CHAPTER 15 Development of the urogenital system
The urogenital system can be functionally divided into two entirely different components, the urinary system and the genital system, but the embryonic developments of the two are intimately interwoven. Both are derived from the nonsegmented intermediate mesoderm, which is also referred to as the nephrogenic plate, and the neighbouring mesodermal coelomic epithelium. Early proliferation of this portion of the mesoderm causes a longitudinal swelling – termed the urogenital plate – along the dorsolateral aspect of the abdomen.
DEVELOPMENT OF THE URINARY SYSTEM
Mammalian kidney formation, nephrogenesis, commences with the successive appearances of three generations of kidney primordia: the pronephros, mesonephros, and metanephros (Fig. 15-1). These primordia arise in an anterior-posterior wave of cellular differentiation in the so-called nephrogenic cord which is part of the urogenital plate. As these primordia develop, their excretory ducts become localized parallel to the nephrogenic cord. The duct associated with the second kidney primordium, the mesonephros, becomes particularly well-developed and is referred to as the the mesonephric or Wolffian duct.
Mesonephros
The fully developed mesonephros is of considerable size in domestic animals, forming an ovoid organ on each side of the midline (Figs 15-2, 15-3). Due to its size, it is partly responsible for the physiological herniation of the growing intestinal loop (see Chapter 14). The size of the mesonephros correlates to some extent with the type of placenta and how well the placenta cleans the blood. Thus, it is largest in species with a six-layered placental barrier (epitheliochorial placenta; see Chapter 9) such as the pig and sheep, and smallest in carnivores with a four-layered barrier (endotheliochorial placenta). Soon after their formation, most of the mesonephric tubules start to degenerate. Degeneration of the cranial portion of the mesonephros occurs around the eighth and ninth week of gestation in the horse, and tenth week in cattle.
Metanephros
It is the third generation of urinary organs, the metanephros, that matures to form the permanent kidney (Figs 15-4, 15-5). In cattle, its development begins at the level of somites 26 through 28, when the embryo is about 6–7 mm in length. The metanephros is derived from two primordial structures: the ureteric bud, an outgrowth of the mesonephric duct, and the metanephric blastema, which is located in the sacral region and originates from the posterior end of the nephrogenic cord. In forming the metanephros, the ureteric bud grows anteriodorsally into the overlying posterior intermediate mesoderm where it interacts with this loosely organized mesenchyme, the metanephric blastema, located on the lateral aspect of the aorta. This epithelial-mesenchymal interaction leads to a dramatic transformation of the mesenchyme into an epithelial phenotype, which reciprocates by inducing the ureteric bud to undergo arborization and generation of nascent nephrons.
Collecting system
The combination of elongation and branching (up to 14 or 15 dichotomous branching divisions) of the ureteric bud plays a central role in the development of the metanephros (Fig. 15-6). Outgrowth of the ureteric bud from the mesonephric duct is induced by the secretion of alial-derived neurotrophic factor (GDNF) by glial cells derived from the undifferentiated mesenchyme of the metanephrogenic blastema. GDNF secretion is controlled by WT-1, a transcription factor that makes the mesenchyme competent to respond to induction by the ureteric bud. The inductive signal GDNF is bound by c-Ret, a member of the tyrosine kinase receptor superfamily, which is located in the plasma membrane of the epithelial cells of the ureteric bud. In response to GDNF, the epithelial cells of the ureteric bud produce fibroblast growth factor 2 (FGF2), BMP1/BMP2, and leukaemia inhibitory factor (LIF), which stimulate the surrounding metanephric mesenchyme to form precursors of renal tubules.
The mesenchymal condensations of the metanephric blastema around the terminal buds of the branches develop into a renal tubule in stages. The aggregates of metanephric blastema cells organize into an epithelial cord that canalizes to form a tubule (Fig. 15-4). The primordium of the tubule first assumes a comma shape, with a central lumen at its distal end and a basal lamina assembled on its outer surface. These events mark the transformation of mesenchymal cells into an epithelium. Subsequently, a slit-like space develops outside the transforming podocyte precursors in the tubular primordium, and precursors of vascular endothelial cells migrate into this space. These vascular endothelial cells ultimately form the capillaries of the glomerulus, which are connected with branches of the lateral segmental arteries arising from the aorta. Between the cells of the glomerular endothelium and the neighbouring podocytes, a thick basal lamina is formed that later serves as an important component of the renal filtration barrier.
Development of the kidney involves the formation of approximately 15 successive generations of nephrons in the peripheral cortical zone (Figs 15-7, 15-8, 15-9). The first renal corpuscles to form are located at the corticomedullary junction. Many of the early nephrons become apoptotic during later fetal stages. Collecting tubules elongate and new generations of nephrons are induced and form at progressively more superficial levels. Thus, the outermost nephrons are less mature than are those located deeper in the cortex. Depending on the species, nephrogenesis ceases at or shortly after birth; nephrogenesis continues during the first weeks post partum in the dog, and for the first three weeks in the pig. The number of nephrons formed differs between the species: about 200 000 nephrons form in the feline kidney, 300 000–500 000 in the dog, and 1.5–4 million in ruminants and pigs.
The variations in macroscopic appearance of the mature kidney result from differences in the branching of the ureteric bud and the arrangement of nephrons associated with these branches (Fig. 15-9). In cattle, the ureteric bud, from which the ureter is derived, forms two major branches (primary branches) that subdivide into 12 to 25 minor (secondary) branches. Consequently, the bovine kidney develops 12–25 separate lobes, each retaining its distinct pyramid-forming papillae. The bovine kidney is therefore often referred to as a multipyramidal kidney. The papillary ducts within each lobe drain into a calyx. The bovine kidney differs from that of the other domestic animals in having no renal pelvis.
In the pig, although the cortex is not lobated, the medulla is subdivided into renal pyramids forming papillae and so the porcine kidney is also multipyramidal. Each papilla consists of nephric loops and the collecting tubules and ducts that empty into terminal branches of a minor calyx. The dilated end of the porcine ureteric bud forms the renal pelvis. From the two major divisions of the renal pelvis (major calyces) up to ten funnel-shaped minor calyces originate. Despite it superficially smooth appearance, the multilobular structure of the porcine kidney reveals itself in both its multipyramidal appearance and the separate drainage of each lobe through minor calyces.
Bladder and urethra
During development of the hindgut, the cloaca is subdivided by the urorectal septum into the rectum (dorsally) and the urogenital sinus (ventrally). The latter comprises an anterior pelvic region and a posterior phallic region (Fig. 15-10). Cranially, the urogenital sinus is connected to the allantoic cavity through the urachus which is continued in the allantoic stalk. After degeneration of the cloacal membrane the allantoic stalk opens caudally into the amniotic cavity through the urogenital orifice.
The bladder forms from the proximal portion of the urachus and the pelvic region of the urogenital sinus (Figs. 15-10, 15-11). The attenuated distal end of the urachus solidifies into a cord-like structure, which is suspended in a peritoneal fold that ultimately forms the median umbilical ligament, leading from the bladder to the umbilical region. As the bladder grows, its expanding wall incorporates the terminal portions of mesonephric ducts and the ureteric buds, and each duct system develops its own separate openings into the developing bladder. Initially, the mesonephric ducts open anterior to the ureteric buds, but gradually the positions of these orifices shift, so that the ends of the ureteric buds finally open into the bladder laterally and anterior to the mesonephric ducts. A triangular area in the dorsal wall of the neck of the bladder and the cranial urethra represents the region of mesonephric duct and ureteric bud incorporation. The base of this trigone is delineated anteriorly by the entrance of the forming ureters. The apex is located where the mesonephric ducts each enter to form the ductus deferens on either side of a small swelling referred to as the urethral crest. The trigone in the dorsal wall of the bladder is lined by epithelium of mesodermal origin whereas the rest of the bladder epithelium is derived from the endoderm. The non-epithelial components of the bladder wall (connective tissue and smooth muscle) are derived from the visceral mesoderm.
DEVELOPMENT OF THE MALE AND FEMALE GENITAL ORGANS
Sex determination
Chromosomal sex is established at fertilization, when a Y- or an X-chromosome-bearing spermatozoon fuses with the oocyte to determine the genetic sex of the zygote. The sex determining region of the Y gene (Sry), a member of the Sox family of transcription factors, is the testis-determining gene. It is located within a 35-kilodalton region on the short arm of the Y-chromosome. In the mouse, Sry encodes a 223 amino acid DNA-binding protein of the high mobility group (HMG) box class. It presumably regulates the expression of other genes, which then confer the cellular phenotype. Additional evidence that Sry is the Y-encoded testis-determining gene has come from experiments with transgenic mice: sex reversal from female to male was found after a small genomic fragment carrying the mouse Sry gene was introduced as a transgene into XX embryos. These results demonstrate not only that Sry is involved in testis determination, but that it is the only gene of the Y chromosome that is required for this process. Although the genetic sex of the embryo is fixed at fertilization, the gross sex-specific phenotype is not apparent until after 4 to 7 weeks of development, depending on the species. Subtle differences, however, are expressed much earlier as it has been demonstrated that the rate of development up to the blastocyst stage is faster in males than in females.
Primordial germ cells and indifferent stage of gonadal development
During the indifferent stage of gonadal development, primordial germ cells (PGCs) migrate from the yolk sac into the gonadal primordium (see Chapter 4). The PGCs are the source of germ cells in the adult gonad. PGCs can first be identified in the epiblast, where their formation, at least in mice, is dependent upon the expression of BMP4 by the extraembryonal ectoderm (see Chapter 20). The extraembryonic ectoderm is formed during development of the amnion in mice, and, because amniogenesis is basically different in the domestic animals and the mouse, it is uncertain whether a similar mechanism operates in the domestic species. PGCs can be identified histochemically by their high alkaline phosphatase activity and by their expression of pluripotency transcription factors such as Oct4. These cells pass through the early primitive streak and become located as a small cluster of cells in the extraembryonic mesoderm, near the base of the allantois. They then become incorporated into the endoderm of the posterior wall of the yolk sac, where they are apparently further dislocated from the embryonic disc. Subsequently, the PGCs shift to a site in the mesoderm along the yolk sac and allantois stalks. From there they apparently migrate in the wall of the hindgut and through the dorsal mesentery until they reach the newly formed genital ridge (Fig. 15-12).
Studies in mutant mice have demonstrated that the passage of PGCs to the dorsal mesentery and into the genital ridges probably requires active locomotion. This, especially the initial stages of migration, is accomplished by active amoeboid movement of the cells in response to molecular clues form the extracellular matrix. In avian species, PGCs reach the genital ridge via the blood stream. PGCs divide during migration to the gonadal primordia in response to mitogenic factors such as LIF and Steel factor and many remain linked to one another through long cytoplasmic processes. They also express the transcription factor Oct4, which is involved in the maintenance of their pluripotent state (see Chapter 4) and is the same gene that maintains the undifferentiated state of blastomeres and the ICM in developing embryos (see Chapter 6). PGCs can be found in the genital ridge by Day 16 in the pig, Day 21 in dogs, Day 22 in sheep, Day 25 in cattle, and Day 28 in humans. Approximately 1000 to 2000 PGCs enter the genital ridge. A few days after colonizing the genital ridges, PGCs undergo mitotic arrest. In the testis primordium the PGCs do not enter meiosis until puberty whereas meiosis is initiated during fetal development in the ovary primordium.
Although the sex of a mammalian embryo is determined genetically at the time of fertilization, the genital ridges remain morphological indifferent during the first weeks of gestation. When the PGCs arrive in the gonadal ridge, the resident mesenchymal cells and the coelomic epithelium proliferate and, consequently, the developing gonadal ridges project into the coelomic cavity (Figs. 15-12, 15-13). This ridge forms in embryos of approximately 9–10 mm CRL and grows quickly when the PGCs arrive. Cords of epithelial cells from the mesonephric tubules and regressing glomerular capsules penetrate the mesenchyme of the genital ridge and form a number of irregularly shaped cords – the primitive sex cords or gonadal cords – that incorporate PGCs. In both sexes these cords are temporarily connected to the surface epithelium. At this time it is still impossible to differentiate the male and female gonad morphologically and they are therefore referred to as indifferent gonads.
Differentiation of the testis
Differentiation of the testis (Figs 15-14, 15-15) occurs under the influence of the Sry gene (testis-determining factor) on the Y-chromosome. Without the expression of products of this gene, the indifferent gonad develops somewhat later into an ovary. In male embryos, transcripts of the Sry gene only become detectable in the genital ridge just at the onset of testis differentiation. Neither the expression of the Sry gene in the testis primordium, nor subsequent testicular development, is dependent on the presence of germ cells. Sry triggers testis formation by inhibiting Dax-1, a member of the nuclear receptor family, which is also expressed in the indifferent gonad at the same time. The inhibition of Dax-1 is necessary for a genetically male gonad to express its sex phenotypically and develop into a testis.
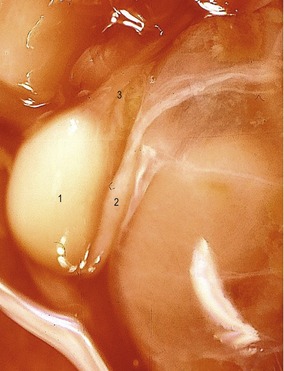
Fig. 15-15: Testis (1) and epididymis (2) of a bovine fetus with a CRL of 12 cm. 3: Plexus pampiniformis.
Under the influence of the Sry gene, the cells of the primitive sex cords continue to proliferate and penetrate deep into the medulla to form the testicular or medullary cords (Figs 15-16, 15-17). The subsequent development of the indifferent gonad into a testis is initiated in the medullary region of the gonadal ridge. The testicular cords transform into solid tubules composed of primitive germ cells centrally and presumptive sustentacular cells or Sertoli cells peripherally. These tubules are arranged in horseshoe-like loops connected at both ends to a network of tiny cells strands, the later rete testis. The testicular tubules develop a lumen at the time of puberty and become the seminiferous tubules. The rete testis eventually joins the efferent ductules, which are derived from remaining mesonephric tubules. They link the rete testis to the mesonephric or Wolffian duct, which becomes the ductus epididymidis and the ductus deferens.
In the mesenchyme between the testicular cords, the first generation of androgen-producing Leydig cells develop in cattle at a CRL of 30 mm (Day 42) and in pigs at 33 mm. During the next two days, these cells initiate an increasing production of testosterone and androstendione. This endocrine activity is important for the differentiation of the male sexual duct system, the development of the external male genitalia, and differentiation of the sexual centres in the brain, which are important for the development of male behaviour. After several weeks to months (7 months of gestation in cattle), the first (fetal) generation of Leydig cells gradually involutes, to be replaced later by a second generation of Leydig cells before puberty. The second generation cells differentiate from connective tissue cells and are responsible for initiation and further stimulation of spermatogenesis.
Descent of the testes
This refers to the migration of the testes from their intra-abdominal site of development into the scrotum, usually located in the inguinal region (Fig. 15-18). In mammals, this process is subject to species variation. For instance, in aquatic mammals, elephants, and armadillos, the testes remain within the abdominal cavity and descent does not occur. In most mammals, however, including all our domestic species, the testes migrate to an extra-abdominal location. In these species, a temperature of 2–4°C below the core body temperature is required for normal spermatogenesis.
< div class='tao-gold-member'>
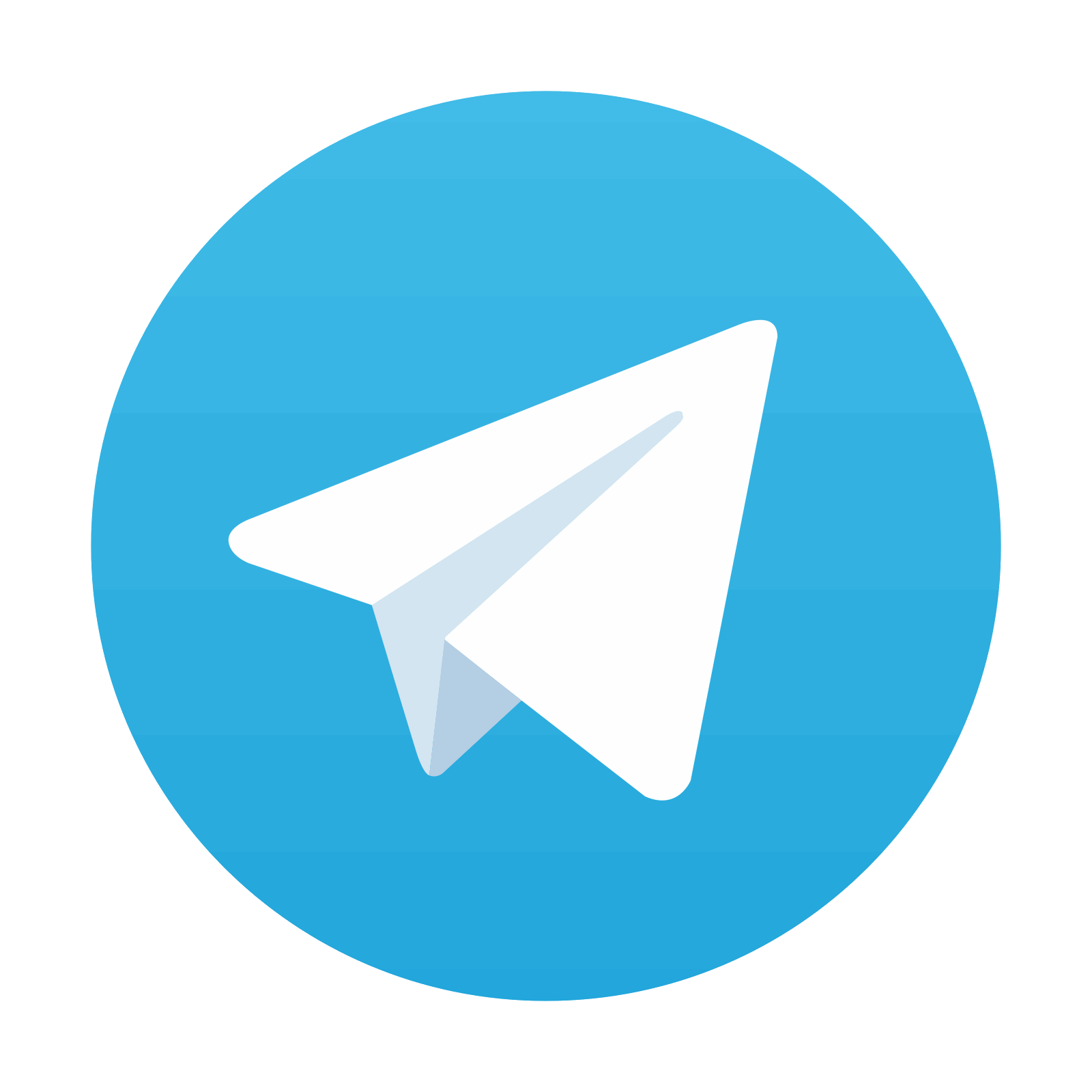
Stay updated, free articles. Join our Telegram channel

Full access? Get Clinical Tree
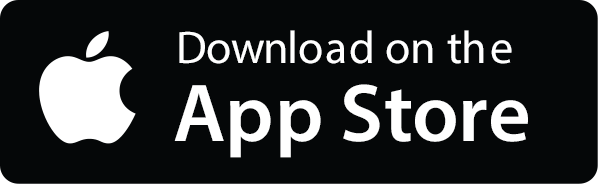
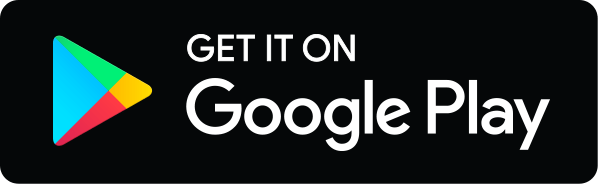