Chapter 6 • The use of antimicrobial drugs (AMDs) can lead to selection of resistant bacterial populations, within the targeted bacterial population and also other commensal bacteria. • Bacterial mechanisms of drug resistance include production of enzymes that inactivate AMDs, drug exclusion through membrane alterations and efflux pumps, and modification of the site of action of the AMD. • Strategies that minimize selection for drug resistance include (1) confirmation of the presence of infection before AMD treatment is commenced, (2) proper identification of the infecting organism, (3) selection of agents with activity specific to the pathogen present, (4) administration of an adequate dose of AMD, and (5) administration of AMD for the proper duration of time. • Selection of an appropriate AMD should be based on the activity of the agent against the suspected pathogen; knowledge of drug pharmacokinetics, which includes bioavailability and tissue penetration; consideration of host factors such as concurrent illness, medications, or immunosuppression; and knowledge of drug pharmacodynamics, that is, whether the drug exhibits concentration- or time-dependent antibacterial effects. Antibiotics have been considered one of the greatest inventions of the 20th century. The modern era of antimicrobial drug (AMD) treatment began with the discovery of sulfonamides in 1935.1 In the 1940s, the therapeutic value of penicillin and streptomycin was discovered, and by 1950, the “golden age” of AMD therapy was underway. With the increasing use of AMDs, there has been a transition to an era of widespread antimicrobial resistance among important veterinary and human pathogens. This has been compounded by a declining rate of development of new classes of AMDs. Guidelines for prudent antimicrobial use have been published by several professional veterinary organizations in order to reduce selection for resistant bacterial pathogens.2–6 Individual hospitals are encouraged to develop antimicrobial guidelines based on evaluation of their local prevalence of AMD resistance. Strategies that minimize selection for antimicrobial resistance include documentation of the presence of infection; proper identification of the infecting organism; and the use of agents that are as specific for the pathogen as possible, at the proper dose and for the proper duration of time. These strategies are described in more detail in this chapter. The detection of fever or leukocytosis does not imply bacterial infection and the need for antibiotic treatment, especially given the relatively high prevalence of sterile inflammatory and immune-mediated diseases in dogs and viral infections in cats. Selection of the most appropriate AMD relies on knowledge of (1) the presence of an infectious agent, (2) the type of infectious agent present, and (3) the susceptibility of the agent to different AMDs. In some cases, treatment with antibiotics may not be necessary even though the cause of disease is bacterial. Some infections undergo spontaneous cures without the aid of an antibiotic. For example, a cat bite abscess can often be drained and resolved without the need to administer an antibiotic (see Chapter 57). In some situations, collection of a specimen for cytology or histopathology permits a diagnosis of infection to be established. The identity of some infectious agents, such as rickettsial and fungal pathogens, may be presumptively identified to the genus or species level based on their morphology. If skin cytology from a dog reveals cocci (a Gram stain is not necessary to confirm the presence of gram-positive cocci), then the presence of Staphylococcus pseudintermedius is highly probable. If, on the other hand, cytology from an ear swab reveals rods, the possibility of Pseudomonas aeruginosa must be considered. In either case, this knowledge is valuable for selecting initial antibacterial therapy. The use of molecular diagnostic techniques, such as PCR assays, can also allow rapid identification of an infecting organism, which can help to guide antimicrobial drug selection. For example, identification of a vector-borne pathogen (e.g., Rickettsia spp., Ehrlichia canis) is helpful because doxycycline is usually the first drug of choice for the majority of these pathogens. Unfortunately, unless susceptibility to a particular AMD is predictable, these techniques do not typically provide information about AMD susceptibility. Given the emergence of widespread AMD resistance, especially among bacteria and some fungi, collection of specimens for culture and susceptibility before treatment with AMDs is commenced has become more and more important. Although there is an initial cost to the client for culture and susceptibility testing, long-term costs to the client may be reduced considerably because of decreased prescription of unnecessary or inappropriate AMDs, improved likelihood of rapid resolution of the underlying disorder with early diagnosis and appropriate treatment, and reduced need for more expensive, second- or third-line AMDs. Administration of AMDs without identification of the infectious agent can also decrease subsequent chances of successful culture and proper diagnosis if the disorder does not resolve. Antimicrobial drugs may be classified based on their structure (e.g., β-lactams, fluoroquinolones), spectrum of activity (gram-positive versus gram-negative bacteria), mechanism of action (Table 6-1, Figure 6-1), whether they are bacteriostatic (inhibit or slow growth) or bactericidal drugs, and their pharmacodynamic properties (see Pharmacodynamics of Antimicrobial Drugs, later). Bactericidal drugs interfere with cell wall or nucleic acid synthesis, whereas bacteriostatic drugs inhibit protein synthesis or cause changes in bacterial physiology. When a bacteriostatic drug is removed, in the absence of host defense mechanisms, organism growth resumes, and any decline in bacterial numbers that occurs when the drug concentration drops below the minimum inhibitory concentration (MIC) reflects clearance by the host immune response. However, the line that separates bactericidal and bacteriostatic is not as precise as previously thought. Some bacteriostatic drugs become bactericidal when present in high concentrations at the site of infection, or if concentrations are maintained above the MIC for the entire dosing interval. Even macrolides and chloramphenicol, which are traditionally considered as bacteriostatic drugs, can be bactericidal against some organisms. A drug may be bactericidal against gram-positive cocci, but bacteriostatic against gram-negative bacilli. Therefore, from a clinical perspective, it is more useful to describe drugs as time versus concentration-dependent, rather than as bactericidal or bacteriostatic. This allows the clinician to consider the dosage regimen and dosing interval to optimize therapy. There are rare instances where a bactericidal agent is preferred over a bacteriostatic one. It may be preferable to prescribe a bactericidal drug to treat life-threatening infections (such as vegetations in endocarditis or in a systemically immunocompromised host).7 TABLE 6-1 Classification of Antimicrobial Drugs Based on Their Mechanism of Action Antibacterial drugs have also been classified for veterinary use as first-line, second-line, or third-line drugs.8 First-line drugs are those that could be used for empirical selection in the absence of or pending the results of culture and susceptibility testing, and include amoxicillin, cephalexin, doxycycline, and trimethoprim-sulfonamides. Second-line drugs are those to be used on the basis of culture and susceptibility testing and because of the lack of any appropriate first-line options. These include ticarcillin, piperacillin, amikacin, and third-generation cephalosporins. Fluoroquinolones were also included in this group because in human medicine, excessive fluoroquinolone use has been associated with emergence of antimicrobial resistance and treatment failures.9 Fluoroquinolones could be considered as first-line drugs for dogs and cats suspected to have serious gram-negative bacterial infections that require treatment pending the results of culture and susceptibility testing. The use of third-line drugs, including vancomycin, linezolid, and carbapenems such as imipenem and meropenem, is usually reserved for situations when certain criteria are met8: 1. Infection must be documented based on clinical abnormalities and culture. 2. The infection is serious and has the potential to be life-threatening if left untreated. 3. Resistance is documented to all other reasonable first- and second-line options. 4. The infection is potentially treatable. 5. The clinician may seek advice from an infectious disease clinician or a clinical microbiologist to discuss antimicrobial susceptibility test results, and to discuss the use of these agents if there is unfamiliarity with their use. In some instances, there may be other viable options (e.g., topical therapy). Pharmacokinetic and pharmacodynamic parameters (PK-PD exposure relationships) are important determinants of the efficacy of AMDs and serve as the basis for determination of clinically effective dosage regimens and susceptibility breakpoints and for the development of guidelines for AMD use for specific types of infections.10 The killing of microbes by AMDs may be classified as concentration dependent or time dependent. AMDs that exhibit concentration-dependent killing are fluoroquinolones and aminoglycosides. These drugs work optimally when the peak concentration in the plasma (Cmax), or the area under the curve (AUC) exceeds the MIC by a defined factor (Figure 6-2). These are typically bactericidal agents and the ability to kill bacteria increases as the AMD concentrations increase. Target concentrations have been defined for laboratory animals and humans and have been extrapolated to veterinary patients. For example, the peak concentration to MIC ratio (Cmax/MIC) should exceed 8 to 10 for optimum dosing of aminoglycosides. The AUC for a 24-hour interval to MIC ratio (AUC24/MIC) should exceed 100 for fluoroquinolones. For some infections, a ratio of 125 is better, and in some patients (e.g., immunocompromised patients with life-threatening infections), a ratio of 250 may be desirable. Drugs that exhibit concentration-dependent killing also have postantibiotic effect (PAE). The PAE is the persistence of antimicrobial effects after drug concentrations at the site of infection fall below the MIC. The exact cause of the PAE varies with the drug, but it may result from irreversible binding of a drug to the target site. The duration of the PAE is influenced by the duration of antibiotic exposure, the drug concentration, the bacterial species present, and the class of antibiotic. For concentration-dependent antibiotics, administration of the total daily dose as a single dose every 24 hours is preferred to a smaller divided dose, in order to maximize Cmax or AUC. For aminoglycosides, this also reduces drug toxicity.7 FIGURE 6-2 A, Antimicrobial drug (AMD) pharmacodynamics. Concentration-dependent drugs are most active when the peak concentration (Cmax), or the area-under-the-curve (AUC) in the plasma exceeds the MIC by a defined factor. For time-dependent drugs, outcome is correlated with the time that the AMD concentration at the site of infection spends above the MIC. This is usually expressed as the percent of time above the MIC during a 24-hour interval (T > MIC). B, As AMD concentrations increase, the ability of concentration-dependent drugs such as fluoroquinolones (in this case, ciprofloxacin) to kill bacteria increases. As concentrations of time-dependent drugs such as β-lactam drugs (in this case, ticarcillin) increase, bacterial killing plateaus. MIC, minimum inhibitory concentration.
Principles of Antiinfective Therapy
Introduction
Identification of the Infecting Organism
Classification of Antimicrobial Drugs
Mechanism of Action
Antimicrobial Drug
Examples
Inhibition of cell wall synthesis
β-Lactams, glycopeptides
Penicillin, cephalexin, meropenem, vancomycin
Protein synthesis inhibition
Tetracyclines, aminoglycosides, chloramphenicol, lincosamides, macrolides
Doxycycline, gentamicin, erythromycin, clindamycin, azithromycin
Inhibition of DNA replication
Fluoroquinolones
Enrofloxacin, marbofloxacin
Inhibition of folic acid metabolism
Trimethoprim-sulfonamides
Trimethoprim-sulfamethoxazole
Pharmacodynamics of Antimicrobial Drugs
Stay updated, free articles. Join our Telegram channel

Full access? Get Clinical Tree
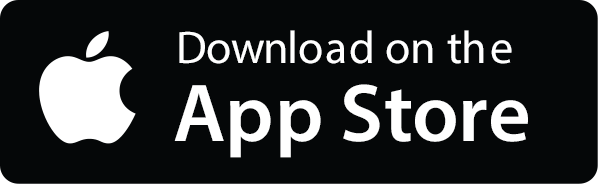
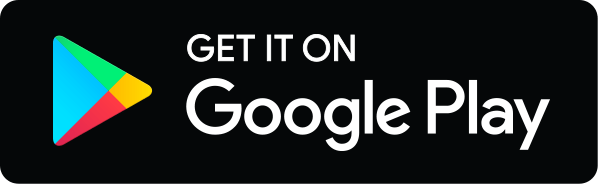