Chapter 8 Porphyrins and the Porphyrias A. Structure of the Porphyrins B. Synthesis of Porphyrins and Heme D. Porphyrinurias (Acquired Toxic Porphyrias) The metal-porphyrin complexes are found widespread in nature as constituents of compounds of fundamental importance in the metabolic processes of life. The photosynthetic pigment of plants, chlorophyll, is a magnesium porphyrin. The iron-porphyrin complexes of animals are found as prosthetic groups of proteins, including the hemoglobins, myoglobins, and the heme enzymes peroxidase, catalase, and the cytochromes. The porphyrins also exist in nature in their free state or as zinc complexes, and it is this group that is associated with the porphyrias and the porphyrinurias. Present knowledge of the porphyrins has its basis in the classic studies of the German physician and chemist Hans Fischer, whose work on the porphyrins dates back to 1915. The development of elegant methods of detection and identification of porphyrins and sophisticated enzymological techniques have resulted in the present clear understanding of the mechanisms of porphyrin biosynthesis and the biochemical and molecular bases for the disorders of porphyrin metabolism. The parent nucleus of the porphyrins is a cyclic tetrapyrrole, which consists of four pyrrole nuclei with their α(adjacent to the β) carbon atoms linked together by methene (-C=) bridges. This compound is called porphin and is shown in Figure 8-1. The various synthetic and naturally occurring porphyrins are derivatives of porphin, distinguished from each other by the type and position of the radicals substituted for the hydrogen atoms at positions 1 through 8. For convenience in discussing the substitutions, the simplified representation of the porphin nucleus as shown in Figure 8-1 is used. The classification of the porphyrins is based on the synthetic porphyrin, etioporphyrin (ETIO), in which two different radicals are substituted at positions 1 through 8. The substituted radicals are four methyl (M) and four ethyl (E) groups. The number of structural isomers possible with these eight substituted radicals is four, as shown at the top of Figure 8-2. The naturally occurring porphyrins are only those in which the positioning of their substituted radicals correspond to isomer I or III of etioporphyrin, ETIO I and ETIO III. This observation led Fischer to speak of a “dualism” of porphyrins in nature, which is in essential agreement with present knowledge of the biosynthesis of the porphyrin isomers as proceeding along parallel and independent paths. The uroporphyrins also contain two different radicals, acetic (A) and propionic (P) acids, and four each of these are arranged to correspond to either isomer ETIO I or ETIO III (Fig. 8-2). In this case, A corresponds to M and P corresponds to E. Therefore, these are designated uroporphyrin I (URO I) or uroporphyrin III (URO III). Similarly, the coproporphyrins contain four M and four P groups and are designated coproporphyrin I (COPRO I) and coproporphyrin III (COPRO III). The protoporphyrin of heme (iron-protoporphyrin, the prosthetic group of hemoglobin) corresponds to the series III isomer. In this case, however, three different radicals instead of two are substituted. These consist of four M, two P, and two vinyl (V) radicals. With three different radicals, 15 isomers are possible, but the protoporphyrin of heme is the only naturally occurring isomer known. This isomer was designated protoporphyrin IX because it was the ninth in the series of protoporphyrin isomers synthesized by Fischer. The arrangement of the methyl groups of this isomer as shown in Figure 8-2 corresponds to that of a type III etioporphyrin isomer and more properly should be called protoporphyrin III. However, by convention, the name protoporphyrin IX (PROTO IX) is the designation for this porphyrin. FIGURE 8-1 The precursor pyrrole and the parent porphin nucleus of porphyrins. Sites of isomeric substitutions are given as circled numbers and the pyrrole rings as letters. A schematic representation is also given. FIGURE 8-2 The isomeric porphyrins. The nomenclature of the porphyrins URO, COPRO, and PROTO is based on the isomeric structure of the etioporphyrins. Note that there are only the type I and type III isomers. An interesting isomer with only one vinyl group located on the A ring occurs and is known as harderoporphyrinogen, which is thought to be an intermediate in the synthesis of protoporphyrinogen III. Other naturally occurring or chemically synthesized porphyrins are derivatives of PROTO IX. If the two vinyl groups are hydrogenated to ethyl groups, the product is mesoporphyrin IX. If the two vinyl groups are converted to hydroxy-ethyl groups, the product is hematoporphyrin IX. If the two vinyl groups are replaced by hydrogen atoms, the product is deuteroporphyrin IX. Protoporphyrin and deuteroporphyrin normally occur in feces but are primarily products of intestinal bacterial degradation. The initial steps in the pathway for porphyrin and heme biosynthesis begins with the incorporation of the methyl carbon (C-2) and nitrogen atom of glycine into the porphyrin ring and ultimately into the heme of hemoglobin. Porphyrin metabolism and the porphyrias have been periodically reviewed (Elder and Sheppard, 1982; Hindmarsh, 1986; Sassa, 2006; Sassa and Kappas, 2000). The methyl carbons (C-2) of glycine supply 8 of the 34 carbons of protoporphyrin: one for each of the four methene bridges and one for each of the pyrrole rings (Fig. 8-3). The carboxyl carbon atom of glycine is given off as CO2 and is not incorporated into the protoporphyrin molecule (Fig. 8-3). The direct incorporation of the nitrogen or the methyl carbon glycine into the heme of hemoglobin has been the basis for a useful technique to label the erythrocyte and to measure its survival time. After administering 15N-glycine, the concentration of 15N in heme rises rapidly, remains constant for a time, and then falls. Mathematical analysis of the curve indicates a survival time of 120 days for the human erythrocyte. On a similar basis, methyl carbon labeled glycine has been used to determine the life span of the erythrocytes of a number of domestic animals (Table 8-1). The remaining carbons of protoporphyrin are supplied by the tricarboxylic acid (TCA) cycle intermediate, succinyl-CoA. An outline of the pathway of heme and porphyrin synthesis is given in Figure 8-4, which depicts the compartmentalization of their pathways between the mitochondria and cytosol. The nomenclature of the enzymes of porphyrin synthesis varies among authors, so a guide to the nomenclature of the enzymes is provided in Table 8-2. FIGURE 8-3 The synthetic pathway for protoporphyrin and heme. Note that two enzymes are required to catalyze the formation of UROgenIII. The circled numbers correspond to the enzymes listed in Table 8-2. The initial step in the pathway of δ-aminolevulinic acid (ALA) synthesis occurs in the mitochondria and involves the enzymatic condensation of glycine with succinyl-CoA to form ALA. This reaction requires vitamin B6 as pyridoxal phosphate, and it is the pyridoxal-phosphate-glycine complex that condenses with succinyl-CoA (Gibson et al., 1958, Kikuchi et al., 1958). The requirement for pyridoxine explains the pyridoxine responsive anemia of pyridoxine deficiency because in the absence of pyridoxine, the condensation cannot occur. The condensing reaction is catalyzed by the enzyme ALA synthase (ALA-Syn). ALA-Syn is the rate-controlling enzyme for heme synthesis (Granick, 1966). ALA-Syn is induced by heme and is also suppressed by negative feedback inhibition by heme. Thus, the end product, heme, controls its own synthesis (Granick and Levere, 1964). The ALA is next transferred into the cytosol (Sano and Granick, 1961). FIGURE 8-4 The synthetic pathway for protoporphyrin and heme. Note the partitioning of the heme synthetic pathway between the mitochondria and the cytosol. The circled numbers correspond to the enzymes listed in Table 8-2. Two moles of ALA are next condensed to form the precursor pyrrole, porphobilinogen (PBG) in the cytosol (Cookson and Rimington, 1953). The enzyme ALA-dehydrase (ALA-D), an enzyme that is strongly inhibited by lead, catalyzes this reaction. The activity of this enzyme has been assayed in lead poisoning, and a reduced activity is generally regarded as presumptive evidence of exposure to lead. TABLE 8-2 Nomenclature for Enzymes of Porphyrin and Heme Synthesis and Their Synonyms Next, two enzymes, porphobilinogen deaminase (PBG-D) (formerly called uroporphyrinogen I synthase [UROgenI-Syn]) and uroporphyrinogen III cosynthase (UROgenIII-Cosyn) act together to condense four moles of PBG into the cyclic tetrapyrrole, uroporphyrinogen III (UROgenIII). PBG-D initially catalyzes the formation of a symmetrical linear tetrapyrrole. UROgenIII-Cosyn then flips the D ring and closes the pyrroles into an asymmetrical porphyrin ring of the type III configuration. In the absence of the UROgenIII-Cosyn, the symmetrical linear tetrapyrrole spontaneously closes into a symmetrical porphyrin ring of the type I configuration. Normally, there is a great excess of UROgenIII-Cosyn, so UROgenIII is synthesized. Both enzymes have been isolated from the spleens of anemic mice and in vitro, in the presence of both enzymes, UROgenIII was produced (Levin and Coleman, 1967). The eight carboxyl UROgens I or III are next progressively decarboxylated into the four carboxyl coproporphyrinogens (COPROgen) I or III with the decarboxylations catalyzed by the enzyme uroporphyrinogen decarboxylase (UROgen-D). UROgen-D is nonspecific so it catalyzes the decarboxylation of either UROgenI or UROgenIII. The COPROgens now move back into the mitochondria (Fig. 8-4). FIGURE 8-5 Alternate pathways for porphyrin synthesis. Normally, enzymes 3 and 4 function together in a coordinated manner to form heme. In the absence of enzyme 4, the alternate and terminal pathway to form the I isomers is taken. The circled numbers correspond to the enzymes listed in Table 8-2. Within the mitochondria, coproporphyrinogen III oxidase (COPROgenIII-Ox) catalyzes the decarboxylation of the two propionic acid groups on the A and B pyrrole rings of COPROgenIII to vinyl groups and the resulting product is protoporphyrinogen III (PROTOgenIII). COPROgenIII-Ox is highly specific for COPROgenIII, and this explains the presence of only type III porphyrin isomers in nature. This also means that COPROgenI is a terminal intermediate that is oxidized to coproporphyrin I, the end product of this path (Fig. 8-5). COPROgenIII, when in excess, is also oxidized to coproporphyrin III. Similarly, the UROgens I and III can be oxidized to their end products, the uroporphyrins I and III. Figure 8-5 illustrates that each of the -gen forms can be oxidized into their free forms, which are the forms usually found in the circulatory system. These free porphyrins and protoporphyrins are photoreactive and are the causative agents of the photosensitivity in the porphyrias. PROTOgenIII, the 2-carboxyl porphyrinogen, is next oxidized at the carbon bridges to form the methene bridges connecting the pyrroles and is catalyzed by protoporphyrinogen III oxidase (PROTOgenIII-Ox). The resulting product is protoporphyrin IX (PROTO IX). Within the mitochondria, ferrous iron (Fe+2) is chelated with PROTO IX to form heme and is catalyzed by the enzyme ferrochelatase (FER-Ch). Iron can also be incorporated with relative ease by a nonenzymic method, but the enzymic iron incorporation is more than 10 times that of the nonenzymic route (Labbe and Hubbard, 1961). Conditions that help to maintain iron in its ferrous form including the presence of reducing agents (ascorbic acid, cysteine, glutathione) enhance both enzymic and nonenzymic iron incorporation. Because iron is incorporated into heme as an integral part of the molecule, labeled iron can also be used as a cohort label to determine the life span of the erythrocyte (Table 8-1). Heme next moves into the cytosol where it is linked to the heme pocket of globin in a precise and stable position that permits binding of oxygen to the heme. Hemoglobin consists of four moles of this heme-globin moiety linked together as a globular tetramer. It is this globular tetrameric form of the hemoglobin molecule that permits the cooperative interaction of oxygen binding, which gives the familiar sigmoid oxygen-hemoglobin saturation curve. In summary, the synthesis of porphyrins, heme, and globin can only occur in those respiring cells with full complements of mitochondrial and cytosolic enzymes. The TCA cycle is an aerobic cycle, and therefore a lack of oxygen would preclude synthesis of succinyl-CoA and hence of heme. PROTO IX formation and the chelation of iron to form heme are also oxygen-requiring systems. Therefore, the reticulocyte with its residual complement of enzymes can synthesize hemoglobin, but the mature erythrocyte, which is devoid of mitochondrial enzymes, cannot. The principal method now employed for the detection of porphyrins in biological materials in the clinical laboratory is based on the characteristic red fluorescence observed when acidic solutions of the porphyrins are exposed to ultraviolet light. The color of the fluorescence cannot be used to distinguish between the uroporphyrins and the coproporphyrins, and, therefore, these must be separated before examination for fluorescence. The separation procedures are based on the solubility differences of the porphyrins in various organic solvents. In general, the following solubility properties are used in the separation of the uroporphyrins from the coproporphyrins: 1. The coproporphyrins are soluble in diethyl ether, whereas the uroporphyrins are not, and therefore uroporphyrins remain in the aqueous phase. 2. Both uroporphyrin and coproporphyrin are soluble in strong acid, 1.5 N HCl. Coproporphyrins are therefore extracted from the organic phase with 1.5 N HCl. The uroporphyrins in the aqueous phase are absorbed with aluminum trioxide and subsequently eluted with 1.5 N HCl. The acidic solutions of the porphyrins are then observed visually for fluorescence or examined in a sensitive fluorometer. The most suitable condition for the excitation of fluorescence is the use of ultraviolet light in the near visible range using aqueous solutions of the porphyrins at pH 1–2. Further means of identification include spectrophotometric examination, melting points of their methyl esters, and high-performance liquid chromatography (HPLC). The quantitative methods employing HPLC with fluorometric detection have been described in detail by Hindmarsh et al. (1999). The HPLC method now appears to be the most accurate method for urine and fecal porphyrins (Zuijderhoudt et al., 2000, 2002). The following screening procedures are guides for further laboratory examinations. The urine for porphyrin examination must be alkalinized because porphyrins readily precipitate in acid urine. Addition of 0.5 g of sodium bicarbonate to the collecting bottle for each 100 ml of urine will keep the urine alkaline. The alkalinized urine can be stored at 4°C for 2 to 3 days before analysis. All contact of the urine with metal must be avoided and unfiltered urine is used. The following is a simplified screening procedure: 1. Place 5 ml urine in a 250-ml separatory funnel and add 5 ml acetate buffer (four parts glacial acetic acid: 1 part saturated sodium acetate) and adjust pH to 4.6 to 5.0. 2. Add 15 ml cold water. 3. Extract the mixture with two 50 ml aliquots of diethyl ether (or until the ether phases show no fluorescence under ultraviolet [UV] light) and pool the aliquots. The coproporphyrins will enter the ether phase. 4. Most of the porphyrins in urine are in the form of their nonfluorescent precursors. Storage of the urine for 24 h in a refrigerator will enhance their conversion to the fluorescent pigments. If fresh urine is used, the ether phase is gently shaken with 5 ml of fresh 0.005% iodine solution (dilute 0.5 ml of a stock 1% iodine in ethanol solution to 100 ml with water) to convert the precursors to the porphyrins. 5. Extract the pooled ether phases with 20 ml 5% HCl (1.5 N) and examine for fluorescence under UV light. Fluorescence indicates the presence of coproporphyrins. The screening test as described for urine can also be used with fecal samples after prior extraction with strong acid. First, 5 g of a fecal sample is emulsified with 10 ml 95% ethanol. Then 25 ml of concentrated HCl and 25 ml water are added to the emulsion, and the mixture is kept overnight at room temperature. The mixture is next diluted to 200 ml with water, filtered, and the filtrate examined for porphyrins as described for urine. Almost all porphyrins in blood are present in the erythrocytes, and only trace amounts are in plasma. Therefore, it is important to use whole blood in the test and to understand that the test is a measure of the porphyrin content of the erythrocytes. Mix 2 ml heparinized whole blood and 6 ml ethyl acetate:glacial acetic acid (4:1) in a glass centrifuge tube. Stir thoroughly, centrifuge, and pour off the supernate into a second centrifuge tube. Add 1 ml 3 N HCl, vortex, and allow the phases to separate. Fluorescence under UV light in the aqueous layer indicates the presence of porphyrins in the erythrocytes. Normally, there will only be a trace of fluorescence so that more than a trace indicates an abnormal concentration. The Watson and Schwartz test for porphobilinogen is reliable for screening (Watson and Schwartz, 1941). For this test, 3 ml fresh urine is mixed with 3 ml Ehrlich’s aldehyde reagent (0.7 g p-dimethylaminobenzaldehyde, 150 ml concentrated HCl, and 100 ml water), the reagent that is used for the urine urobilinogen test. Next, 5 ml chloroform are added, shaken vigorously in a separatory flask, and allowed to separate. The porphobilinogen aldehyde formed in the test is insoluble in chloroform and will remain in the lower aqueous phase. If the pink color is due to urobilinogen, it will be extracted into the chloroform phase. Porphobilinogen is found characteristically in the urine of patients with hepatic forms of porphyria, forms that have not been reported in animals. By convention, the term porphyria is used to define those disease states that have a hereditary basis and increased urinary or fecal excretion of uroporphyrins and coproporphyrins. Depending on the fundamental biochemical defect, the porphyrias can be broadly classified on the basis of their tissue of origin, the erythropoietic system, or the liver. The term porphyrinuria is used to define those acquired conditions in which the principal, if not the sole, porphyrins being excreted are the coproporphyrins. Excess coproporphyrin excretion is observed in a wide variety of conditions including infections, hemolytic anemias, liver disease, and lead poisoning. The screening test for coproporphyrinuria has been especially useful for detecting exposure to lead. Many systems for classifying porphyrias have been devised, and most are based on the defect in the tissue of origin, the erythropoietic system, or the liver. There is general agreement on the classification of the erythropoietic forms, but there is still some uncertainty as to the classification of the hepatic forms. These have been classified on the basis of their clinical manifestations, heredity, porphyrins excreted, and their enzymatic defects (Elder and Sheppard, 1982; Hindmarsh, 1986; Sassa, 2006; Sassa and Kappas, 2000). Each of the enzymatic defects has now been described on a molecular level in humans, and they have been summarized by Sassa and Kappas (2000). The genes have been cloned, and it is now known that there is a great deal of genetic heterogeneity, which presumably accounts for the wide variety of disease manifestations in each of the porphyrias. A useful system of classification is given in Table 8-3. Methods are also available for the experimental production of the two major types of porphyria. In lead or phenylhydrazine poisoning, a type of porphyrinuria has been observed that has some of the characteristics of erythropoietic porphyria of humans and cattle (Schwartz et al., 1952). A hepatic form can be produced with sedormid (allylisopropylacetylcarbamide) (Schmid and Schwartz, 1952), dihydrocollidine (Granick and Urata, 1963), or hexachlorobenzene. a. Introduction One of the characteristic findings in bovine congenital erythropoietic porphyria (CEP) is a reddish brown discoloration of the teeth and bones. Discolorations of this type have been observed in cattle at slaughter since the turn of the century, and these cattle are presumed to have had the disease. The first living cases were encountered in South Africa in a herd of grade Shorthorn cattle. Since then, CEP has been reported in cattle in Denmark (Jorgensen and With, 1955), the United Kingdom (Amoroso et al., 1957), the United States (Rhode and Cornelius, 1958), and Jamaica (Nestal, 1958). The disease has been seen primarily in Holsteins with a few cases in Shorthorns and Jamaican cattle. The simple Mendelian autosomal recessive heredity of the disease was established by the study of the genealogy of the affected cattle and by breeding experiments. The affected homozygotes are characterized by discoloration of the teeth and urine, photosensitivity of the light areas of the skin, and generalized lack of condition and weakness. The condition is present at birth, and severely affected calves must be protected from sunlight if a state of health is to be maintained. The predominant symptoms of teeth and urine discoloration and the photosensitization of the severely affected animal are readily apparent, and a tentative diagnosis can be confirmed by the orange-red fluorescence of the teeth and urine when examined with near ultraviolet light or a Woods lamp. The symptomatology of affected animals, however, may vary from minimal to severe and with age and season. The discoloration of the teeth may vary in the same breed, being more pronounced in the young and less apparent in the older animals. Porphyrin deposits are heavily concentrated in the dentine so the occlusal surfaces should also be examined. If porphyrins are present, the discoloration and the fluorescence of the dentine will be readily detected. The degree of photosensitization will vary with the amount of porphyrin deposition in the dermis, coat color, density of the coat, and extent of exposure to sunlight. The photosensitization may be so slight as to escape detection. At times, loss of condition may be the only outward symptom for which the veterinarian is called. Marked variations in the urinary excretion of the porphyrins also occur. These may range from minimal to thousands of micrograms in the same animal so that the urine color may vary widely. The variations observed in this disease indicate the dynamic state of flux of porphyrin metabolism in the living animal, and the porphyrin deposits constitute a part of this dynamic state. b. Distribution of Porphyrins Some normal values for the porphyrins in animals are given in Table 8-4. These values are to be considered as best approximations obtained from a relatively few animals. The figures, however, indicate the very low concentrations of the free porphyrins normally found in the body. Thus, the finding of porphyrins in greater than trace amounts is always significant. Porphyrin concentrations in porphyric cows and calves are given in Table 8-5 (Kaneko and Mills, 1970).
I. INTRODUCTION
II. PORPHYRINS
A. Structure of the Porphyrins
B. Synthesis of Porphyrins and Heme
1. δ-amino levulinic acid
2. Porphobilinogen
Abbreviations
Nomenclature
1. ALA-Syn
delta-aminolevulinate synthase (synthetase)
2. ALA-D
delta-aminolevulinate dehydrase (dehydratase); porphobilinogen synthase
3. PBG-D
porphobilinogen deaminase, uroporphyrinogen I synthase (synthetase), hydroxymethylbilane synthase
4. UROgenIII-Cosyn
uroporphyrinogen III cosynthase (cosynthetase)
5. UROgen-D
uroporphyrinogen decarboxylase
6. COPROgenIII-Ox
coproporphyrinogen III oxidase
7. PROTOgen-Ox
protoporphyrinogen oxidase
8. FER-Ch
ferrochelatase; heme synthase (synthetase)
3. Uroporphyrinogen
4. Coproporphyrinogen
5. Protoporphyrinogen
6. Protoporphyrin
7. Heme
8. Hemoglobin
9. Summary
III. METHODOLOGY
A. Urinary Porphyrins
B. Fecal Porphyrins
C. Blood Porphyrins
D. Porphobilinogen
IV. PORPHYRIAS
A. Classification
B. Erythropoietic Porphyrias
1. Bovine congenital erythropoietic porphyria
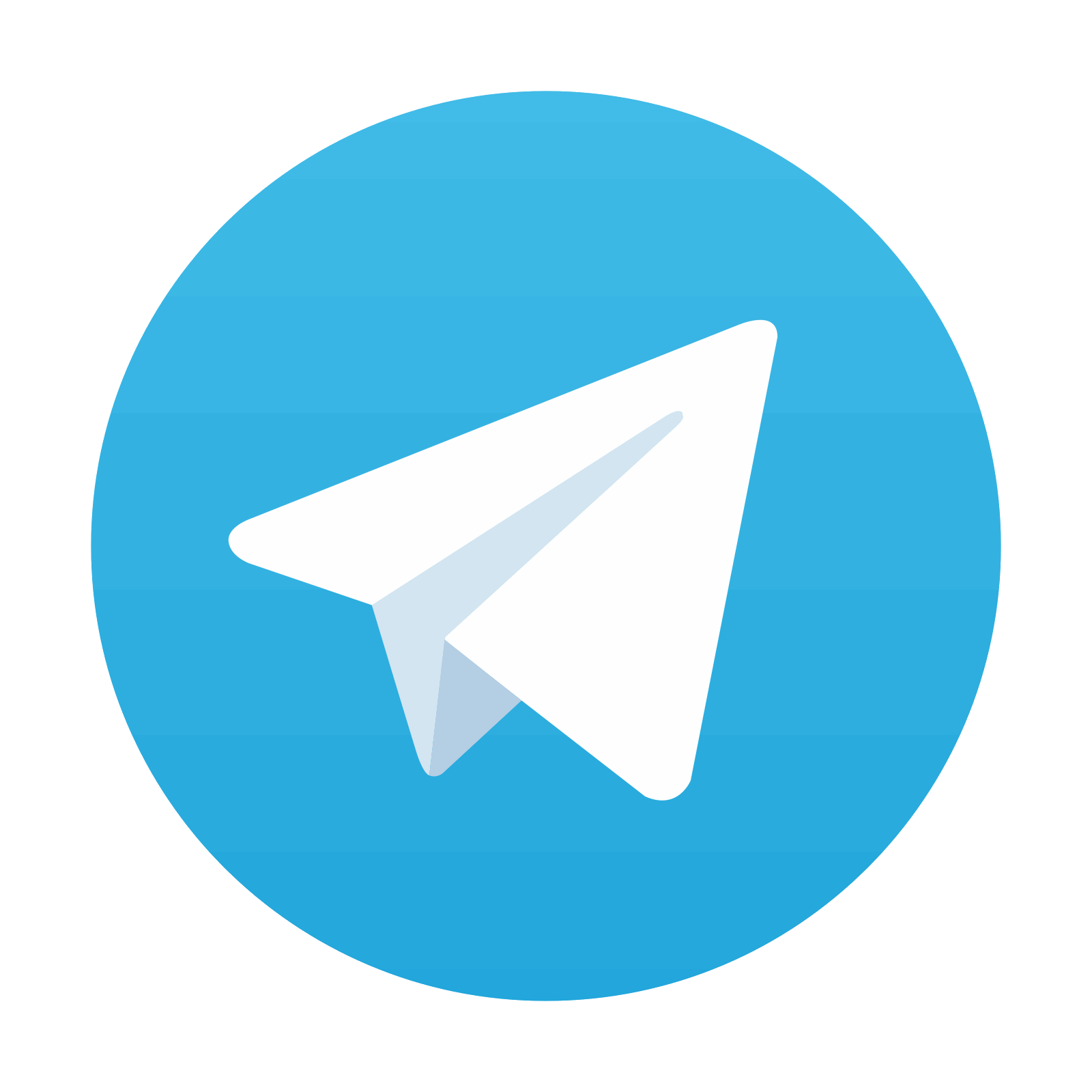
Stay updated, free articles. Join our Telegram channel

Full access? Get Clinical Tree
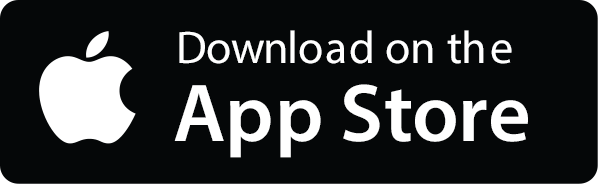
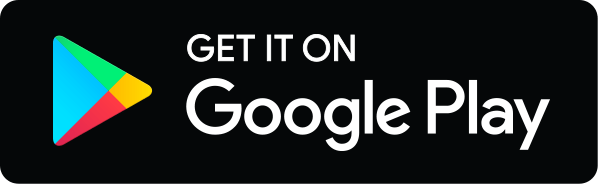