CHAPTER 10 Development of the central and peripheral nervous system
The central nervous system (CNS) develops from the ectoderm once the latter has become specified into surface ectoderm and neuroectoderm. Some of the early features of nervous system development have already been addressed in relation to neurulation (see Chapter 8) and will be briefly recapitulated here in discussing the development of the CNS more specifically. The peripheral nervous system (PNS) develops in association with the CNS as the communication system between the CNS and the rest of the body.
NEURAL PLATE
The development of the neural plate, a thickening of the ectoderm that represents the primordium of the nervous system, is induced by the notochord (see Chapter 8) a major axial signalling centre of the trunk of the early embryo. Subsequently, the neural plate folds and forms the neural tube. Some of the molecular mechanisms underlying these processes have been clarified recently in mice. The notochord, which is in close proximity to the midline neural plate during this stage, releases sonic hedgehog (Shh). Much of the surface ectoderm of an embryo during gastrulation produces Bone Morphogenetic Protein-4 (BMP4). This signalling protein prevents the dorsal ectoderm from forming neural tissue. Under the influence of Hepatic Nuclear Factor-3beta (HNF-3beta), cells of the developing notochord secrete noggin and chordin. These two molecules are potent neural inducers that block the inhibitory influence of BMP4 and thus allow the ectoderm dorsal to the notochord to form neural tissue.
NEURAL TUBE
The neural tube is a prominent structure that dominates the anterior (future cephalic) end of the embryo. In the following we will see how the early neural tube develops into the major morphological and functional components of the mature nervous system (Fig. 10-1). Before neurogenesis, the neural plate and the neural tube are composed of a single layer of neuroepithelial cells (neuroepithelium). Neuroepithelial cells are highly polarized along their apical-basal axis. This is reflected, for example, in the organization of their plasma membranes: certain transmembrane proteins such as prominin-1 (CD133) are found selectively in the apical plasma membrane.
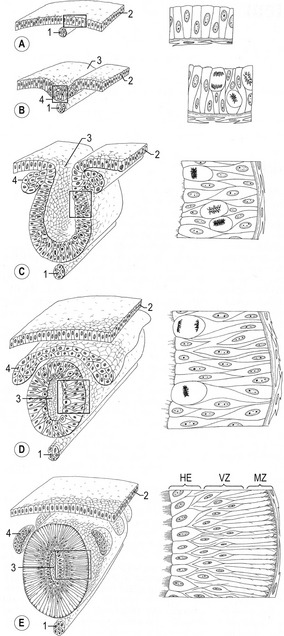
Fig. 10-1: Formation of the neural tube and the neural crests. The boxed areas in A–C are enlarged to the right (modified after Rüsse and Sinowatz, 1998). A: 1: Notochord; 2: Surface ectoderm: B: 1: Notochord; 2: Surface ectoderm; 3: Neural groove; 4: Neural plate. C: 1: Notochord; 2: Epithelium of the neural groove; numerous mitoses occur in the neural epithelium; 3: Neural groove; 4: Neural crest; D: 1: Notochord; 2: Surface ectoderm; 3: Neural tube; 4: Neural crest, which at this stage is still a continuous sheet of cells. E: 1: Notochord; 2: Surface ectoderm; 3: Neural tube; 4 Neural crests, which are segmented into groups of cells giving rise to different cell types. E: Ependymal cells; VZ; Ventricular zone; MZ: Marginal zone.
Courtesy Sinowatz and Rüsse (2007).
Shortly after induction, the epithelium of the neural plate and early neural tube organizes into a pseudostratified epithelium in which the nuclei appear to be located in several separate layers because they are at different heights within the elongated neuroepithelial cells. The nuclei shift extensively within the cytoplasm as the cell cycle progresses (Fig. 10-2). DNA synthesis (the S-phase) occurs in nuclei located near the external limiting membrane (the basal lamina surrounding the neural tube). As these nuclei prepare to go into mitosis, they migrate within the cytoplasm towards the lumen of the neural tube where mitosis is completed. The orientation of the mitotic spindle during this division is important for the fate of the daughter cells. If the cleavage plane is perpendicular to the apical (inner) surface of the neural tube, the two daughter cells slowly migrate towards the periphery of the neural tube, where they prepare for another round of DNA synthesis. If, on the other hand, the cleavage plane runs parallel to the inner surface of the neural tube, the two daughter cells have completely different fates. The daughter cell that is closer to the inner surface migrates away very slowly and remains a proliferative progenitor cell that is still capable of mitosis. The daughter cell that is closer to the basal surface (external limiting membrane) inherits a high concentration of the Notch receptor on its surface and can now be called a neuroblast. The neuroblasts are the precursor cells of neurons and begin to produce cell processes that ultimately become axons and dendrites.
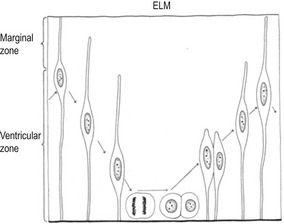
Fig. 10-2: Interkinetic nuclear migration within the neural tube. Within the pseudostratified epithelium of the neural tube, nuclei that synthesize DNA (S-phase) are located near the external limiting membrane (ELM) but then move toward the inner margin of the neural tube, where mitosis occurs.
Interkinetic nuclear migration in the neuroepithelium is accompanied by a change in nuclear morphology: the nucleus adopts an elongated shape along the apical-basal axis when migration begins and rounds up when migration stops. This is consistent with the idea that the nucleus is pulled by some cytoskeletal machinery. Early work on interkinetic nuclear migration indicated the involvement of microtubules, an idea that is supported by the observation that nuclear positioning is a microtubule-dependent process in many cell types. Recent studies of the lissencephaly 1 (LIS1) gene also support this idea. Mutations in the human LIS1 gene are responsible for the type I form of lissencephaly (smooth brain), a severe genetically caused malformation of the brain. The LIS1 protein forms a complex with cytoplasmic dynein and dynactin which binds to microtubules and disturbs microtubule dynamics. Mice with reduced LIS1 expression show defects in the interkinetic nuclear migration in neuroepithelial cells and abnormal neuronal migration.
In addition to microtubules, actin and myosin filaments are also probably involved in the interkinetic nuclear migration in neuroepithelial cells. Cytochalasin B, a drug that inhibits actin polymerization, blocks the process, and ablation of non-muscle myosin heavy chain II-B results in disordered nuclear migration in the cells.
CELL LINEAGES OF THE CENTRAL NERVOUS SYSTEM
During development, neural stem cells give rise to all the neurons of the mammalian CNS (Figs 10-3, 10-4, 10-5) and also the two types of macroglial cell, the astrocytes and oligodendrocytes. Usually, two criteria are applied to define a cell as a stem cell: self-renewal and pluripotency (or at least multipotency). Self-renewal indicates that the cell is capable of an unlimited number of divisions each resulting in either two stem cells or a stem cell and a committed cell. Pluripotency or multipotency implies that the cell can give rise to numerous types of differentiated cells – all cell types of the mammalian body in the case of pluripotency (see Chapter 2). However, this concept has to be somewhat modified when applied to the CNS: ‘stem cells’ in this context are neural cells that are self-renewing, but not necessarily for an unlimited number of cell divisions, and they can be multipotent or unipotent.
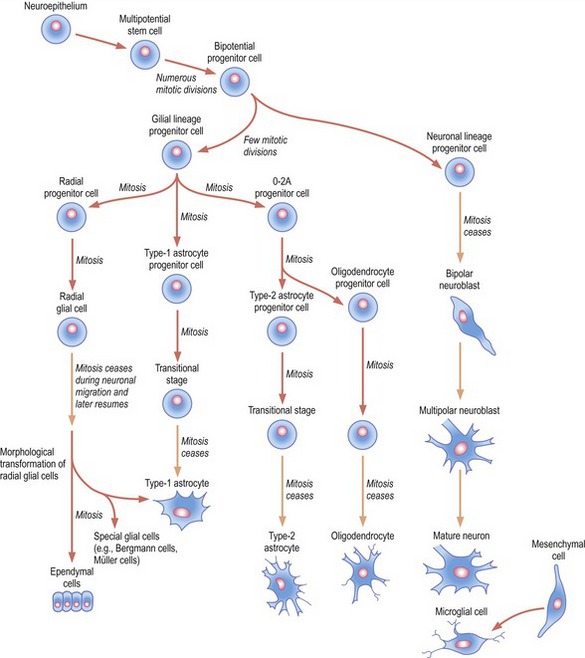
Fig. 10-4: Origins of neurons and various types of glial cells. Neurons, oligodendrocytes, fibrillar and protoplasmic astrocytes, and ependymal cells orginate from neuroepithelial cells. Microglia (Hortega glia) develop from mesenchyme cells.
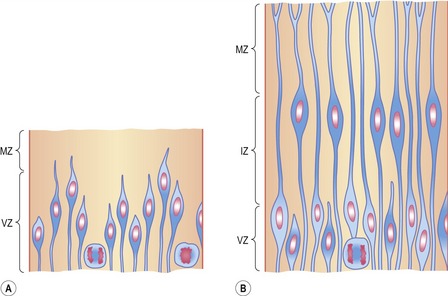
Fig. 10-5: A: During its early development, the epithelium of the neural tube consists of the ventricular zone (VZ), where neural epithelial cells undergo mitoses, and the marginal zone (MZ), which contains elongated processes of the cells. B: Section through the neural tube at a slightly more advanced stage than A. Neuroepithelial cells, which have left the mitotic cycle, migrate away from the lumen of the neural tube and form an intermediate cell layer (IZ: intermediate zone).
The neuroepithelial cells that can be considered the stem cells of the CNS first undergo symmetrical, proliferative divisions, each generating two daughter stem cells. These divisions are followed by numerous asymmetrical, self-renewing divisions, each of which results in a daughter stem cell and a more differentiated cell such as a progenitor cell. Neural progenitor cells typically undergo symmetrical, differentiating divisions that generate two postmitotic cells ready for terminal differentiation.
The origins of most cells found in the mature CNS can be traced to multipotent stem cells within the early neuroepithelium. These cells undergo many mitotic divisions before maturing into bipotent progenitor cells, which give rise to either neuronal or glial progenitor cells. This developmental bifurcation is accompanied by a significant change in gene expression. For example, multipotent stem cells express an intermediate filament protein called nestin. Nestin expression is down-regulated as descendants of bipotent progenitor cells separate into neuronal progenitor cells, which express neurofilament protein, and glial progenitor cells, which express glial fibrillary acidic protein.
The neuronal progenitor cells give rise to a series of neuroblasts. The earliest bipolar neuroblasts possess two slender cytoplasmic processes that contact both the external limiting membrane and the central luminal border of the neural tube. By retracting the inner process, a bipolar neuroblast loses contact with the inner luminal border and becomes a unipolar neuroblast. The unipolar neuroblasts accumulate a large amount of rough endoplasmic reticulum (Nissl substance) in their cytoplasm and then begin to send out several cytoplasmic processes. At this point, they are known as multipolar neuroblasts with the principal developmental activities of sending out axonal and dendritic processes and making connections with other neurons or end organs.
The other major lineage stemming from the bipotential progenitor cells is that of the glial progenitor cells. Glial progenitor cells continue to undergo mitosis, and their progeny split into several lines. One, the O-2A progenitor cell, is a precursor to two important lines of glial cells that ultimately form the oligodendrocytes and type-2 astrocytes, the latter distinguished by its antigenic phenotype from type-1 astrocytes derived from another glial lineage. Anatomically, the astrocytes can be divided into protoplasmic astrocytes, found in the grey matter, and fibrous astrocytes found in the white matter. The origin of oligodendrocytes has long been a matter for debate, but studies have shown that they probably arise from progenitor cells located in the ventral ventricular zone alongside the floor plate. From there, they spread throughout the brain and spinal cord and ultimately form the myelin coverings around axons in the white matter of the CNS. The formation of oligodendrocyte precursors depends on sonic hedgehog (Shh), produced by cells in the notochord, as an inductive signal.
A third glial lineage has a more complex history. Radial progenitor cells give rise to radial glial cells, which act as guide wires in the brain for the migration of young neurons. When the neurons are migrating along the radial glial cells of the fetus during midpregnancy, they inhibit the proliferation of the radial glial cells. After neuronal cell migration, the radial glial cells, now free from the inhibitory influence of the neurons, re-enter the mitotic cycle and produce progeny that can transform into a number of cell types: some can seemingly cross lineage lines and differentiate into type-1 astrocytes; others differentiate into various specialized glial cell types; yet others even into ependymal cells and neurons.
Another type of glial cell of the CNS does not originate from the neuroepithelium. These microglial cells, which act as motile macrophages after damage to the CNS, are mesoderm-derived cells that enter the CNS along with vascular tissue and are therefore not found in the developing CNS until it is penetrated by blood vessels.
Whether neural stem cells and their derivative progenitor cells proliferate (by symmetrical divisions) or differentiate (through asymmetrical division) is closely linked to their epithelial characteristics, especially their apical–basal polarity and cell-cycle length. Generally, the period of neuron production precedes that of gliogenesis. The time at which the precursor of a neuron undergoes its last division is called its birthday. Neurogenic cells in the ventral part of the spinal cord and the hindbrain are usually the first to stop dividing, with dorsal and intermediate neurons following. Cortical neurons in the cerebrum and cerebellum are the last population to be formed; they continue to proliferate until the third to fourth month after birth in the dog and the third year of life in humans. In precocial species, including cattle and horses, most cortical neurons are already formed by the time of birth.
HISTOLOGICAL DIFFERENTIATION OF THE CENTRAL NERVOUS SYSTEM
Nerve cells
Neuroblasts arise by division of neuroepithelial cells and, once formed, they lose their ability to divide (Fig. 10-4). Initially, neuroblasts develop two processes and extend from the the lumen of the neural tube to the external limiting membrane. When they start to migrate into the intermediate layer, the central process is retracted and the neuroblasts appear temporarily unipolar. During further differentiation, several small cytoplasmic processes extend from their cell body. One of these processes elongates rapidly, forming the primitive axon, while arborization of the others gives rise to the primitive dendrites. These cells can be now called multipolar neuroblasts that eventually become mature multipolar neurons. Axons of neurons in the basal plate, which leave the marginal zone on the latero-ventral aspect of the cord, form the efferent ventral root of the spinal cord. Axons of neurons in the alar plate penetrate into the marginal zone of the cord where they ascend to higher or lower levels to form association neurons.
Glial cells
The other major cell lineage originating from bipotent progenitor cells is that of the glial progenitor cells (glioblasts), which are formed by neuroepithelial cells after the production of neuroblasts ceases, and their progeny split into several lines. One of them, the 0–2A progenitor cell, is the precursor of two glial cell types that ultimately differentiate into type-2 astrocytes and oligodendrocytes. It has been recently shown that oligodendrocytes are derived from progenitor cells located in the ventral ventricular zone. From there they migrate throughout the spinal cord and brain and form the myelin sheath around neuronal processes. The formation of oligodendrocytes depends on the signalling molecule sonic hedgehog (Shh) produced by cells of the notochord. In contrast to the Schwann cells of the peripheral nervous system, each of which can only wrap itself around one axon, the flat processes of a single oligodendroglial cell in the central nervous system can myelinate several nerve fibres. Myelin sheaths begin to form in the spinal cord during the late fetal period. In general, fibre tracts become myelinated at about the time they become functional (Table 10-1).
Table 10-1: Beginning of formation of the myelin sheath in the central and peripheral nervous systems.
Species | Tissue | Stage of gestation |
---|---|---|
Cat | ||
Pig | Spinal cord | |
Sheep | ||
Cattle |
p.n. = post natum; p.c. = post coitum
Not all glial cells of the spinal cord originate from the neuroepithelium. Microglial cells, which appear in the second half of fetal development, are highly phagocytotic cells derived from the mesoderm.
When neuroepithelial cells cease to produce neuroblasts, they differentiate into the ependymal epithelial cells lining the central canal of the spinal cord. With the generation of neurons, the neuroepithelium is transformed into an epithelium with several cell layers. With the switch to neurogenesis, neuroepithelial cells down-regulate certain epithelial features (notably their expression of tight-junction proteins) at the same time that astroglial hallmarks appear. In essence, after the onset of neurogenesis, neuroepithelial cells give rise to a distinct, but related, cell type: the radial glial cells which exhibit residual neuroepithelial as well as astroglial properties.
Radial glial cells represent more fate-restricted progenitors than neuroepithelial cells and gradually replace them. As a consequence, many of the neurons in the central nervous system are derived from radial glial cells. The neuroepithelial properties that are maintained by radial glial cells include the expression of neuroepithelial markers (such as the intermediate-filament protein nestin) and the maintenance of an apical surface and important features of apical–basal polarity (such as an apical localization of centrosomes and prominin-1). Like neuroepithelial cells, radial glial cells show interkinetic nuclear migration, with their nuclei undergoing mitosis at the apical surface of the ventricular zone and migrating basally for completion of the S-phase of the cell cycle. However, in contrast to neuroepithelial cells, radial glial cells show several properties of astroglia, such as the expression of astrocyte-specific glutamate transporter (GLAST), Ca2+-binding protein S100β, and glial fibrillary acidic protein.
In contrast to early neuroepithelial cells, most radial glial cells have a limited developmental potential; usually they generate only a single cell type, either astrocytes, or oligodendrocytes, or (most commonly) neurons.
DEVELOPMENT OF THE SPINAL CORD
With the beginning of cellular differentiation in the neural tube, the neuroepithelium thickens and appears layered (Fig. 10-6). The layer closest to the lumen of the neural tube is called the ventricular or neuroepithelial layer; it remains epithelial and still shows mitotic activity. However, with further development, the proliferating cell population in the neuroepithelial layer becomes largely exhausted and the remaining cells differentiate to become the ependyma of the central canal and the ventricular system of the brain.
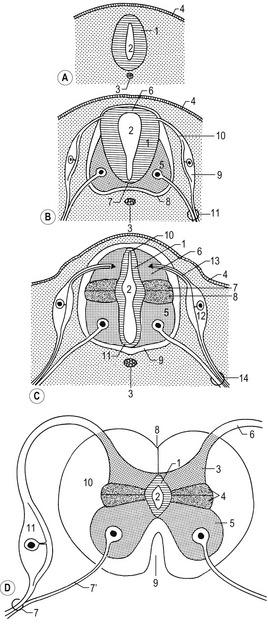
Fig. 10-6: Four successive stages in the development of the spinal cord. A and B: 1: Neuroepithelium; 2: Central canal; 3: Notochord; 4: Surface ectoderm; 5: Basal plate; 6: Roof plate; 7: Floor plate; 8: Marginal zone; 9: Spinal ganglion; 10: Dorsal (sensory) horn; 11: Spinal nerve. C: 1: Neuroepithelium; 2: Central canal; 3: Notochord; 4: Surface ectoderm; 5: Basal plate; 6: Alar plate; 7 and 8: Intermediate horn; 9: Marginal zone; 10: Roof plate; 11: Floor plate; 12: Spinal ganglion; 13: Dorsal root; 14: Spinal nerve; D: 1: Ependyma; 2: Central canal; 3: Dorsal (sensory) horn; 4: Intermediate horn; 5: Ventral (motor) horn; 6: Dorsal (sensory) root; 7: Spinal nerve. 7′: Ventral (motor) root; 8: Septum dorsale; 9: Median fissure; 10: White matter; 11: Spinal ganglion.
The ventricular zone is surrounded by the intermediate or mantle layer (Figs 10-1, 10-5) which contains the cell bodies of postmitotic neuroblasts and presumptive glial cells. As the spinal cord matures, the intermediate layer becomes the grey matter, where the cell bodies of the neurons are located. (Fig. 10-7)
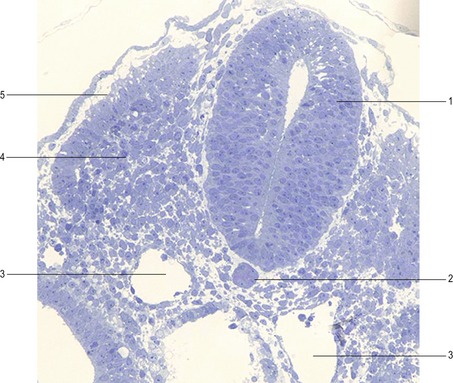
Fig. 10-7: Porcine embryo, Day 16, transverse section. 1: Primordium of the spinal cord; 2: Notochord; 3: Dorsal aorta; 4: Myotome; 5: Dermatome.
As the neuroblasts continue to develop axons and dendrites, a peripheral marginal layer (marginal zone) is formed. It contains neural processes but not neural cell bodies and later forms the white matter of the spinal cord.
Continous addition of neuroblasts to the intermediate layer thickens the neural tube ventrally and dorsally on each side (Figs 10-6, 10-8). The ventral thickenings are referred to as the basal plates. They contain motor neurons (general somatic efferent nerve fibers) and autonomic neurons (visceral efferent nerve fibres; Table 10-2). The dorsal thickenings, the alar plates, form the sensory area with neurons receiving their input from the skin, joints and muscles (general somatic afferent nerve fibres), from the pharynx (special visceral afferent nerve fibres) and from the viscera and heart (general visceral afferent nerve fibres). A small longitudinal groove, the sulcus limitans, marks the boundary between these two areas (Fig. 10-6). The left and right alar plates are connected dorsally over the central canal by the thin roof plate, the two basal plates are connected by the floor plate ventral to the central canal. The roof and floor plates do not contain neuroblasts, their nerve fibres serving primarily to connect one side to the other.
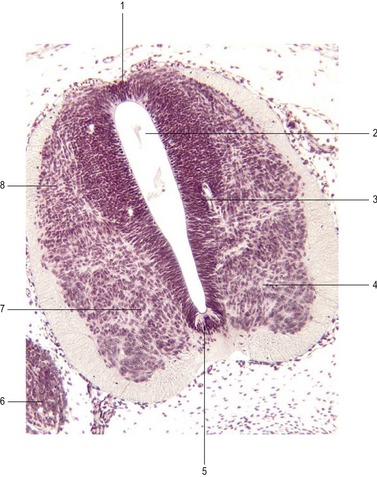
Fig. 10-8: Cat embryo, 17 mm CRL. 1: Roof plate; 2: Central canal; 3 Ependymal zone; 4: Marginal zone; 5: Floor plate; 6 Spinal ganglion; 7: Basal plate; 8: Alar plate.
The mature spinal cord is organized similarly to the embryonic pattern except that the basal and alar plates become subdivided into somatic and visceral components. Transformation of the embryonic into the mature spinal cord (Fig. 10-9) results from proliferation, asymmetrical cell movement of immature neurons in the intermediate layer, and the development of neuronal processes. In the process, the intermediate layer becomes shaped like a butterfly, with prominent dorsal and ventral grey horns arranged around the central canal. In addition to the ventral efferent and the dorsal afferent horns, a small lateral projection of grey matter can be observed between the dorsal and ventral columns at spinal levels thoracic 1 (T1) to lumbar 2 (L2). This is the lateral horn; it contains cell bodies of sympathetic autonomic (visceral efferent) neurons.
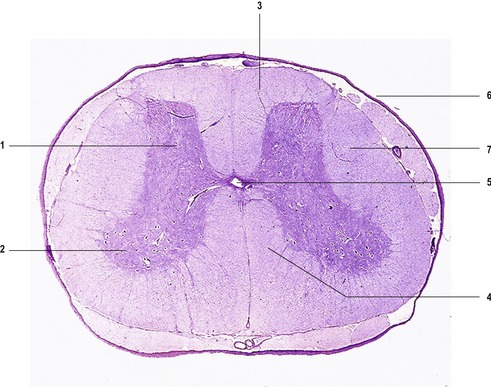
Fig. 10-9: Adult dog, cross-section through the spinal cord. 1: Dorsal (sensory) horn; 2: Ventral (motor) horn; 3: Funiculus dorsalis; 4: Funiculus ventralis; 5: Central canal; 6: Dura mater; 7: Funiculus lateralis.
The marginal layer develops into the white matter of the spinal cord, named because of its whitish appearance that results from the dominance of myelinated axons. This outer layer contains tracts of ascending and descending axons that are grouped together in bundles (funiculi). The dorsal, lateral and ventral funiculi are separated by the efferent spinal nerve roots emerging from the cord and afferent roots entering the cord (Fig. 10-9).
Dorsal root ganglia and spinal nerves
As more fully described in Chapter 8, neural crest cells migrate from the edge of the neural folds and give rise to sensory or spinal ganglia (dorsal root ganglia) of the spinal nerves, as well as several other cell types, including other types of ganglia cells (sensory ganglia, general visceral efferent ganglia of the sympathetic and parasympathetic system), Schwann cells, melanocytes, odontoblasts, and mesenchyme of the pharyngeal arches. Neuroblasts of the spinal ganglia develop two processes, which soon unite in a T-shaped fashion (pseudo-unipolar neurons). Both processes of spinal ganglion cells have the structural characteristics of axons, but the peripheral process can be functionally classified as a dendrite in that conduction within it is toward the cell body. The centrally growing processes enter the dorsal portion of the neural tube and constitute the afferent dorsal root of the spinal cord. In the spinal cord, they either form synapses with afferent dorsal horn interneurons or ascend through the marginal layers to one of the higher brain centres. The peripherally growing processes join the fibres of the ventral root to form the trunk of the spinal nerve through which they eventually terminate in sensory receptors. The common trunk of the spinal nerve almost immediately splits into a dorsal and a ventral ramus. The dorsal rami of the spinal nerves innervate dorsal axial musculature, vertebral joints and the skin of the back. Ventral primary rami innervate the limbs and the ventral body wall and form the two major nerve plexuses, the brachial and lumbosacral plexuses.
Positional changes of the spinal cord: ascensus medullae spinalis
Intitially, the spinal cord runs the entire length of the embryo with spinal nerves passing through the intervertebral foramina at the levels of their origin. Later, however, the vertebral column and the dura grow more rapidly than the spinal cord, leaving the posterior end of the cord terminating at a gradually higher level in the vertebral column (Fig. 10-10). This phenomenon is called ascensus medullae spinalis. The disproportionate growth also forces the spinal nerves to run obliquely from the spinal cord to their corresponding vertebral foramina.
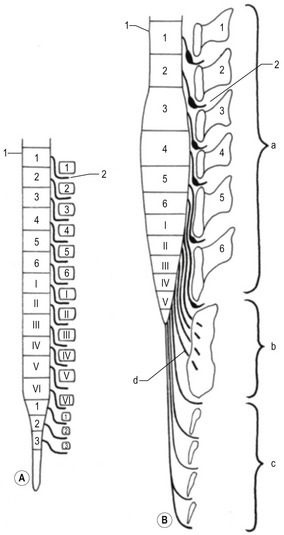
Fig. 10-10: Terminal end of the bovine spinal cord in relation to the end of the vertebral column at two stages (A: 3 months gestation, B: adult) of development. 1: Segments of the spinal cord, from cranial to caudal: 1–6: Pars lumbalis; I–VI: Pars sacralis; 1–3: Pars coccygea; 2: Spinal nerve; a: lumbar vertebrae; b: Os sacrum; c: Coccygeal vertebrae; d: Cauda equina.
DEVELOPMENT OF THE BRAIN
The anterior two-thirds of the neural tube develop into the brain. Fusion of the neural folds in the anterior region, and closure of the anterior neuropore, result in the formation of the three primary brain vesicles (Figs 10-11, 10-12) from which the brain develops. An expansion at the most rostral end of the neural tube forms the first brain vesicle, the prosencephalon or forebrain. The optic vesicles grow out as evaginations from each side of the prosencephalon. The two enlarged regions of the brain posterior to these become the mesencephalon and rhombencephalon (second and third primary brain vesicles). The prosencephalon partly divides into two vesicles, the telencephalon and diencephalon (Figs 10-13, 10-14). The lateral walls of the telencephalon soon become domed, presaging the future cerebral hemispheres. The diencephalon remains undivided, located in the midline and connected to the laterally expanding optic vesicles. The rhombencephalon also divides into rostral and posterior portions: the metencephalon and myelencephalon, respectively (Figs 10-14, 10-15). The metencephalon will give rise to the pons and the corpus trapezoideum ventrally and the cerebellum dorsally. The myelencephalon forms the medulla oblongata, which is the most posterior part of the brain stem and connects it with the spinal cord (Figs. 10-14, 10-16).
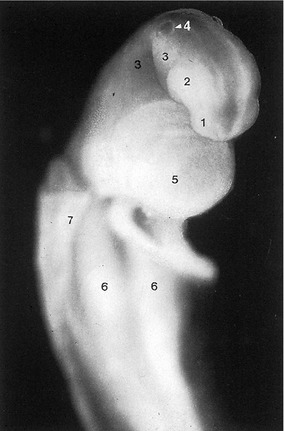
Fig. 10-11: Sheep embryo with three brain vesicles. 1: Prosencephalon; 2: Mesencephalon; 3: Rhombencephalon; 4: Otic placode; 5: Heart; 6: Mesonephros; 7: Body wall.
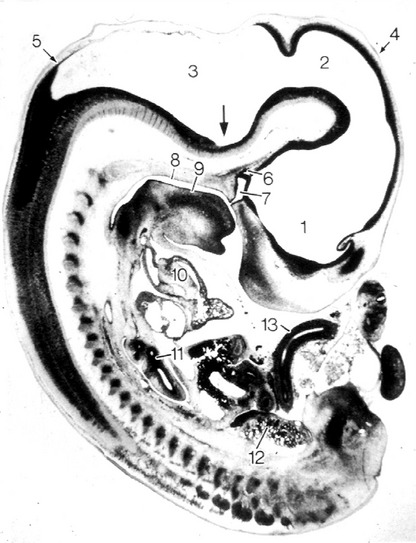
Fig. 10-12: Cat embryo, 10 mm CRL, longitudinal section, 3-vesicle stage of the brain. 1: Prosencephalon; 2: Mesencephalon; 3: Rhombencephalon with pontine flexure (arrow); 4: Cephalic flexure; 5: Cervical flexure; 6: Primordium of neurohypophysis; 7: Rathke’s pouch, primordium of adenhypophysis; 8: Primary oral cavity; 9: Tongue; 10: Heart; 11: Lung; 12: Mesonephros; 13: Intestine.
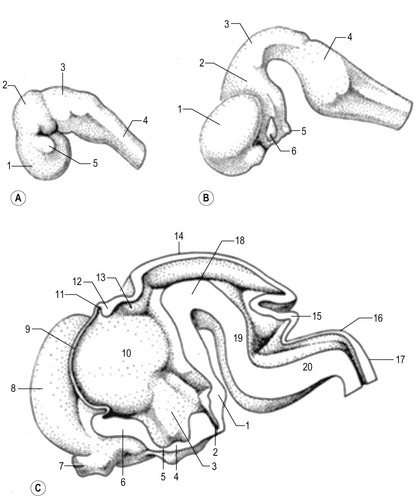
Fig. 10-13: Development of the feline brain at Day 18 (A), Day 22 (B) and Day 25 (C).
A: 1: Prosencephalon; 2: Mesencephalon: 3: Rhombencephalon; 4: Spinal cord; 5: Optic vesicle.
B: 1: Telencephalon; 2: Diencephalon; 3: Mesencephalon; 4: Rhombencephalon; 5: Infundibulum; 6: Stalk of the optic vesicle. C: 1: Primordium of corpus mammilare; 2: Infundibulum; 3: Hypothalamus; 4: Chiasma opticum; 5: Lamina terminalis; 6: Commissural plate; 7: Bulbus olfactorius; 8: Cerebral hemisphere; 9: Roof of the IIIrd ventricle; 10: Thalamus; 11: Primordium of epiphysis; 12: Commissura caudalis; 13: Metathalamus; 14: Corpora quadrigemina; 15: Cerebellum; 16: Lamina tectoria; 17: Cervical flexure; 18: Crus cerebri; 19: Pons; 20: Medulla oblongata.
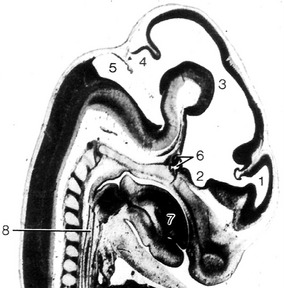
Fig. 10-14: Feline embryo, 17 mm CRL, 5-vesicle stage of brain development, longitudinal section, from Rüsse and Sinowatz, 1998. 1: Telencephalon; 2: Diencephalon; 3: Mesencephalon; 4: Metencephalon; 5: Myelencephalon; 6: Hypophysis; 7: Tongue; 8: Oesophagus.
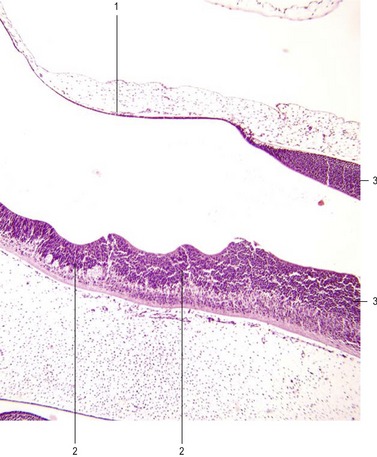
Fig. 10-15: Pig embryo, Day 21.5, longitudinal section through the metencephalon and myelencephalon. 1: Roof plate of the IVth ventricle; 2: Neuromeres of the rhombencephalon; 3: Myelencephalon.
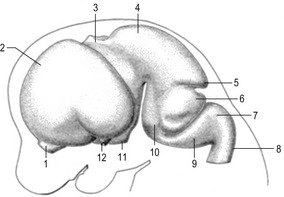
Fig. 10-16: Development of the feline brain at day 33. 1: Bulbus olfactorius: 2: Cerebral hemisphere; 3: Epithalamus; 4: Primordium of corpora quadrigemina; 5: Colliculus caudalis; 6: Cerebellum; 7: Lamina tectoria; 8: Cervical flexure; 9: Medulla oblongata; 10: Pons; 11: Crus cerebri; 12: N. opticus.
The brain flexures
Differential growth of the five secondary brain vesicles (telencephalon, diencephalon, mesencephalon, metencephalon and myelencephalon) gives rise to flexures (Fig. 10-12). As head folding occurs, the mesencephalon bends ventrally to produce the midbrain flexure (cephalic flexure). A second more gradual ventral bend between the hindbrain and the spinal cord is termed the cervical flexure. In the rhombencephalon a slight dorsal bending, the pontine flexure, occurs. The pontine flexure is located in the future pontine region and causes a thinning of the roof of the hindbrain.
Rhombencephalon (hindbrain)
The rhombencephalon consists of the myelencephalon, the most posterior brain vesicle, and the metencephalon, which extends from the pontine flexure to the rhombencephalic isthmus. Posteriorly, the cervical flexure demarcates the myelencephalon from the developing spinal cord. Later, this junction is defined as the level of the roots of the first cervical spinal nerve, roughly at the foramen magnum.
Myelencephalon
The myelencephalon resembles the spinal cord both developmentally and structurally and develops into the medulla oblongata – the posterior part of the brain stem. The medulla oblongata serves as a conduit for tracts between the spinal cord and the higher regions of the brain and also contains important centres for the regulation of respiration and heartbeat.
The fundamental arrangement of alar and basal plates, which are separated by the sulcus limitans as seen in the spinal cord, is retained almost unchanged, but the lateral walls are everted. This causes a pronounced expansion of the roof plate, closing the central canal dorsally, and a widening of the canal to the fourth ventricle. The roof plate of the myelencephalon is reduced to a single layer of ependymal cells that is covered by mesenchymal cells forming the pia mater. Active proliferation of the vascular mesenchyme produces a number of sac-like invaginations into the underlying fourth ventricle. They form a choroid plexus, which produces the cerebrospinal fluid.
As a result, instead of being arranged dorsoventrally, the alar and basal plates come to lie in the floor of the hindbrain like the pages of an open book so that the efferent areas of the basal plates become situated medially to the afferent areas of the alar plates. The cavity of this part of the myelencephalon (posterior part of the future fourth ventricles) becomes rhomboid shaped.
The basal plates, as in the spinal cord, contain the nuclei (aggregations of neuron cell bodies) of efferent nerves. On each side, these nuclei are arranged into three groups (Table 10-3). The first is the medial general somatic efferent group, represented by neurons of the hypoglossal (XII) and accessory (XI) nerves, a cephalic continuation of the ventral horn of the spinal cord. This general somatic efferent group expands rostrally into the mesencephalon and is also called the general somatic efferent motor column. The second group is the intermediate special visceral efferent group, represented by neurons that innervate muscles derived from the pharyngeal (branchial) arches (the glossopharyngeal (IX), vagus (X) and accessory (XI) nerves innervating the musculature of the third and fourth pharyngeal arches). The third, lateral general visceral efferent group, is represented by neurons of the vagus (X) and glossopharyngeal (IX) nerves. The axons of the vagus neurons supply the thoracic and abdominal viscera and the heart while the axons of the glossopharyngeal neurons supply the parotid gland.
Table 10-3: Functional regions in the brain and spinal cord
Alar plate (afferent or sensory) | ||
Basal plate (Efferent motor or autonomic) |
Neuroblasts of the alar plates in the myelencephalon migrate into the marginal zone and form isolated areas of grey matter, the gracile nuclei medially and the cuneate nuclei laterally. These nuclei are associated with the corresponding tracts ascending from the spinal cord within the funiculus dorsalis. Another group of neuroblasts from the alar plates migrates ventrally and forms the olivary nuclei. Yet other neuroblasts of the alar plates cluster into nuclei that are arranged in four columns at each side. From lateral to medial, these are: (1) special somatic afferent, receiving impulses from the inner ear; (2) general somatic afferent, receiving input from the surface of the head; (3) special visceral afferent, receiving input from the taste buds, and (4) general visceral afferent, receiving impulses from the viscera.
Metencephalon
The metencephalon represents the anterior portion of the rhombencephalon (Figs 10-17, 10-18). It develops into two main parts: the pons, a transverse structure demarcating the anterior end of the medulla oblongata, and the cerebellum, a phylogenetically newer and ontogenetically later developing structure, acting as a coordination centre for posture and movement. In mice it has been shown that the development of these structures depends on the expression of the gene engrailed-1 in this area during early development.
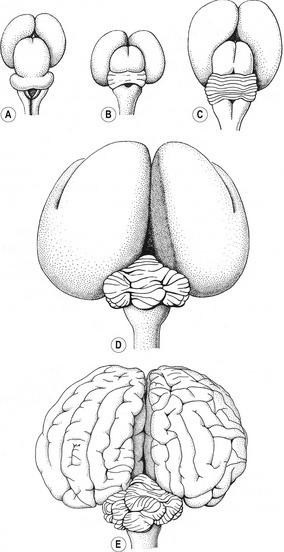
Fig. 10-17: Prenatal development of the bovine cerebrum and cerebellum. A: Day 60; B: Day 65; C: Day 80; D: Day 120; E: Day 180.
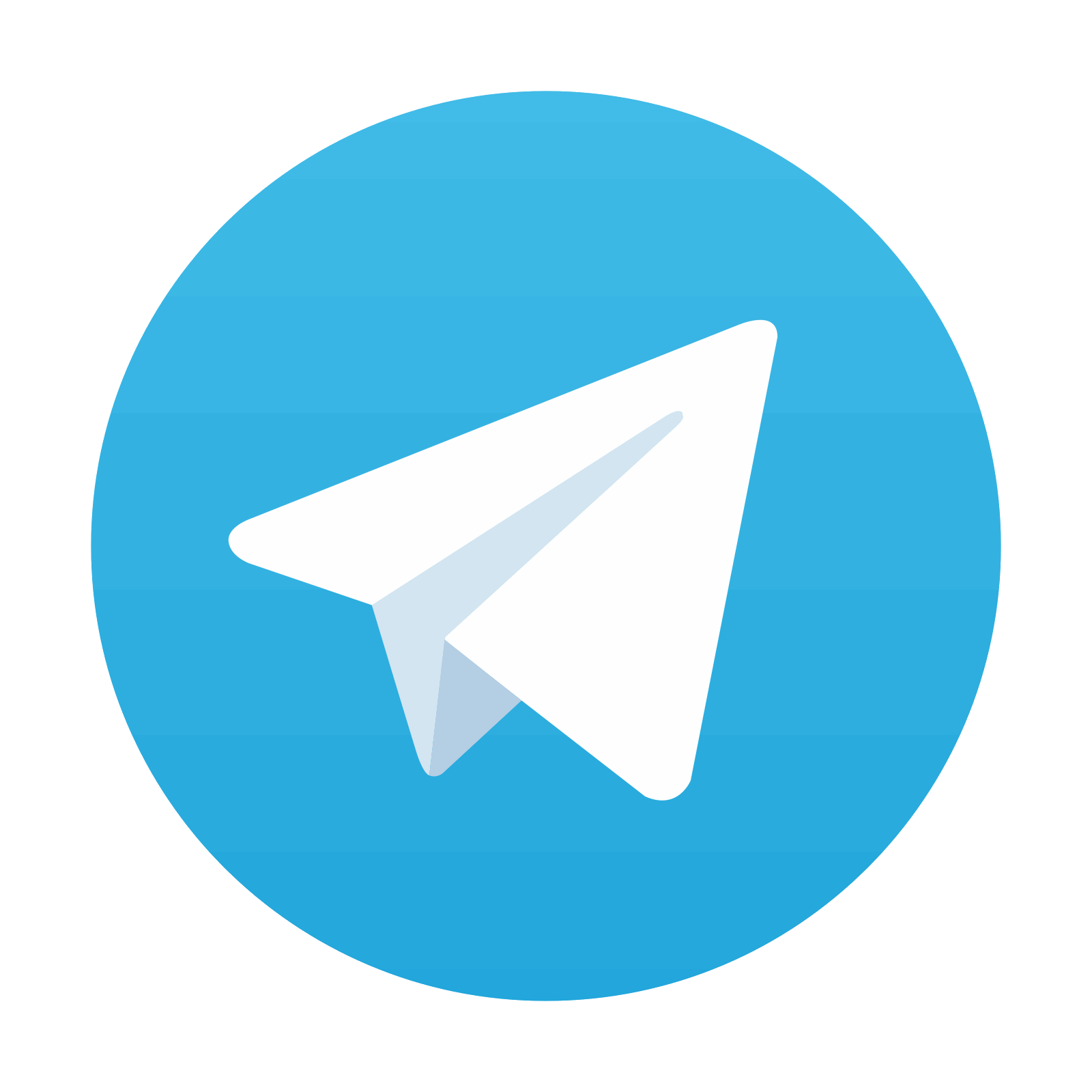
Stay updated, free articles. Join our Telegram channel

Full access? Get Clinical Tree
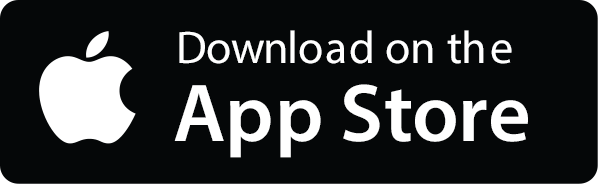
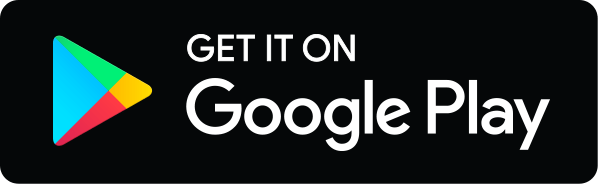