Nutritional Requirements
Llamas and alpacas are well adapted to survive and flourish in the harsh high-altitude environment of South America. Nutritional challenges in their native environment encompass yearly cycles of “feast and famine” as a result of a brief 3-month rainy season producing an abundance of high-quality forages, followed by a longer dry season during which forage nutrient availability and quality are limited.1 Traditional pastoral management of llamas and alpacas focuses on aligning the birthing season with the beginning of the rainy season to match forage availability and quality with increasing nutrient requirements. Higher mortality and poor reproduction or growth is often associated with females and their crias when parturition is misaligned relative to the rainy season. Although llamas and alpacas have been domesticated for several thousands of years, the pastoral management approach has resulted in minimal scientific publications describing nutrient requirement prediction models at various physiologic states.
Importation of llamas and alpacas to North America, Europe, and Australia has placed greater importance on understanding nutrient requirements to develop well-balanced feeding programs that will optimize animal health and performance while minimizing disease risks. The first in-depth review of South American camelid (SAC) nutritional requirements was published in 1989.1 In this review, only one study was cited for the determination of energy requirement for llama maintenance and protein requirement for alpaca maintenance.2,3 Energy and protein requirements for other physiologic states were not available. Additionally, no reports describing mineral and vitamin requirements were included. Much of the review focused on comparative digestive anatomy and digestive efficiency between SACs and other ruminants, highlighting the competitive advantage of llamas and alpacas with lower-quality forages (see Chapter 8). Furthermore, numerous studies characterizing feeding behavior, feed selectivity, and intake capacity were cited, consistent with the pastoral approach to feeding management of llamas and alpacas.
Following this review, a second study on energy requirement for llama maintenance finding a very different result from the original study was published.4 Fowler first suggested energy requirements for other physiologic states based on extrapolations from data on goats.5 With these limited data and a framework from extrapolated requirements, a series of models to predict energy and protein requirements for llamas and alpacas for all physiologic states were developed.6 These models mimicked similar factorial approach to defining requirements for sheep and goats but were adjusted to the documented lower energy and protein requirements for llamas and alpacas. The recent National Research Council (NRC) publication describing nutrient requirements for small ruminants included these models to describe llama and alpaca nutrient recommendations.7 It needs to be emphasized that these models are, at best, estimations based on available data and have been evaluated in comparison with previous recommendations and current successful feeding methods, but they have not been robustly validated with animal feeding trials.
With the paucity of information, it has been assumed that no differences in nutrient requirements exist between llamas and alpacas. Recent North American studies determined a slightly higher maintenance protein requirement for alpacas compared with the original study and a higher protein requirement for llamas.8,9 This latter study suggested that protein requirements could not be equally applied to llamas and alpacas. A review of feeding recommendations from Chile recommended different energy requirements for llamas and alpacas, and both recommendations are lower than the energy requirement reported for llamas from a North American study.10 A pattern of determined lower energy and protein requirements from South American data, compared with North American data, seems to exist. These differences have been interpreted to suggest a greater nutrient assimilation efficiency at higher (>3000 meters [m]) compared with lower altitudes, although this hypothesis has not been adequately tested.11 Animal owners and veterinarians should become familiar with the nutrient requirements of llamas and alpacas not only to provide an appropriate diet but also to better understand and recognize risks for nutritional diseases. However, it is important to recognize the limitations of these recommendations, as a result of our knowledge of llama and alpaca nutrient requirements is incomplete, especially in light of more recent findings.
Water
Water plays an important role in body temperature regulation and provides an aqueous medium to sustain all metabolic reactions. Total body water will decrease with age and body fat content. The body desiccates with age; newborn animals have more than 80% body water content compared with adult animals that have between 60% and 70%. Total body water measured in four 3-to-6 year old llamas weighing between 62 and 149 kg ranged from 70.4% to 63.8%, respectively.12 Similar total body water content (68%) was reported in free-ranging alpacas and llamas.13,14 The latter study showed higher body water content during summer (71.8%) compared with winter (65.9%) and much higher body water content in lactating females (78.3%), which might be explained by fat mobilization in support of lactation.14 These values are consistent with observations based on observed body weight range in other species accounting for variation in body fat content.
Total body water is distributed between intracellular (62%–69%) and extracellular (31%–38%) physiologic pools.7 The intracellular water pool is highly regulated through exchange with the extracellular pool via modification of sodium ion flux to maintain a fairly stable sodium concentration. The extracellular water pool comprises blood plasma (25%) and interstitial fluids (75%). Water contained within the gastrointestinal (GI) tract is another important body water reservoir that may be associated with the extracellular pool. SACs, similar to other ruminant species, have significant GI water reserves that can be exchanged with the extracellular pool to maintain hydration status. Foregut water content is highly variable (10%–30% of total body water) as a result of dry matter (DM), mineral, water, and saliva influx, balanced with digestion and fermentation end product generation and outflow, but accounts for a fairly constant 81% to 88% of total gut water.7
Animals derive water from free water, feed, and metabolic sources. Metabolic sources (e.g., oxidation reactions from food digestion and body tissue breakdown) generally account for 10% or less of total needs, except in desert-adapted species, in which metabolic water may account for more than 30% of water needs. Feeds vary tremendously in water content, from less than 15% in most dry feeds to more than 80% on lush pasture. Voluntary water consumption (liters per kilogram [L/kg] DM consumed) is influenced by the composition and character of the consumed diet relative to animal water requirements. Water intake is highest with dried forage and slightly less for pelleted or coarse grains. Forage moisture content and ambient temperature are the primary determinants of voluntary water consumption (Figure 9-1). A study showed that voluntary water consumption increased from 3 to 5 L/kg DM over an ambient temperature range of 40°F to 80°F, respectively, when dried forage was consumed (10% moisture). Conversely, water consumption ranged from 0.1 to 2.2 L/kg DM over the same temperature range when forage containing 75% moisture was consumed.15 Although these data were obtained using grazing steers at a maintenance intake, they are consistent with observations in other ruminants and applicable to SACs.
Figure 9-1 Effects of forage water content and ambient temperature on water consumption. (Adapted from Hyder DN, et al: Sampling requirements of the water-intake method of estimating forage intake by grazing cattle, J Range Manage 21(6):392-397, 1968.)
Water loss from the body occurs primarily via urine and feces, although small ruminants have reduced fecal moisture as a result of greater colonic water absorption. Adaptation to water deprivation comes from the ability to concentrate urine. Although SACs are not similar to Old World camels in their ability to adapt to water deprivation, SACs can concentrate their urine, decrease renal water excretion, and increase oxidative water production, thus facilitating their adaptation of water restriction.12 Additional insensible water losses are attributed to sweating, respiratory evaporation, and licking or drooling.7 For physiologic states beyond maintenance, water loss is associated with milk production (milk is 85%–87% water), whereas body water accretion occurs with tissue gain (muscle is 75% water) and pregnancy.
Inadequate water intake results in reduced feed intake and reduced water output. However, llamas were observed to have less reduction in DM intake compared with goats when water was restricted.12 Urinary volume would dramatically decline with water restriction via mechanisms to concentrate urine. Llamas can concentrate their urine to a greater extent (>1500 milliosmoles per liter [mOsm/L]) compared with other ruminant species (approximately 500 mOsm/L).7,12 Fecal water content is also reduced with water restriction, although higher dietary protein content minimizes this response. More severe water restriction relative to requirement results in reduced ability to sweat and salivate. Water restriction results in lower milk yield and growth rate, although these responses may be further exacerbated by reduced DM intake. Severe or prolonged water restriction exceeds the capability of compensatory mechanisms to maintain physiologic water pools, resulting in progressive degrees of dehydration. Described clinical manifestations of water restriction typically are associated with less than 5% dehydration. As dehydration progresses to 8%, animals become depressed, weak, have dry mucous membranes, and have slower capillary refill time. Further progression to 10% or 12% dehydration results in the animals becoming recumbent, comatose, having a rapid heart rate with weak pulse, and dying without aggressive fluid replacement therapy. Neonates are more sensitive to dehydration because of their higher body water content compared with adult animals.
Water Requirements
Total water requirement is determined by body weight, physiologic state, level of activity, production level, dietary composition, and environmental conditions. Animals maintained on pasture have nearly twice the water turnover rate compared with indoor housed animals. The numerous factors influencing a given animal’s water needs confound our ability to generate specific requirement models (see NRC for review).7 Using isotope dilution methods, water turnover rate can be measured, and this value is assumed to be equivalent to the animal’s total water intake or loss assuming a steady state balance. Only one study measured water turnover rate in llamas. Under confinement in a temperature-neutral environment, adult llamas had higher water turnover rate (62.1 ± 8.8 milliliters per kilogram of body weight [mL/kg BW0.82]) compared with goats (59.0 ± 10.9 mL/kg BW0.82).9 In this same study, llamas maintained on pasture had a water turnover rate nearly twice (122.2 ± 29.8 mL/kg BW0.82) that of confined animals. A similar water turnover rate (116 ± 5 mL/kg BW0.82) was determined in free-ranging llamas, whereas a higher rate (151.8 ± 28 mL/kg BW0.82) was reported for free-ranging alpacas.13,14 These higher values are consistent with water turnover rates in grazing sheep and goats. Water requirement can be estimated relative to energy expenditure or DM intake.7 Estimates for water requirements for SAC maintenance in a thermoneutral environment have been related to energy intake (1 milliliter per kilocalorie [mL/kcal] of metabolizable energy intake) or energetic requirement based on metabolic body weight (122 mL/kg BW0.75).6,16 The former estimate is essentially equivalent to the observed water turnover rate for confined animals, whereas the latter model predicts slightly lower water requirements than the water turnover rate measured in pastured llamas (Table 9-1).
TABLE 9-1
Comparison of Models Estimating Maintenance Water Requirement and Extrapolated Water Requirement for Other Physiologic States under Isothermic Conditions
*ME = 1 mL water per 1 kcal of metabolizable energy; Confined = 62.1 mL/kg BW0.82; Pasture = 122.2 mL/kg BW0.82; MBW = 122 mL/kg BW0.75; 2.5 × MBW = 182.1 mL/kg BW0.75.
Few reports have documented additional water requirements for physiologic states beyond maintenance for SACs, so some extrapolation from other species is necessary. Water requirement is increased between 30% and 70% above maintenance in the final 2 months of pregnancy in goats and sheep, respectively.7 Given that sheep seemingly have a much higher pregnancy water requirement compared with other small ruminants, a suggested increase of 30% to 50% above maintenance should be reasonable for SACs. Water loss in support of milk production in goats was estimated at 1.43 kg/kg of milk yield. Other studies have shown a water requirement for lactating small ruminants to range between 412 and 433 mL/kg BW0.75 housed on pasture in warmer weather.7 One study reported the water turnover rate in free ranging lactating llamas as 396 ± 9 mL/kg BW0.82 (equivalent to 552.5 mL/kg BW0.75) under pastoral conditions.14 This higher requirement relative to other small ruminants may reflect lower body fat composition during lactation in llamas and higher metabolic rate associated with extensive pasture management. Additional required water in support of growth has been evaluated in various small ruminant species. Suggested water requirements for nursing and weaned animals are 8 to 13 mL/g BW gain and 7 to 8 mL/g BW gain, respectively.7 Incorporating these suggested water requirements for gain with maintenance, nursing animals gaining between 100 and 400 g/day have an estimated water requirement of 255 to 316 mL/kg BW0.75, whereas weaned animals gaining 200 to 400 g/day would have a water requirement of 244 to 348 mL/kg BW0.75.7 The range in daily gain for nursing animals is consistent with observations in growing llamas and alpacas; however, this rate of gain for weaned animals would be higher than that observed in llamas and alpacas.
The goal for a water program is to provide water in amounts that would exceed voluntary consumption under given environmental conditions to ensure adequate water availability. Although water consumption is highly associated with DM intake (see Figure 9-1), accurate intake determination for llamas and alpacas is difficult under typical group feeding systems; thus, water requirements might be best predicted relative to energy requirements. A comparison of various maintenance water requirement models and suggested models using metabolic body size to estimate SAC water requirements over various physiologic states are shown in Table 9-1. These models are assuming an isothermic ambient temperature (50°F) and will need to be adjusted for differing ambient temperatures (see Figure 9-1). In comparing maintenance water requirements (Figure 9-2), the confined model is the minimal water requirement and could be used to estimate water needs for sick or strictly confined animals. Animals that are confined or managed in dry lots would have a water requirement estimated by the 122 mL/kg BW0.75 model, whereas pasture-based animals have a higher requirement (182.1 mL/kg BW0.75). For the final 3 months of pregnancy, water requirements should be increased by 50% above either confinement or pasture maintenance requirements, depending on the management system. The lactating water requirement (552.5 mL/kg BW0.75) was based on a single reported study, but adjusted to a metabolic body weight basis. With no camelid data for a basis, recommended water requirements are based on described NRC models for nursing animals with a range of growth from 100 to 400 g/day.
Figure 9-2 Comparison of different models estimating maintenance water requirements for llamas and alpacas. Confined and pasture estimates. (Based on data from Rübsamen K, von Engelhardt W: Water metabolism in the llama, Comp Biochem Physiol 52A:595-598, 1975; model based on metabolic body weight (MBW) from Van Saun RJ: Nutritional diseases of South American camelids, Small Rumin Res 61:153-164, 2006.)
Fresh clean, high-quality water should be accessible for free-choice intake at all times. At least two watering stations should be provided for each pen of animals to prevent water access to socially inferior animals being restricted by the socially superior animals within a group. Minimal documented information is available regarding water space requirements for SACs. At least 12 inches of water trough space per ewe or goat has been recommended; however, this greatly exceeds water station size recommendations for dairy cattle (36 inch per 10–15 cows).17 If two watering stations are present in a pen, then 2.5 to 3.5 inches of water space per animal should be sufficient. SACs suck water with a slightly opened mouth and generally adapt to most open surface watering units.
Water Quality Assessment
Water is the most essential, although often the most neglected, nutrient. A short time frame exists between inadequate water intake and clinical manifestations of water deficiency. Required amounts and availability of water, as in the case of other essential nutrients, are not the only issues to be addressed; water quality must also be addressed. Water quality can be evaluated subjectively by its color, taste, and smell. High-quality water should be colorless and have no offensive odor or taste. A “rotten egg” smell to water is the result of high sulfate (odorless) content, in which sulfate ion is reduced to produce hydrogen sulfide (H2S) gas, which gives out the obnoxious smell. Determinations of pH, total dissolved substances (TDSs), mineral content and hardness, contaminants (herbicides, pesticides, organic compounds, etc.), and microbiologic culture provide objective measures of water quality. Water that meets current standards for human consumption obviously is equally satisfactory for animal consumption. The question is the tolerance level of animals relative to their willingness to consume adequate amounts of water that does not meet current human standards and not experience adverse consequences. Definitive evidence is minimal regarding the lower tolerance level of water quality for animals, especially for microbial contamination. A number of publications addressing livestock water quality generally describe experiential assessment of water quality for livestock.18–20
Increasing salinity of water sources, irrespective of mineral sources, results in decreased water intake, transient diarrhea, and reduced performance because of lower feed intake (Table 9-2). Younger animals and lactating animals are most sensitive to water salinity. Most animals can acclimate to moderately saline water. High levels of TDSs in a water sample would suggest that further evaluation of mineral content is warranted, although low levels of TDSs water could contain inappropriate mineral content. The most common dissolved minerals include calcium, magnesium, sodium, sulfate, and bicarbonate. Water hardness, a measure of calcium and magnesium content, is not a significant health or water intake issue. Hard water may contribute additional calcium or magnesium to the diet but does not increase urolithiasis risk. Beside potential toxic minerals that may contaminate water (Table 9-3), dissolved substances may affect aesthetic quality, which may lead to decreased intake; provide an additional mineral source to the diet potentially altering available mineral balance; or interfere with other minerals thus altering dietary mineral availability. Iron, manganese, and sulfate content of water are of primary concern, as they have an adverse impact on aesthetic qualities and also interfere with other minerals. Excessive iron and sulfate intake may adversely affect dietary copper availability, although by separate mechanisms. High sulfate intake may induce polioencephalomalacia that is nonresponsive to thiamin supplementation. Nitrates are another potential water contaminant of concern, especially in ruminant animals, in which nitrate is converted to more toxic nitrite by ruminal microbes. Absorbed nitrites will bind to hemoglobin reducing the blood’s oxygen carrying capacity, which results in varying stages of anoxia in affected animals. Although water nitrate content generally is not solely responsible for animal intoxication, it can significantly contribute to dietary nitrate content in predisposing to potential intoxication (Table 9-4).
TABLE 9-2
Guidelines for Water Salinity Interpretation as Measured by Total Dissolved Solids Content or Electrical Conductivity
TDS Mg/L or ppm | Electrical Conductivity Umhos/cm | Interpretation |
<1000 | < 1670 | Relatively low salinity; suitable for all classes of livestock |
1000–2999 | 1670–5,008 | Satisfactory for all classes of livestock; may produce transient diarrhea in animals not accustomed to them but should not affect health or performance |
3000–4999 | 5010–8,348 | Temporary water refusal and diarrhea may be seen when animals are introduced to them; may reduce productivity in lactating animals |
5000–6999 | 8350–11,688 | Likely to reduce productivity in lactating animals; may reduce growth rates; may result in water refusal and diarrhea; avoid in lactating and pregnant animals; avoid, if possible |
7000–10,000 | 11,690–16,700 | Unfit for poultry or swine; considerable risk in all other species; animals may subsist on them in low stress situations; avoid unless extreme circumstances |
> 10,000 | >16,700 | High saline water; not recommended for use under any conditions; dangerous, avoid at all costs |
mg/L, milligram per liter; TDS, total dissolved solids; Umhos/cm, micro-ohms per centimeter.
Modified from National Research Council: Nutrient and toxic elements in water, Washington, DC, 1974, National Academy Press; Pfost DL, et al: Water quality for livestock drinking, Bull EQ 381, University of Missouri-Columbia Extension, 2001; and Bagley CV, et al: Analysis of water quality for livestock, Bull AH-Beef-28, Utah State University Extension, 1997)
TABLE 9-3
Recommended Limits of Concentration of Some Potentially Toxic Substances in Water
Mineral | Safe Upper Limit Mg/L (ppm)* | Comment |
Aluminum (Al) | 5.0 | Potential toxicant, Interfering agent |
Arsenic (As) | 0.2 | Toxicant |
Boron (B) | 5.0 | |
Cadmium (Cd) | 0.05 | Toxicant |
Chloride (Cl) | <300 | High values of concern when not balanced with sodium |
Chromium (Cr) | 1.0 | Toxicant |
Cobalt (Co) | 1.0 | |
Copper (Cu) | 0.5 | Potential toxicant |
Iron (Fe) | 2.0 | Aesthetic issues, Interfering agent |
Fluoride (F) | 2.0 | Toxicant |
Lead (Pb) | 0.05 | Toxicant |
Manganese (Mn) | <0.1 | Aesthetic issues, Interfering agent |
Mercury (Hg) | 0.01 | Toxicant |
Nickel (Ni) | 1.0 | Toxicant |
Nitrite (NO2) | <33 | Toxicant |
Nitrite-N (NO2-N) | <10 | Toxicant |
Selenium (Se) | 0.05–0.1 | Potential for intoxication |
Sulfate (SO4−2) | <300 | Aesthetic issues, Interfering agent, Potential toxicant |
Vanadium (V) | 0.1 | Toxicant |
Zinc (Zn) | 25.0 | Potential toxicant, Interfering agent |
*Milligrams per liter = mg/L; ppm = parts per million; both are equivalent measures
Modified from National Research Council: Nutrient and toxic elements in water, Washington, DC, 1974, National Academy Press; Pfost DL, et al: Water quality for livestock drinking, Bull EQ 381, University of Missouri-Columbia Extension, 2001; and Bagley CV, et al: Analysis of water quality for livestock, Bull AH-Beef-28, Utah State University Extension, 1997.
TABLE 9-4
Interpretation of Water Nitrate Contamination
Nitrate (NO3) | Nitrate Nitrogen (NO3 –N) | Interpretation |
0–44 ppm | 0–10 ppm | No harmful effects |
45–132 ppm | 10–20 ppm | Safe if diet is low in nitrates and nutritionally balanced |
133–220 ppm | 20–40 ppm | Could be harmful if consumed over long periods |
221–660 ppm | 40–100 ppm | Ruminant animals at risk; possible death and pregnancy losses |
661–800 ppm | 100–200 ppm | Unsafe; high probability of death losses |
> 800 ppm | >200 ppm | Unsafe; do not use |
Modified from National Research Council: Nutrient and toxic elements in water, Washington, DC, 1974, National Academy Press; Pfost DL, et al: Water quality for livestock drinking, Bull EQ 381, University of Missouri-Columbia Extension, 2001; and Bagley CV, et al: Analysis of water quality for livestock, Bull AH-Beef-28, Utah State University Extension, 1997.
A zero tolerance level is expected for microbiologic contamination of human water sources. Animals, however, seemingly tolerate low to moderate bacterial loads in their water supply. Younger animals are perceived to be less tolerant than older animals to coliform contamination of water. Although no definitive criteria have been established, total bacteria, fecal coliform, and concentrations of fecal streptococcal organisms exceeding 1 million—less than 10, and less than 30 organisms per 100 mL, respectively—are considered potential problem levels.19 Water poses a risk for transmission of various pathogenic organisms (i.e., cryptosporidia, coccidia, leptospira, and fecal coliforms) if contaminated by the urine or feces of infected animals. During summer months, surface water sources may become contaminated with blue-green algae, which, if consumed, may produce hepatotoxins capable of causing severe liver disease and death.
Energy
Dietary energy supports all body functions and thus is quantitatively considered the most important nutrient from the perspective of animal performance. Unlike other essential nutrients, energy is not described by a single physical entity such as protein, calcium, or selenium. Rather, energy is an abstract entity that can only be measured in its transformation from one form into another.21 In nutrition, chemical energy is measured by heat production as molecules are oxidized, thus energy is quantified as calories or joules (1 calorie [cal] = 4.184 joules [J]). One calorie is defined as the amount of heat required to raise 1 mL of water from 58°F to 60°F. From a practical standpoint, a calorie is a very small unit of energy, therefore larger units termed kilocalories (kcal; 1 kcal = 1000 calories) and megacalories (Mcal; 1 Mcal = 1000 kcal) are used to describe energy requirements.
Energy derived from the diet provides resources in support of body maintenance and productive functions. SACs, like any other species, experience the full spectrum of dietary energy intake relative to requirements ranging from deficiency (starvation) to excess (obesity). Inadequate energy intake (e.g., negative energy balance) is of greatest concern, as it results in a range of adverse consequences with regard to animal health and performance. Immediate effects of energy deficiency manifest as lower milk production in lactating animals or slower daily gain in growing animals, whereas impaired immune response can be seen in any energy-deprived animal. These consequences may be recognized before obvious changes in BW or body condition score (BCS; refer to Chapter 12 for description of scoring system) are measured. More severe or prolonged energy deficiency is quantified by marked reductions in BW and BCS and characterized by greater impairment of growth, production, health (i.e., greater disease susceptibility), and reproductive capacity (i.e., delayed puberty, decreased fertility).
Energy consumed in excess of requirements (e.g., positive energy balance) results in energy reserve deposition as adipose tissue. Increasing BCS or BW may directly or indirectly, respectively, quantify accumulating energy stores and describe progressive stages of obesity. Severe obesity (BCS >4 on a 1–5 scale; >30% overweight) has obvious associated health risks related to musculoskeletal, metabolic, and reproductive diseases. However, even moderate obesity (BCS 3.5–4 on a 1–5 scale; >15%–20% overweight) may have adverse effects on metabolic health and immune function. Obesity is more common in SACs managed outside of South America, most likely because of higher-quality forages being more readily available year round in those countries. With unique metabolic conditions of low insulin secretion and insulin insensitivity (refer to Metabolic Disease discussion, Chapter 41), SACs may be more predisposed to metabolic diseases associated with inappropriate excess or deficient energy intake.
Energy Partitioning
During digestion, energy stored within chemical bonds of carbohydrate, fat, and protein molecules is released in varying degrees and made available to the animal. Gross energy (GE) is the total amount of energy in a feed measured as heat released during total combustion. GE is categorized into definable components, namely, digestible, metabolizable, and net energy, based on the proportion of GE available to the animal at different phases of the digestion and utilization process.21 Digestible energy (DE) is that portion of GE that is not excreted in feces. Metabolizable energy (ME) is the portion of DE that is not lost in urine or as fermentation gases. ME is available to the animal’s tissues and is used for various productive functions. Although ME would be a more descriptive measure of animal performance based on energy availability, combustible gas production is fairly consistent (approximately 18% of DE) in nonruminant animals and ruminants at maintenance. Therefore, ME is often approximated as 0.82 × DE; however, this relationship may vary considerably, depending on diet composition and level of feeding. Finally, net energy (NE) is the proportion of ME used to maintain body physiologic functions (maintenance) or productive condition (e.g., tissue growth, pregnancy, lactation, fiber growth). The difference between ME and NE is heat production following heat losses during digestion and metabolism of a given nutrient (heat increment) and heat losses from fermentation. Efficiency of conversion of ME to NE also depends on the productive purpose. Pregnancy is the least efficient process (approximately 12%–15% ME energy conversion to fetal energy deposition), whereas maintenance and lactation are relatively efficient (67%–72% conversion). Conversion efficiency for growth will depend on tissue composition (fat versus protein) that is being deposited.
Dietary Energy Sources
Carbohydrates
Carbohydrates are a very diverse compilation of organic compounds that comprise more than 70% of the SAC diet. Categorization of dietary carbohydrates into structural and nonfiber groupings is determined by their solubility in neutral detergent solution.22 Structural carbohydrates are insoluble in neutral detergent and include cellulose and hemicellulose. These complex fiber carbohydrates are structural components of the plant’s primary and secondary cell wall and, with the cementing phenolic compound lignin, provide rigidity and support to the plant. Cellulose undergoes very slow fermentation by microbial cellulases, whereas hemicellulose fermentation ranges from moderate to slow, depending on the degree of lignification between cellulose and hemicellulose in the secondary cell wall.22 Nonfiber carbohydrates are soluble in neutral detergent and range from simple sugars to very complex fiber compounds that include pectic substances, β-glucans, and fructosan polysaccharides. Pectic substances and β-glucans are components of the middle lamellar layer between plant cell walls and are moderately to rapidly fermented. Simple sugars and complex oligomers of glucose (starch) and fructose (fructosans) are located within the plant cell cytosol. Sugars and starches are the only carbohydrates amenable to enzymatic digestion (i.e., digestible carbohydrates) by the host animal, whereas fructose oligomers or larger polysaccharides are very rapidly fermented.
Collectively, carbohydrates account for the greatest energy source in the SAC diet. As with other forestomach fermenting animals, the primary energy source for the host animal is volatile fatty acids (VFAs), as little digestible carbohydrate presents to the small intestine because of efficient forestomach microbial fermentation. Acetate, propionate, and butyrate are the primary end products of carbohydrate fermentation and comprise the primary VFAs available to the animal for energy utilization. Sugar and starch fermentation generates a greater amount of propionate, which is exclusively converted to glucose in the liver. In addition to propionate, digestible carbohydrate fermentation results in lactic acid production that can accumulate, resulting in reduced pH and impaired fiber fermentation within the fermentation system. Fiber fermentation generates relatively more acetate and butyrate, substrates for tissue oxidation for energy or conversion to fats and utilized by tissues. SACs are well adapted to consume diets predominated by structural carbohydrates. Amounts of dietary sugar and starch carbohydrates are not significant components of the SAC diet in their native habitat, and need to be limited relative to structural carbohydrates to prevent excessive acid production and altered microbial population balance in the forestomach.
Fats
Fats are not a significant energy source for SACs, since they are not present in large amounts in a forage-based diet (<4% of DM). Endogenous fat compounds in forages are predominantly nontriglyceride compounds and thus are not energy resources to the animal. Ruminant animals, because of their forestomach fermentation, are not very tolerant of high fat diets compared with nonruminant animals. Nonruminant animals tolerate and efficiently digest diets containing very high fat content (>20% DM). Free dietary fatty acids, especially polyunsaturated fatty acids (PUFA), in the rumen are inhibitory to fiber fermenting microbes.23 Forestomach microbes detoxify dietary PUFA through biohydrogenation ultimately resulting in saturated fats, which are less toxic to the microbes.24 Fatty acid biohydrogenation accounts for the exclusive presence of saturated fats in ruminant animals. Under some feeding conditions, microbial biohydrogenation is incomplete, and various isomers of trans fatty acids are generated.24 Trans fatty acid isomers may have many metabolic effects, ranging from decreased fat synthesis to altered insulin and glucose metabolism.
Information about fats in the SAC diet is nonexistent, so extrapolation from other ruminant feeding practices is necessary. High-producing dairy cattle can tolerate diets up to 9% (DM) crude fat, although not all from the same source. Fat supplementation practices suggest categorizing dietary fat sources into three groupings: (1) endogenous plant fats, (2) vegetable oil sources, and (3) rumen inert fats. Vegetable oils, namely, soybean, corn, flaxseed, canola, or cottonseed sources, contain large amounts of polyunsaturated fats and are the most problematic relative to the forestomach system. Inert fat sources are saturated fats and modified fatty acids (i.e., calcium soaps), which minimize any potential effect on forestomach microbial populations and provide fats to the small intestine for digestion and absorption. Current recommendations for feeding fat to ruminants are to balance the amounts of fat sources across the three defined groups. With endogenous plant fat accounting for 2% to 3% (DM), an additional 2% to 3% (DM) can come from vegetable fat sources followed by another 2% to 3% (DM) from inert fat sources. Efficacy of feeding inert fat sources to SACs is presently unknown. The amount of vegetable fat supplementation is dependent on diet composition where forage-based diets are more adversely affected by fat supplements compared with high grain diets.25 A supplementation rate of 3% or less (DM) for vegetable fat sources to high-forage diets is recommended.25
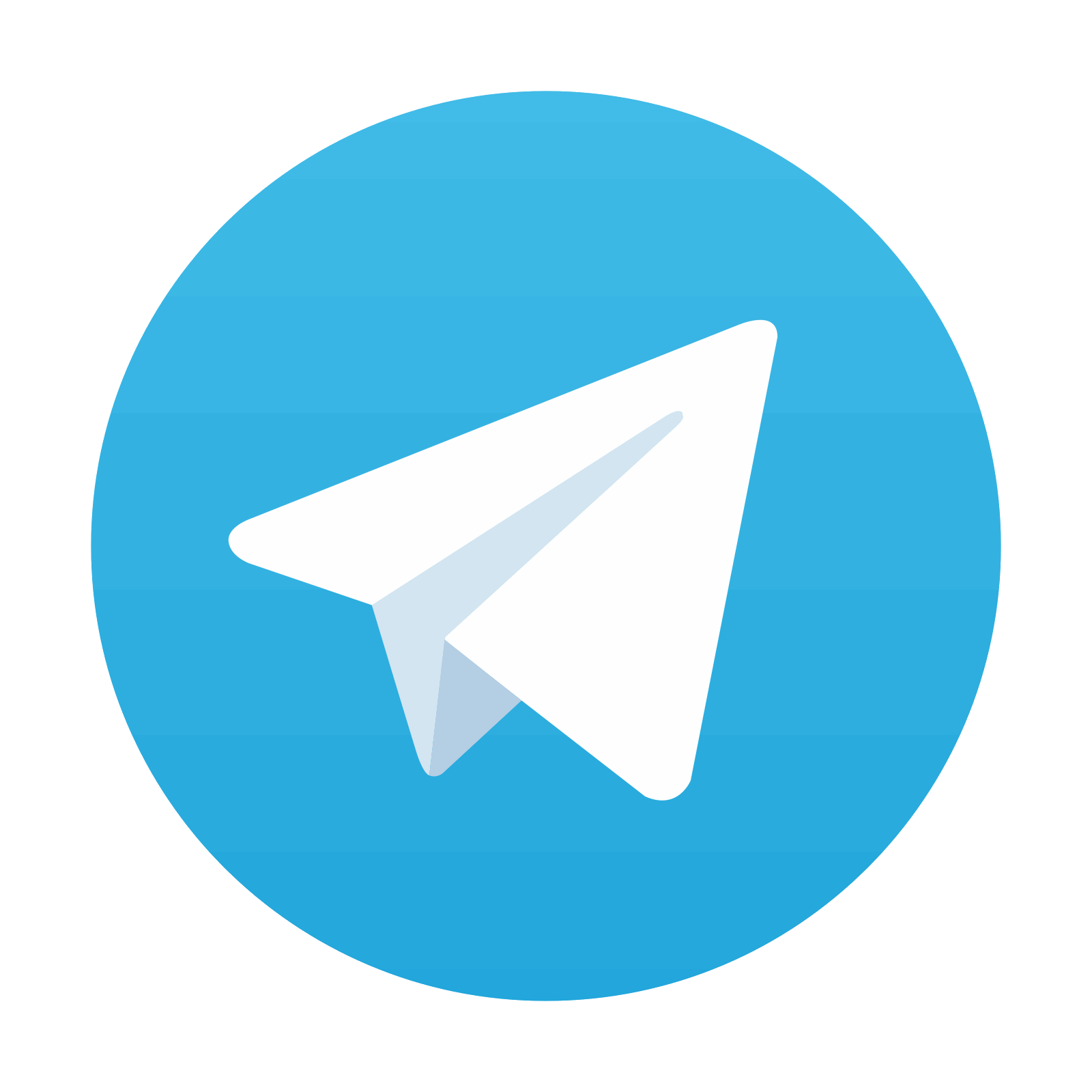
Stay updated, free articles. Join our Telegram channel

Full access? Get Clinical Tree
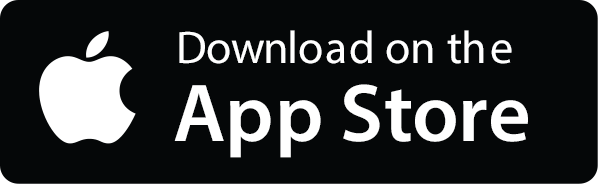
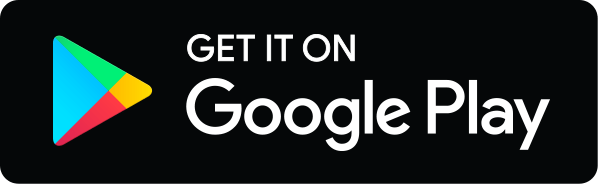