Nutritional Assessment
Nutritional requirements were defined in Chapter 9, feeds and their composition were characterized in Chapter 10, and feeding management practices were described in Chapter 11. However, how can the integration of these components be assessed with regard to the nutritional adequacy of a feeding program? An obvious measure of nutritional adequacy is observed good animal health and performance, but quantitative measures must be used for evaluation. Given the stoic nature of llamas and alpacas and the fiber coat masking obvious physical changes, a proactive nutritional monitoring program should be undertaken. Further confounding the assessment process are many external factors, most importantly environmental influences, on an animal’s response to a nutritional program.
Nutrient Composition Analysis
In many situations, qualitative assessment of forage quality is used in lieu of nutrient analysis. Organoleptic assessment of forage quality is based on identifying properties of plant maturity, leaf-to-stem ratio, and foreign object presence using visual and tactile senses as well as distinguishable smells indicative of potential problems (Table 12-1). Though sensory assessment might provide some insight relative to forage quality, it does not provide any quantitative information on forage nutrient content. Routine and specialized chemical measures are considered the gold standard for evaluating forage quality and its ability to meet an animal’s nutrient needs. Chemical measures of crude protein (CP) and neutral (NDF) and acid (ADF) detergent fiber best characterize forage quality at differing physiologic growth stages (Table 12-2).
TABLE 12-1
Comparison of Essential Nutrients, Feed Chemical Composition, and Analytical Testing Procedures
*Lignin is not truly a carbohydrate compound but is so intimately associated with cell wall carbohydrates that it is often included as such.
†Enzymatic methods used to determine sugar and starch content.
TABLE 12-2
Sensory Evaluation in Assessing Forage Quality
Sensory Evaluation | Description/Comments |
Visual | |
Stage of maturity | Look for the presence of seed heads (grass forages) or flowers or seed pods (legumes), indicating more mature forages. |
Leaf-to-stem ratio | Look at forage, and determine whether the stems or leaves are more obvious; good-quality legume forages will have a high proportion of leaves, and stems will be less obvious and fine. |
Color | Color is not a good indicator of nutrient content, but bright green color suggests minimal oxidation; yellow hay indicates oxidation and bleaching from sun, and hay will have lower vitamins A and E content. |
Foreign objects | Look for presence and amount of inanimate objects (twine, wire, cans, etc.), weeds, mold, or poisonous plants. |
Touch | Feel stiffness or coarseness of leaves and stems; see if alfalfa stems wrap around your finger without breaking; good-quality hay will feel soft and have fine, pliable stems. |
Smell | Good quality hay will have a fresh mowed grass odor; no musty or moldy odors; caramel or tobacco smell to hay indicates heat damage; silage should have slight pleasant fermented smell; vinegary, sweet, alcohol, tobacco, or rancid milk odors to silage indicate an abnormal fermentation has taken place and further diagnostic testing should be completed. |
Adapted from Van Saun R: Nutrition. In Hoffman E, editor: The complete alpaca, Santa Cruz, CA, 2003, Bonny Doon Press.
Feed Sampling Procedures
To obtain usable information from feed testing reports, a representative feed sample must be collected and submitted to an accredited feed testing laboratory. The National Forage Testing Association (www.foragetesting.org) provides a listing of accredited laboratories for all of North America and other geographic regions. To best sample hay, a coring device must be used to collect the sample. Hand-grab sampling is not an appropriate method for collecting representative hay samples. The coring instrument is a long (12 to 24 inches [30 to 60 centimeters [cm]]) hollow metal tube with a sharpened edge and attaches to a hand or electric drill. The National Forage Testing Association website also provides information on hay coring devices and recommended sampling procedures. A core sample should be obtained from approximately 10% of the bales for any given forage lot. Ideally, a single sample of 200 to 225 grams (g; approximately 0.5 pounds [lb]) is obtained. The sample is packaged into an airtight plastic bag, enough to fit into a liter (L) or quart-sized bag, and submitted to the laboratory. The sample must be properly identified. If a larger amount is submitted the laboratory, the entire sample cannot be ground, which will lead to subsampling and introduction of errors in the results. Separate samples should be taken from each field and cutting for best information on forage analysis. When hay is purchased, each purchased lot should be tested unless subsequent purchases are from the original lot.
Obtaining representative pasture or browse sample is a more arduous task. The goal is to mimic the selective feeding behavior of the animals in obtaining a representative sample. Typically, pastures should be sampled prior to animals being allowed to graze; however, this may limit the ability to collect samples of forage consumed by the animals. Another approach is to randomly select areas and cut samples of forage material being consumed by the animals (observation based). To randomize the sampling process, a 12-inch (30 cm) diameter plastic ring (e.g., Frisbee ring) may be used to toss around the pasture as the selection process for collecting 10 to 20 representative samplings. It is important to not clip too far down the plant. Animals generally do not eat down to the dirt, and if dirt contaminates the sample, mineral content values will be altered (recognized as high ash and iron content in sample). For browse, it is essential to watch what the animals eat and selectively harvest similar feeds. Once sufficient material is collected, it should be placed in an airtight plastic bag and the sample frozen. Sampling silage is equally challenging. A larger volume of ensiled material should be collected from throughout the silo face. Material collected is combined, mixed thoroughly, and appropriately subsampled for submission. To appropriately subsample larger volume samples, the combined amount is divided into equal quarters and opposing quarters combined for the submitted sample. High-moisture samples such as those from pasture, ensilage, and browse should be sent in the frozen state to the laboratory.
Analytical Procedures
A range of chemical, enzymatic, and biologic analytical methods are used to evaluate nutrient composition of feeds (Table 12-3). Traditional chemical methods directly quantify nutritionally important compounds and essential nutrients important to the formulation or evaluation of an animal’s diet. However, these methods provide little information about nutrients available to the animal. Additional biologic and enzymatic methods have been developed for ruminant animals to provide further evaluation of nutrient availability relative to forestomach microbial population, depending on the animal. Although some of these measures may be empirically applied to llamas and alpaca feed evaluations, more information is needed to understand the potential applications of these specialized analytical techniques to feeding llamas and alpacas.
TABLE 12-3
Typical Test Value of Temperate and Subtropical Alfalfa and Grass Hays Harvested at Various Stages of Plant Maturity
Note: All values are based on a dry matter basis.
*Alfalfa growth stages: prebloom = bud to first flower; early bloom = up to of plants in bloom; mid-bloom =
to
of plants in bloom; late bloom = >66% in bloom.
†Grass growth stages: prehead = late vegetative to early boot stage; early head = emergence of seed heads (inflorescence); head = further emergence of seed heads, seeds become well formed; posthead = seeds fully matured and released.
‡Summary analysis from orchardgrass, reed canarygrass, smooth bromegrass, and tall fescue.
§Summary analysis from Bahiagrass, Pangola, and Bermudagrass at 2–3 (prehead), 4–6 (early head), 6–8 (head) and 10 (posthead) weeks of growth.
Adapted from Van Soest PJ: Nutritional ecology of the ruminant, ed 2, Ithaca, NY, 1994, Cornell University Press; and National Research Council, Subcommittee on Feed Composition, Committee on Animal Nutrition: United States-Canadian Tables of Feed Composition: Nutritional Data for United States and Canadian Feeds, rev ed 3, Washington, DC, 1982, National Academy Press.
Although wet chemistry analysis is considered the “gold standard” for feed testing, simpler and less expensive methods with shorter turnaround time are also available. Near-infrared reflectance (NIR) spectroscopy is a readily available testing method.1,2 With NIR analysis, ground feed samples are exposed to infrared light, molecular bonds either absorb or reflect this light, and the reflectance pattern is measured and compared with a standard. Critical to the accuracy of NIR testing is laboratory quality control standardization for feed types. In general, NIR analysis has high accuracy in measuring CP and fiber fractions compared with wet chemistry but is less accurate in measuring feed mineral content.2,3 Many certified feed laboratories are capable of completing wet chemistry, NIR analyses, or both. These laboratories often offer lower-cost mixed methodology testing packages, in which mineral content is determined by wet chemistry and all other parameters by NIR.
Crude Protein
CP content varies significantly across feeds, but within forage species, higher protein is usually associated with higher quality (see Table 12-2). As forages mature, CP content is diluted by increasing fiber content. Forage fertilization practices may alter this relationship, which suggests that CP should not be used as the sole quality criterion without evaluating fiber content.
Other chemical and biologic methods have been used to further characterize dietary protein into fractions potentially utilized by forestomach microbial populations or ruminant animal host (Figure 12-1).4 The host animal can only use dietary nitrogen in the form of amino acids, which are derived from bacterial cells and dietary protein not microbially degraded in the forestomach. In contrast, forestomach microbial populations can utilize nearly all forms of dietary nitrogen, including NPN. Some of these protein fractionation methods are directly applicable to SAC diets, whereas others will require more species-specific determinations.
Soluble Protein.
Protein solubility is defined as the amount of feed nitrogen that solubilizes in a buffered solution, which mimics rumen fluid and is typically expressed as a percent of CP. Soluble protein provides readily available nitrogen to forestomach microbial populations in support of microbial protein synthesis. Forestomach fiber fermenting microbes have an exclusive requirement for ammonia as their nitrogen source.5 Given the high-fiber diets consumed by llamas and alpacas, dietary soluble protein content would be recommended to range between 30% and 40% of dietary CP. Excessive dietary soluble protein would be reflected by elevated blood urea concentrations, which potentially could be recycled to the forestomach via saliva. Llamas have a lower rate of urea turnover and kidney urea excretion rate compared with other ruminants.6 In addition to recycling more urea to bacteria, llamas have been shown to have greater urease activity, the enzyme needed to metabolize urea, compared with other ruminants.6 These differences allow the llama to recycle more urea to the forestomach for use by bacteria in support of microbial protein production.
Degradable Protein.
Degradable protein is defined as the amount of dietary nitrogen or protein equivalent amenable to forestomach microbial degradation in support of microbial protein synthesis. Degradable protein is quantified as a percent of dietary CP or DM. In contrast, undegradable protein is defined as the dietary protein not amendable to microbial degradation. The sum of degradable and undegradable protein as a percent of CP equals 100%. All soluble protein is defined as degradable, but not all degradable protein is soluble. Together, soluble and insoluble degradable proteins support forestomach microbial growth. Likewise, all unavailable protein is undegraded protein, but all undegraded protein is not unavailable (see Figure 12-1). Undegraded dietary protein can provide amino acids to the host animal if the protein is amenable to hydrolytic digestion and absorption in the small intestine.
Protein degradability is measured by exposing the feed protein source to mixed microbial populations in a flask (in vitro) or within dacron bags placed within the rumen (in situ), and undigested residue protein content is measured at defined periods. Dietary protein degradability is a function of chemical and physical properties of the protein itself but is mitigated by forestomach retention time. Retention time is controlled by animal body size, level of intake, and dietary composition (i.e., fiber content and particle size); thus, the degradability properties of a protein are not static. Although forestomach microbial populations are similar between ruminants and SACs, reported feed ingredient degradability values for ruminant animals are not directly applicable to SACs. An observed DM and cell wall digestibility advantage for alpacas compared with cattle was determined at various periods using in situ methods but not in vitro methods in feeding a canary grass species.7 Forestomach retention time is longer in SACs compared with ruminants, which potentially results in a greater extent of protein degradation. Current degradable protein estimates for various feedstuffs might provide a starting point for SAC diets, although increasing the ruminant-derived value by 5% to 10% might be a reasonable estimate for SAC applications.
Fiber
Total cell wall content or structural carbohydrate content, both essentially the same concept, was traditionally measured by the crude fiber procedure. During the acid and alkali digestion of the crude fiber procedure, much of the hemicellulose and lignin is solubilized, thus underestimated total cell wall.8 Although still used as a standard measure of fiber on feed analysis tags, crude fiber does not adequately define the total cell wall fraction and overestimates energy values for forages in comparison with concentrates.
The Van Soest detergent feed analysis system uses sequential neutral and acid detergent digestions to separate feed samples into digestible and indigestible components relative to their cell wall association with the plant.8 Neutral detergent fiber (NDF) is the insoluble residue following neutral detergent digestion. This residue better represents the total cell wall components of a feed sample and comprises primarily hemicellulose, cellulose, and lignin. As the plant’s cell walls thicken with advancing plant maturity, NDF content will increase (see Table 12-2). Feed NDF content impacts dry matter intake (DMI) and rumination activity in ruminant animals as well as in SACs. Rumination activity will increase and DM will decrease with increased feed NDF content. Within a given feed, NDF is a reasonable measure of feed quality and plant maturity.
NDF accounts for the greatest proportion of llama and alpaca diets, similar to ruminant diets. However, all NDF in a diet is not equally fermentable by forestomach microbial populations. Similar to methods used to determine protein degradability, microbial fermentability of feed NDF may be determined using in vitro or in vivo methods. Comparative feeding trials have shown llamas and alpacas to be more efficient in fermenting lower-quality (high NDF) forages compared with other ruminants because of the greater forestomach retention time in llamas and alpacas.9,10 However, not all studies have shown this competitive advantage, possibly because of differences in the quality of forage.11
An important component of plant cell wall structure is the polyphenolic compound lignin. Lignin acts as a cementing structure binding the outer and inner plant cell wall structure to provide rigidity. The lignin compound itself is essentially inert to digestion or fermentation. Consequently, lignin acts to reduce digestibility of associated cellulose and hemicellulose compounds in the cell wall. Plant cell wall lignification increases with maturity and is influenced by interactions between plant species and environmental conditions.8 Generally, cell wall lignification increases with higher ambient temperatures. Lignin content of feed samples may be determined using a sulfuric acid digestion (Klason procedure) of the acid detergent residue.
Energy
Energy content is often used to compare feeds and evaluate quality. Feed energy content is not directly measured like other nutrients are but derived through mathematical regression equations. Traditionally, ADF, alone or in combination with CP, was used to predict energy value of various feeds. Newer National Research Council (NRC) models predict feed energy using a summation equation that accounts for differences in feed component digestibility based on lignin content.12 Most laboratories report TDN and net energy (NE) feed energy values based on cattle equations, although laboratories may use different prediction equations. TDNs are reported as a percent of feed DM, whereas digestible energy (DE), metabolizable energy (ME), or NE are reported as megacalories per unit weight (Mcal/lb [Mcal/kg]). Forage TDN values typically range from 40% to 65%, depending on forage type and maturity (see Table 12-2). Concentrate feeds will have higher TDN values (65% to 90%), whereas fats will have greater than 100% TDN content as a result of their higher energy density compared with carbohydrates. One kilogram or one pound (lb) of TDN is equivalent to 4.4 or 2.0 Mcal digestible energy, respectively. Multiplying percent TDN times 0.02 converts TDN to DE in Mcal/lb (50% TDN ×0.02 = 1.0 Mcal/lb DE).
Nonfiber Carbohydrate Fractions
A large portion of feed carbohydrates are not directly measured (see Table 12-1). The neutral detergent soluble carbohydrate fraction of feed is termed nonfiber carbohydrates (NFCs). This fraction is not directly measured, but determined by difference. With all values on a DM basis, NFC is the remaining portion of total feed content after subtracting CP, NDF, fat, and ash content (e.g., NFC = 100 − (CP + NDF + Fat + Ash). Inherently, all laboratory errors associated with other feed fractions will be compiled into the NFC fraction. Although susceptible to error, NFC represents a highly available portion of a feed and, as such, positively reflects on evaluation of feed quality. Plant NFC content will reflect maturity stage and growing conditions, primarily daylight to support photosynthesis. In grasses, NFC content will range between 14% and 24% DM, whereas legumes will range between 27% and 40% with the lower values associated with more mature forages.
Feed NFC does not represent a single compound but is a compilation of many different compounds. Organic acids, sugars, and starch are readily digested compounds within the NFC fraction. Some feed analysis laboratories now offer enzymatic methods to individually determine feed sugar or starch content. Together, feed sugar and starch content define a subset of NFC termed nonstructural carbohydrates (NSCs). Sugar, starch, or total NSC content of a feed, especially a grain or concentrate feed, is of interest, as these carbohydrates are rapidly and readily fermented by forestomach microbes. A product of microbial NSC fermentation is lactic acid, which will reduce forestomach pH if allowed to accumulate. With their greater fermentation capacity, SACs are potentially prone to forestomach acidosis (see Digestive Diseases, Chapter 40) when consuming diets high in NSC content.
In addition to digestible carbohydrates, NFC contains one or more complex soluble fiber compounds such as pectic substances, fructosans, β-glucans, and galactans. Although these fiber compounds are not amenable to enzymatic digestion by the host animal, they are readily fermented by forestomach microbial populations and support microbial growth. The high NFC content associated with legume forages is a result of its pectin content. Grass forages, especially rapidly growing cool season grass species, contain significant amounts of fructosan compounds as the predominant NFC form. Similar to sugars and starch, fructosan compounds are readily fermentable and can generate lactic acid, thus potentially contributing to forestomach pH load. In contrast, pectic substances and β-glucans are fermentable but do not generate lactic acid; however, their fermentation is pH sensitive with reduced fermentation under acidic conditions.
Ether Extract
Ether extract is the traditional chemical method where all lipid soluble compounds are extracted by being dissolved in ether (see Table 12-1). Following extraction, the ether is evaporated off, leaving the dissolved feed sample residue termed crude fat. This fraction accounts for nearly all lipid-soluble compounds, including triglycerides (triacylglycerol), fatty acids, pigments, sterols, oils, alcohols, and organic acids. Fatty acids and fat-soluble vitamins are essential nutrients contained within this feed fraction. Additionally, triglycerides may contribute to energy needs of the animal but exert negative effects on forestomach microbial populations (refer to Energy Sources − Fat, Chapter 9). Forages typically contain between 1.5% and 4% fat (DM basis), but much of this fat is in the form of galactolipids, pigments, and organic acids. Supplement feeds containing vegetable oils can range in fat content from 3% to over 20% (DM basis), depending on the amount of included fat source.
Minerals
Most feed laboratories offer a selection of mineral panels as part of their feed analysis packages. Most often, chloride, sulfur, molybdenum, and selenium are not included in most routine packages but may be requested. Feed selenium determination is an expensive method and should be requested only when the selenium status of a geographic region is known to be variable or high. Molybdenum and sulfur should be routinely requested, as they influence copper availability. Chloride is not routinely indicated in evaluating SAC diets, although special situations in which cation–anion balance of the diet is of interest may exist. Forage mineral content will vary by forage type and geographic region; thus, contribution of dietary mineral from forage spans the spectrum from deficient to potentially toxic (Table 12-4). Supplement mineral content will depend on constituent ingredients and additional mineral sources. Supplement mineral composition should be complementary to forage mineral content in meeting animal mineral requirements.
Dietary and Feeding Management Assessment
The saying “Three diets exist on the farm: formulated, delivered, and consumed diets” is often used on dairy farms in association with nutritional evaluations. This statement is equally applicable to llama and alpaca feeding programs. The management challenge for the feeding program is to maintain consistency in nutrient content across all three diets, whereas the goal of nutritional investigations is to determine how these diets may differ from each other. The evaluation process should be tailored to the feeding system in place and interpreted relative to animal performance expectations.
Dietary Evaluation
The principal focus of dietary evaluation is to compare provided essential nutrient amounts relative to defined animal requirements in assessing nutrient delivery adequacy. This is accomplished most easily with the use of a computer software program or spreadsheet to complete the necessary calculations.13 Assessment accuracy is dependent on the quality of information used, namely, precision of feed ingredient intake amounts and nutrient composition. A potential difference between formulated or “intended” diet and the actual delivered diet available for consumption is in the distribution of feed ingredient quantities provided. Most notorious in separating nutrient delivery between intended and delivered diet is supplement quantity. Expected supplement intake is often defined as an amount per 100 lb (45 kg) body weight, yet feeding practices often do not account for individual weight differences within a group unless individual feeding is practiced. Thus, at best, an averaged supplement intake is determined for a group. Additionally, supplements are more often measured by volume (i.e., cups, scoops, and cans) rather than by weight. Supplement components vary in inherent density (weight per unit volume), so volume measures are not appropriate in partitioning feed amounts. Owners and veterinarians have demonstrated an inability to accurately assess supplement weight without direct measurement.14 Direct measurement of supplement amount provided to a feeding group is essential to assessing the difference between intended and delivered diets.
Assessing adequacy differences between intended or delivered diet and the consumed diet is more challenging because of inherent selective feeding behaviors of llamas and alpacas. The forage component of llama and alpaca diets is often provided as free choice in the form of pasture or hay. Actual intake may be lower or exceed intended intake amounts because of forage quality effects on intake. Pasture intake is further influenced by stocking density relative to forage availability. Similarly, hay intake is influenced by feeder space relative to animal numbers and wastage. Averaged forage intake is estimated by the amount of forage provided minus wastage and amount remaining after a defined feeding period and is then divided by animal numbers. Depending on the feeding group, animal numbers may need to be adjusted for differences in age and body size to account for differential intake capacity. Remaining forage in pasture is estimated by measuring plant height with a pasture ruler or rising plate meter and calculating forage DM per acre based on forage type.15 Hay fed on the ground may have more than 30% wastage because of trampling and spoilage. Hay wastage when fed from feeder racks or hanging bags is much less (5% to 10%) and is usually the result of dropped or tossed hay. Assessing forage nutrient intake is further complicated by the selective feeding capability of llamas and alpacas. Leaves may be preferentially consumed from legume forages leaving the stems; thus, a very different nutrient composition diet is consumed from what is delivered. Refused feed may be collected and analyzed for nutrient content to estimate consumed nutrients. With these difficulties in accurately characterizing the consumed diet, use of blood analytes to assess nutritional status (see Blood Metabolite Analysis section below and in Chapter 41) in conjunction with dietary evaluation may provide diagnostic advantages.
Dietary evaluation must go beyond a singular focus on essential nutrient composition relative to requirements. A number of recognized associative effects exist between dietary nutrients and ingredients for ruminant animals, which would be applicable to llamas and alpacas. Many of these apply to ratios between various mineral components in recognition of antagonistic actions on availability. Additional parameters may be applied to characterize dietary intake capacity and ingredient composition as well as criteria used to describe dietary components to support microbial population activity (Table 12-5). These dietary parameters are solely based on ruminant information and require further refinement in being applied to llama and alpaca diets.
Feeding System Evaluation
For sheep, linear feed bunk space per animal for limit feeding ranges between 16 and 20 inches (41 and 51 cm ).16 Longer feed bunk space has been recommended for sheep (24 inches [60 cm]) and goats (12 inches [30 cm]).17 Linear feed bunk space per cow is suggested to be 1.15 times the chest width, or 1.25 times chest width for pregnant cows, which defines the minimal amount of space required.18 Consuming feed from both sides of a feed bunk does not double the linear feed bunk space as animals generally are hesitant to eat nose-to-nose. To utilize both sides of a feed bunk, the bunk width should be sufficient to minimize head-to-head contact. Recommended feed bunk space for llamas and alpacas would range between 16 and 20 inches (41 and 51 cm) and 14 and 18 inches (35 and 45 cm), respectively, for limit-feeding of supplement. For continuous feeding of forage in a feed bunk, 6 to 8 inches (15 to 20 cm) per animal may be sufficient.
In pasture feeding systems, the key issue to evaluate is stocking density or rate. Stocking rate is the number of animals allowed to graze for defined amounts of time for a given pasture size. Critical to stocking rate is not the size of the pasture but the type and amount of vegetation growing in the pasture. More detailed information describing stocking rate calculations and grazing management can be found in other texts.15 With good-quality temperate region pasture, typical recommended stocking rate is 2.5 animal units per hectare (1 animal unit per acre). A standard animal unit is defined as a 1000 lb (454 kg) steer, which is equivalent to 5 sheep or 0.7 dairy cows. Using average body weights for llamas and alpacas, 1 animal unit would be approximately equal to 3 to 4 adult llamas and 6 to 7 adult alpacas. In many situations, overstocking is of concern as a result of poor-quality or inadequate forage resources to adequately support animal numbers. Overstocking not only requires additional forage feeding but adversely affects pasture quality and regrowth potential.
Water accessibility is essential to animal health and performance. Recommendations for sheep suggest 15 to 25 head per 12 inches (30 cm) of water tank length or 40 to 50 head per watering bowl.16 These recommendations are less than those suggested for dairy cows, in which case 24 inches (60 cm) of accessible water trough perimeter supports 15 to 20 cows.19 The doubling of accessible watering space accounts for the greater water needs of lactating dairy cows and would be appropriate for lactating camelids or for those animals housed in hotter environments where water needs are greater. In general, a watering unit should accommodate 5% to 7% of a group drinking at the same time. In hotter environments, the capacity may be increased to accommodate 15% of the group at one time. Most animal groups should have more than one watering unit so that a dominant animal does not prevent others from drinking.
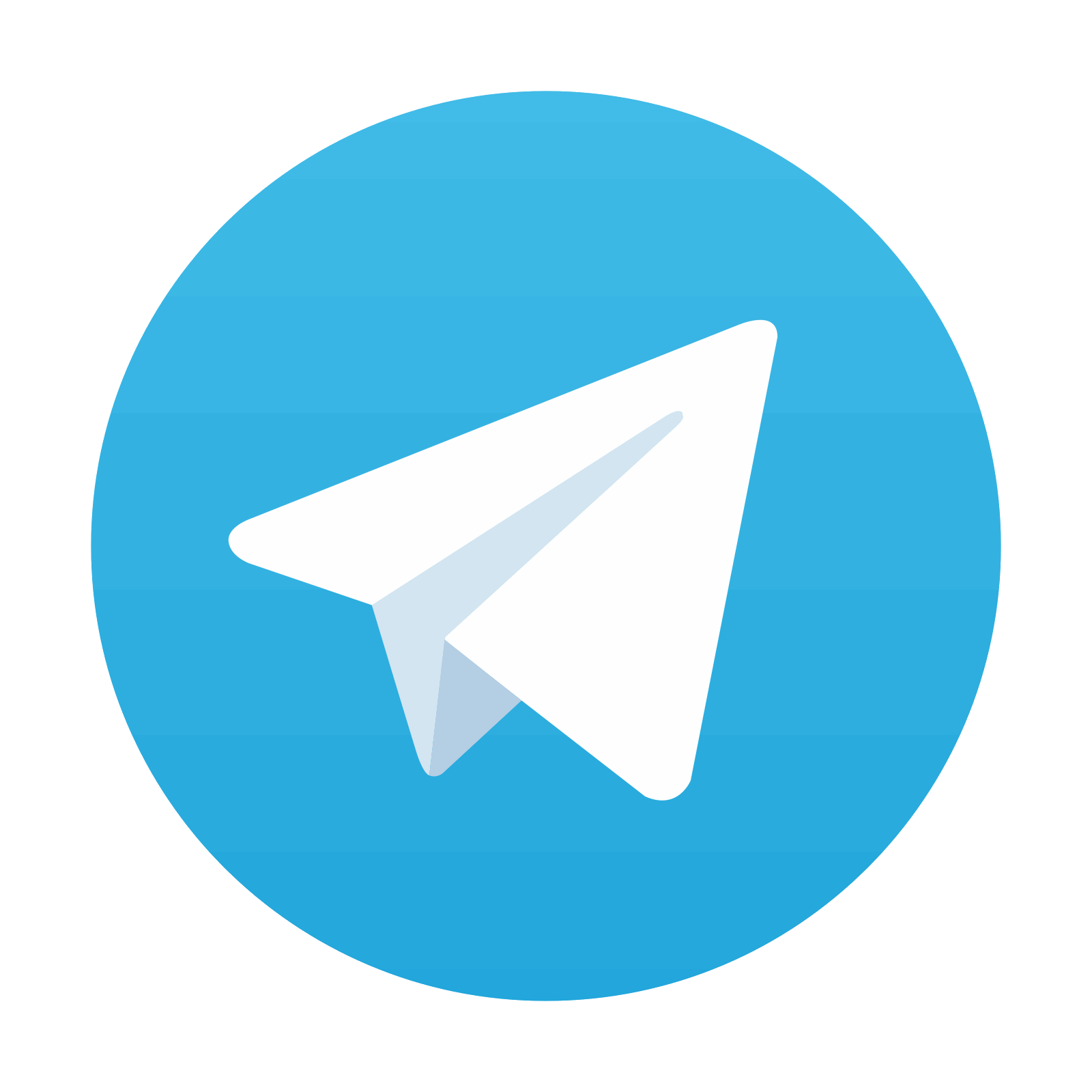
Stay updated, free articles. Join our Telegram channel

Full access? Get Clinical Tree
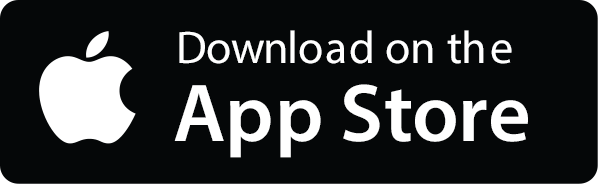
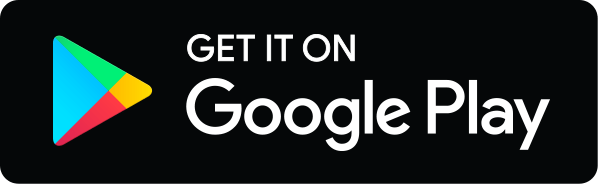