the myocardial cell membrane. Decreased extracellular Na+ transport causes intracellular accumulation, which promotes Ca2+ entry via the Na+–Ca2+ exchange. The drug’s modest positive inotropic effect results from increased Ca2+ available to the contractile proteins.
(2) Antiarrhythmic effects. Digoxin also has some antiarrhythmic effects against supraventricular tachyarrhythmias which are mediated primarily via increased parasympathetic tone to the sinus and A-V nodes and atria. The drug also has some direct effects, which further prolong the conduction time and the refractory period of the A-V node.
(3) Digoxin also improves arterial baroreceptor sensitivity in heart failure, which helps counteract excessive neurohormonal activation.
c. Therapeutic uses and administration
(1) Digoxin is used for its modest positive inotropic effect. Digoxin has generally been used in the treatment of heart failure caused by or associated with myocardial failure, such as dilated cardiomyopathy and advanced, chronic pressure or volume overloads (e.g., aortic insufficiency, long-standing patent ductus arteriosus, mitral, or tricuspid insufficiency). However, this use is being supplanted by pimobendan.
(2) Digoxin is used for its antiarrhythmic effect against supraventricular arrhythmias. It is only moderately effective in slowing the ventricular response rate in atrial fibrillation and does not cause conversion to sinus rhythm.
(3) Digoxin is usually contraindicated in HCM, where it may worsen existing ventricular outflow obstruction; although it has been used when clinical signs of right heart failure develop. Digoxin is generally not useful for patients with pericardial disease. Digoxin is usually contraindicated when sinus or A-V nodal disease is present; it is relatively contraindicated with most serious ventricular arrhythmias because the drug may exacerbate them.
(4) Factors affecting dosage. Conservative doses should be used. Therapy is almost always begun using oral maintenance (rather than IV or loading) doses. There is only a weak correlation between digoxin dose and serum concentration in dogs with heart failure, indicating that other factors are important in determining the serum concentrations of this drug.
(a) Renal function. Serum digoxin concentrations are increased in dogs and cats with renal dysfunction because of reduced total body clearance and volume of distribution. There appears to be no correlation between degree of azotemia and serum concentration.
(b) Body condition. Since much of the drug is bound to skeletal muscle, animals with reduced muscle mass or cachexia, as well as those with compromised renal function can easily become toxic at the usual calculated doses. In addition, because digoxin has poor lipid solubility the dose should be based on the calculated lean body weight; this is especially important in obese animals.
(c) Serum concentration measurement. After 1 week of therapy (or dosage alteration) serum concentrations should be measured.
i The sample is drawn 8–10 hours after the previous dose. If serum concentration is <0.8 ng/mL, the digoxin dose can be increased by ≤30%, and the serum concentration measured the following week.
ii If serum concentration cannot be measured and toxicity is suspected, the drug should be discontinued for 1–2 days, then reinstituted at half of the original dose.
(d) Therapeutic serum concentration is considered to be between 0.8 and 2.0 ng/mL. This is reached within 2–4.5 days (with PO dosing every 12 hours) in dogs. In cats, therapeutic serum concentration is achieved with low doses given every 48 hours; concentrations are ~50% higher with the alcohol-based (less palatable) elixir than with tablets. In horses, serum concentrations of 0.5–2.0 ng/mL are achieved within 1–2 hours. Significant enterohepatic recycling may produce a second peak serum concentration in horses.
d. Pharmacokinetics
(1) Absorption is ~60% for the tablet form and 75% for the elixir. Absorption is decreased by food, kaolin–pectin compounds, antacids, and malabsorption syndromes. Steady state serum concentrations are achieved in ~7 days for dogs, in ~10 days for cats, and in 3–5 days for horses.
(2) Fate. About 27% of the drug in serum is protein bound. Elimination is mainly renal. There is minimal hepatic metabolism. Digoxin t½ is reported to be 23–39 hours in dogs, 25–78 hours in cats, and 13–23 hours in horses.
e. Adverse effects. Myocardial toxicity is of greater concern than GI toxicity. Fatal myocardial toxicity may occur before other signs develop, especially in patients with myocardial failure. P-R interval prolongation or signs of GI toxicity should not be used to guide progressive dosing of digitalis. Serum concentration measurement should be used to guide dosing. Loading doses should not be used in myocardial failure.
(1) Myocardial toxicity can cause almost any cardiac rhythm disturbance, including ventricular tachyarrhythmias, supraventricular premature complexes and tachycardia, sinus arrest, Mobitz type I second-degree A-V block, and junctional rhythms.
(a) Calcium overload. Diastolic sequestration and systolic release of Ca2+ may be impaired in diseased myocardial cells. Digoxin use can lead to cellular Ca2+ overload and electrical instability.
(b) Toxic levels of digoxin also increase sympathetic tone to the heart causing increased automaticity. In addition, the parasympathetic effects of slowed conduction and altered refractory period facilitate the occurrence of re-entrant arrhythmias.
(c) Digoxin also can stimulate spontaneous automaticity of myocardial cells by inducing and potentiating late after-depolarization; this is enhanced by cellular stretch, calcium overloading, and hypokalemia.
(2) GI toxicity. GI upset is the other major toxicity and may occur before signs of myocardial toxicity develop, especially in patients without myocardial failure. Its signs include anorexia, depression, vomiting, borborygmus, and diarrhea. Direct effects of digitalis on chemoreceptors in the area postrema of the medulla may be the cause of some of the GI signs (e.g., vomiting).
(3) Factors predisposing to digoxin toxicity
(a) Hypokalemia predisposes to myocardial toxicity by leaving more available binding sites on membrane Na+, K+-ATPase for digitalis.
(b) Renal dysfunction (see above)
(c) Hypercalcemia and hypernatremia potentiate both the inotropic and toxic effects of the drug.
(d) Abnormal thyroid hormone levels can lead to digitalis toxicity (hyperthyroidism increases the drug’s myocardial effects and hypothyroidism reduces its clearance); therefore, dosage reduction may be needed.
(e) Hypoxia sensitizes the myocardium to the toxic effects of digitalis.
(f) Certain drugs increase digoxin serum concentration. Quinidine displaces the drug from skeletal muscle binding sites and reducing its renal clearance. Verapamil and amiodarone also can increase serum digoxin concentration; other drugs possibly do as well (including diltiazem, prazosin, spironolactone, and triamterene). Drugs affecting hepatic microsomal enzymes may also have effects on digoxin metabolism. Neomycin and sulfasalazine decrease bioavailability.
(4) Therapy for digitalis toxicity depends on the signs manifested.
(a) GI signs usually respond to drug withdrawal and correction of fluid or electrolyte disturbances.
(b) Atrioventricular conduction abnormalities also usually resolve with drug withdrawal, although sometimes an anticholinergic agent is needed.
(c) Ventricular tachyarrhythmias in dogs are treated with lidocaine or phenytoin.
i Other therapy includes IV K+ supplementation (if serum K+ is <4.0 mEq/L), IV fluid to correct dehydration and maximize renal function, and in some cases, propranolol to help control ventricular tachyarrhythmias (as long as no conduction blocks are present).
ii Oral administration of the steroid-binding resin cholestyramine is only useful very soon after accidental overdosage of digoxin, since this drug undergoes minimal enterohepatic circulation. A preparation of digoxin-specific antigen binding fragments (Digoxin immune Fab; Digibind®) derived from ovine antidigoxin antibodies have been used occasionally for digoxin overdose in dogs.
3. Dobutamine and dopamine (sympathomimetic agents). See Chapter 2 for more information.
a. Preparation and chemistry. Dopamine (Intropin®) is an endogenous catecholamine that is a precursor to norepinephrine. Dobutamine (Dobutrex®) is a synthetic analog of dopamine.
b. Pharmacologic effects and mechanism of action. Catecholamines increase contractility and heart rate, especially at higher doses. Stimulation of cardiac β1-adrenergic receptors (coupled to Gs) activates adenylyl cyclase to increase cyclic AMP synthesis, which stimulates a protein kinase A that in turn activates Ca2+ channels, leading to increased Ca2+ influx and greater contractility.
(1) Dopamine stimulates β- and α-receptors at higher doses. Peripheral vasoconstriction occurs at 10–15 mcg/kg/min. At low doses (less than 2–5 mcg/kg/min IV infusion), it also stimulates vasodilator dopaminergic receptors in the renal, mesenteric, coronary, and cerebral circulations.
(2) Dobutamine stimulates β1-receptors, but has only weak action on β2– and α-receptors; it does not stimulate dopaminergic receptors. The drug increases contractility, with minimal effects on heart rate and blood pressure at lower infusion rates (3–7 μg/kg/min).
c. Therapeutic uses and administration
(1) Myocardial failure. Dopamine and dobutamine are used, in conjunction with other therapy, for short-term inotropic and blood pressure support in dogs and cats with myocardial failure. Use of catecholamines for heart failure is limited by the development of β-receptor downregulation. Generally these drugs are given for no more than 3 days.
(2) Hypotension that is not responsive to fluid loading.
(3) Dopamine is also used to increase renal blood flow in acute oliguric renal failure.
d. Pharmacokinetics. The very short t½ (less than 2 minutes) and extensive hepatic metabolism of the catecholamines used clinically makes them suitable only for IV administration, usually by constant infusion.
e. Adverse effects. At higher doses, dopamine and dobutamine increase heart rate, myocardial oxygen demand, and the risk of inducing ventricular arrhythmias. Development of sinus tachycardia or tachyarrhythmias should prompt a decrease in infusion rate.
(1) Dobutamine is less arrhythmogenic than other catecholamines, but may precipitate supraventricular and ventricular arrhythmias at higher infusion rates (10–20 mcg/kg/min). Cats are more sensitive to dobutamine than dogs and may exhibit seizures or other adverse effects at relatively low dosages.
(2) By increasing renal blood flow, dopamine may enhance the renal clearance of other drugs.
4. Amrinone and Milrinone (phosphodiesterase inhibitors)
a. Preparation and chemistry. Amrinone is also known as inamrinone. Amrinone and milrinone are bipyridine cardiac inotropic agents.
b. Pharmacologic effects and mechanism of action. These agents inhibit phosphodiesterase III, an intracellular enzyme that degrades cAMP. They produce vasodilation as well as an increase in myocardial contractility.
c. Therapeutic uses and administration. Amrinone and milrinone are sometimes used for short-term inotropic support in dogs and cats with severe myocardial failure. The drugs are available for IV injection. They can be used concurrently with other types of inotropic agents.
d. Pharmacokinetics. Effects begin within 3 minutes and peak in 10 minutes after IV bolus injection, but are short-lived in normal dogs (<30 minutes). Constant infusion is required for sustained effect. Peak effect using constant rate infusion occurs in ~45 minutes in dogs.
e. Adverse effects
(1) These agents may exacerbate ventricular tachyarrhythmias.
(2) Higher dosages result in greater vasodilation, with reduction of blood pressure and increases in heart rate.
(3) Other adverse effects could include vomiting/diarrhea, hepatotoxicity, thrombocytopenia (with prolonged use).
FIGURE 8-2. Digoxin mechanism of action in myocardium. Digoxin-induced inhibition of Na+, K+-ATPase leads to an increase in myocardial Na+ concentration. Through Na+–Ca2+ exchanger, an increase in Ca2+ influx is reached. The increase in myocardial Ca2+ concentrations enhances the muscle contractility.

G. Drugs used in heart disease causing diastolic function. Agents that increase diastolic filling time, reduce myocardial O2 requirements, and/or facilitate myocardial relaxation are used in the treatment of HCM and other causes of severe hypertrophy.
1. β-Blockers such as atenolol (propranolol, metoprolol, and others) are often used to slow heart rate and reduce myocardial O2 consumption. See II D and Chapter 2 for more information.
a. Certain β-blockers (e.g., carvedilol, metoprolol) may also be useful in the long-term management of dilated cardiomyopathy and chronic valve disease, but further study is needed.
2. Calcium entry blockers, as a group, cause coronary and systemic vasodilation, enhanced myocardial relaxation, and sometimes, reduced cardiac contractility. Diltiazem has been used commonly in cats with HCM (see II D for additional information).
3. ACE inhibitors (e.g., enalapril, benazepril) are being increasingly used in the management of CHF from HCM (see above and also Chapter 3).
II. ANTIARRHYTHMIC DRUGS
A. Introduction
1. Multiple factors underlie the development of cardiac rhythm disturbances.
a. Both overt and subclinical changes in cardiac structure or function can alter cell electrophysiologic characteristics in ways that predispose to arrhythmia formation.
(1) Changes in normal cellular conduction properties or automaticity caused by cardiac structural or physiologic remodeling can predispose to arrhythmia development. Genetic factors and environmental stresses can contribute.
(2) Additional triggering (e.g., premature stimulus or abrupt change in heart rate) and/or modulating factors (e.g., changes in autonomic tone, circulating catecholamines, ischemia, or electrolyte disturbances) also appear necessary to provoke and sustain rhythm disturbances.
b. Abnormal rhythms, as well as normal sinus node activity, are influenced by the autonomic nervous system. Many conditions affect prevailing autonomic tone.
2. In general, underlying mechanisms are categorized as disorders of impulse formation, disorders of impulse conduction, or combinations of both. Identifying the specific mechanism for an arrhythmia in the individual patient is often difficult.
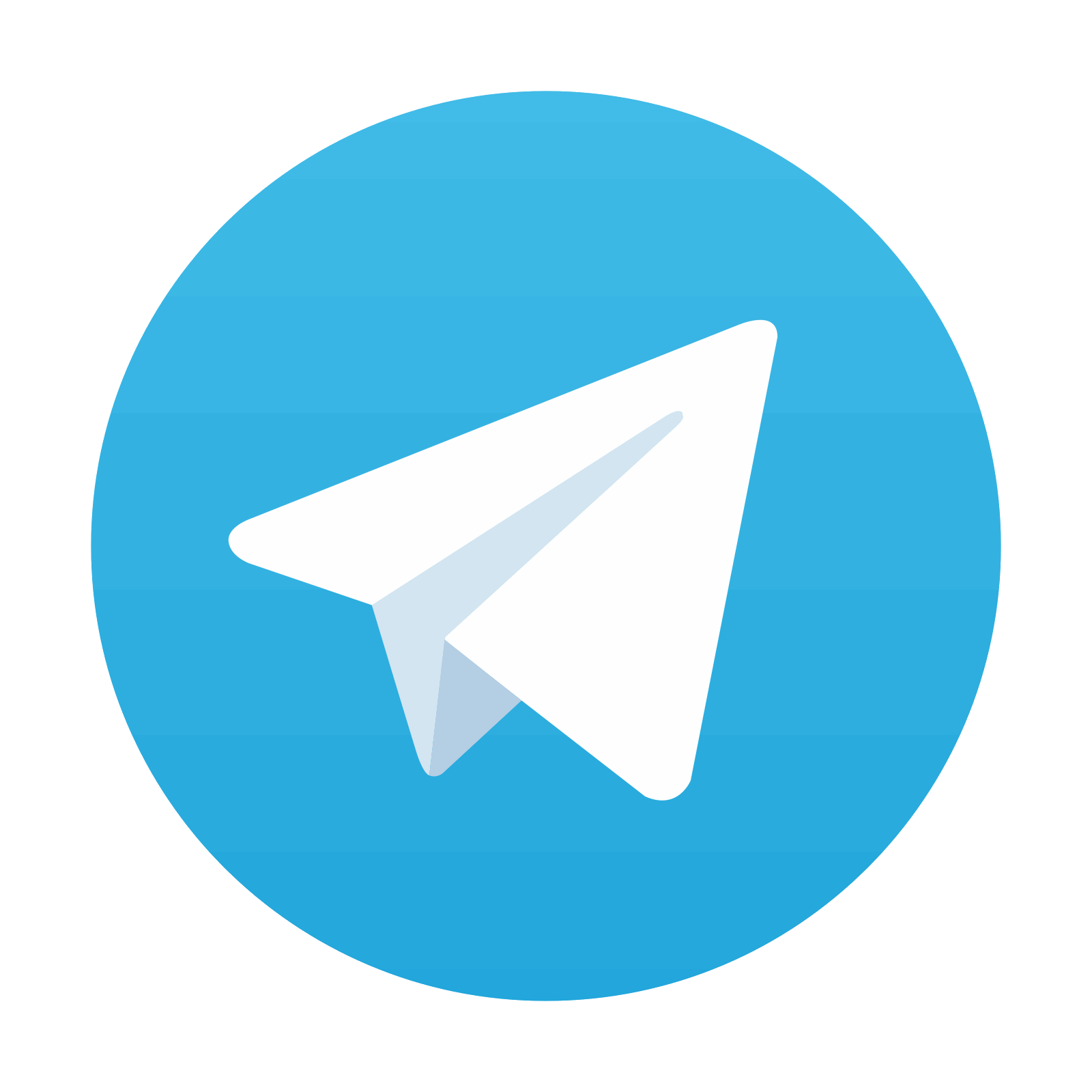
a. Disorders of impulse conduction can cause bradyarrhythmias when conduction fails in the AV node, atria, or SA node, causing asystole or a slow escape rhythm.
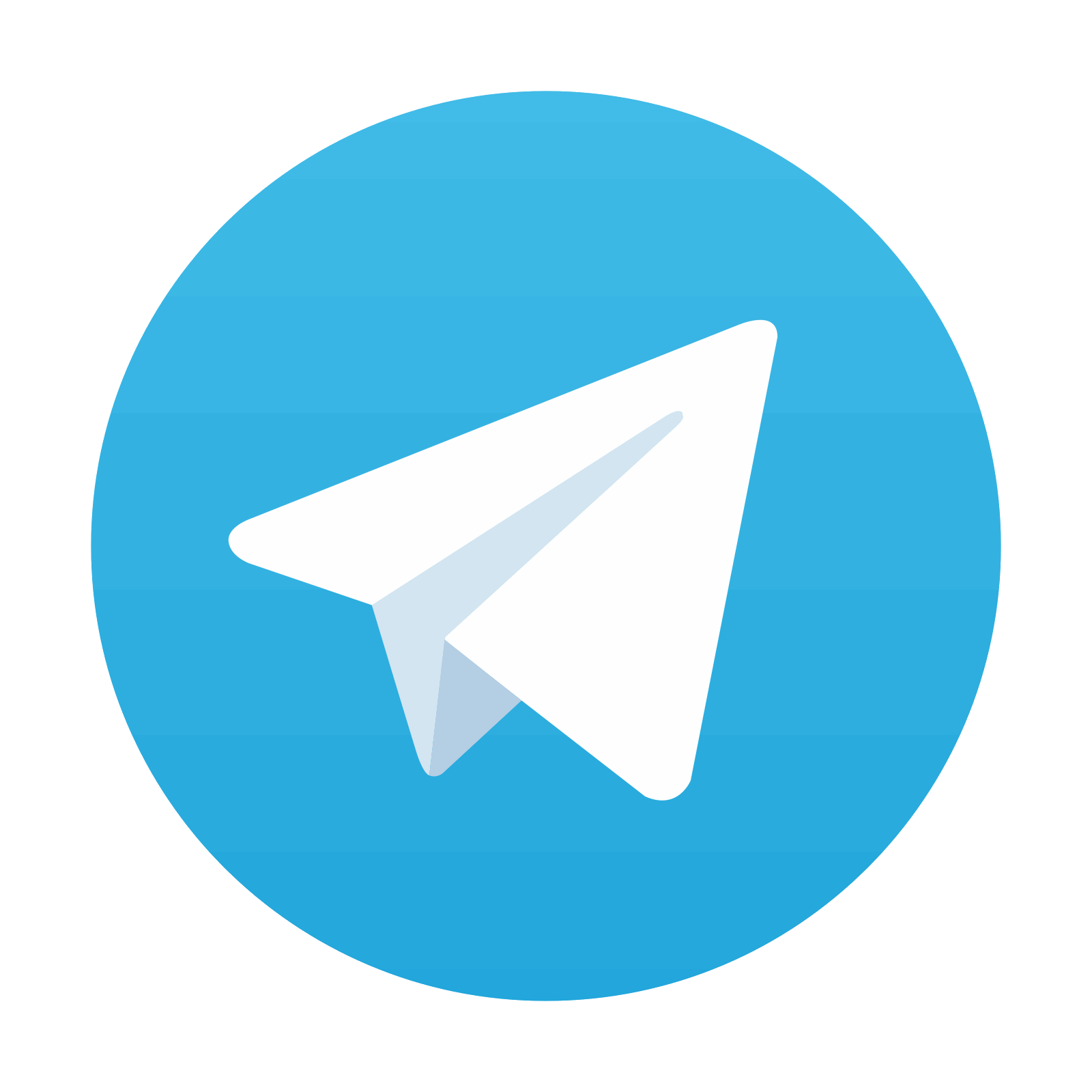
Stay updated, free articles. Join our Telegram channel

Full access? Get Clinical Tree
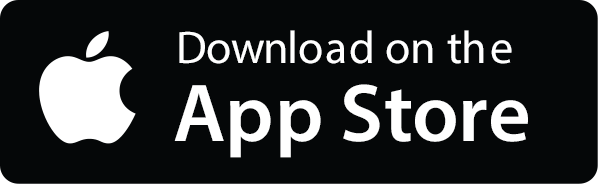
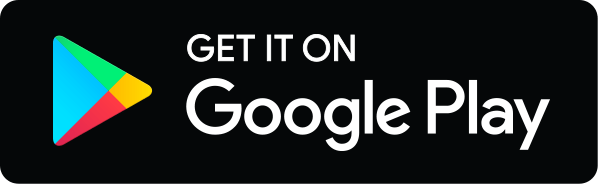