Chapter 18 NEW ASSISTED REPRODUCTIVE TECHNIQUES APPLIED FOR THE HORSE INDUSTRY
The development of assisted reproductive technologies (ARTs) in the horse dates back to the late nineteenth century with the establishment of the first equine pregnancies obtained by artificial insemination.1 Since then, ARTs have helped to produce offspring from valuable mares that cannot produce embryos and are considered infertile. Besides artificial insemination, which is discussed in detail elsewhere, embryo transfer has been the most common ART in mares. However, there are a number of mares that cannot produce embryos because of various pathological conditions of the reproductive tract. Therefore, other embryo production techniques using both in vivo and in vitro procedures have been developed. These techniques include (1) oocyte transfer (OT), (2) intracytoplasmic sperm injection (ICSI), (3) in vitro fertilization (IVF), and (4) nuclear transfer or cloning. All of these techniques are intended to increase the chances of producing offspring from severely subfertile mares or stallions. ARTs involve the endocrine stimulation of the gonads and the in vitro and in vivo manipulation of gametes. In addition, it is not uncommon to use surgical procedures to accomplish some of these techniques.2
The development of techniques such as ultrasound-guided aspiration of follicles,3–6 which allows recovery of in vivo matured oocyte from live mares, has led to some clinical interest in the standardization of IVF, which includes the culture of mature oocytes with capacitated sperm. However, this procedure has not resulted in an efficient method to produce equine offspring. The main reasons for the limited progress of IVF in the horse include the scarce availability of abattoir ovaries, poor in vivo recovery of immature oocytes, the difficulty in developing efficient systems for oocyte maturation in vitro, and problems in defining and achieving sperm capacitation in vitro. The lack of basic scientific information on both of these fields has made IVF an expensive and inefficient technique in the horse.
To date, only two foals have been produced after IVF of oocytes matured in vivo.7 Because of the inefficiency of IVF, researchers and clinicians have opted for different approaches in order to fertilize equine oocytes both in vivo and in vitro. As mentioned previously, these techniques include OT, gamete intrafallopian transfer (GIFT), and ICSI. In addition, several recent reports describe successful cloned horses, some of which are performing the procedure commercially.8 Of the in vitro ARTs, OT has been the most repeatable and affordable in horses. However, recent improvements in the efficiency with the use of ICSI have also increased the interest for the use of these techniques by horse breeders.
OOCYTE COLLECTION AND IN VITRO MATURATION
The first successful maturation of equine oocytes in vitro was achieved by Fulka and Okolski,9 which coincided with the time when research on the technology of in vitro maturation of ruminant oocytes was at its peak. This was in great part enhanced by the unlimited availability of abattoir-collected bovine oocytes and the lack of restrictions to conduct research with such material.2 In contrast, in the case of the horse, availability of abattoir ovaries is scarce and in vivo oocyte recovery rate; with the use of ultrasound-guided follicular aspiration is far from providing enough viable oocytes.
In the equine, the anatomy of oocyte attachment to the follicular wall interferes with the efficiency of oocyte recovery both in vivo and in vitro. In the mare, the follicle has a thecal pad beneath the cumulus cell attachment. These processes are the extension of the granulosa cells into the thecal layer. The position of the pad, the granulosa cell processes that extend into it, and the acid polysaccharide component of the pad seem to act as an anchor for the cumulus attachment.10 In addition, the equine ovary has a much smaller number of antral follicles compared with other species, with the average number of visible follicles in the horse’s ovaries being six per ovary.2,11,12 Several investigators have reported the number of harvested cumulus oocyte complexes (COCs) using different techniques. Okolski et al.13 and Hinricks and DiGiorgio12 reported an average of 1.5 and 2.7 COC per ovary, respectively, when aspirating the follicles. Zhang et al.14 reported a COC recovery of 3.1 COC per ovary when the follicular wall is scraped. Choi et al.15 obtained 4.14 oocytes per ovary when the ovaries were sliced and washed.
In vivo or in vitro follicular aspiration results in an average recovery of 1.5 oocytes per ovary in horses compared with an average of 10 oocytes per ovary in the cow. However, harvest of in vitro recovered cumulus intact oocytes or COCs is greatly increased (50% to 80% of aspirated follicles) if follicles are opened and the granulosa layer is scraped from the follicle using a curette12,16 (Fig. 18-1). Unfortunately, this process significantly increases the time and number of personnel required to collect oocytes from a given number of slaughterhouse ovaries. The difficulties in obtaining large numbers of oocytes represent a major limitation for research on technologies such as IVF, ICSI, embryo culture, and cloning.
Palmer et al.17 were the first to describe a standing in vivo aspiration technique for horses, where the operator held the ovary via rectum and guided a needle through the flank toward the pre-ovulatory follicle. The recovery rate reported in this pioneering work was 63%. The same year, McKinnon et al.18 reported a recovery rate of 71.4% when using a trocar cannula and a larger needle (9.8 mm) to aspirate preovulatory follicles via the flank. A similar procedure was described by Hinrichs and DiGiorgio12 with recovery rates of 73%. To aid in the fixation of the ovary for the flank technique, a colpotomy incision was made in the cranial vagina, enabling the operator to introduce his hand into the peritoneal cavity and to fix the ovary directly against the abdominal wall.11
The first group of researchers to describe the ultrasound-guided transvaginal aspiration (TVA) approach to harvest oocytes in the standing mare was Brück et al.3 Based on a similar approach used in humans and cattle IVF programs, a finger-shaped transducer connected to an ultrasound and equipped with a needle guide that showed the puncture line on the screen was used to aspirate preovulatory follicles (Fig. 18-2). A single lumen needle attached to a 50-ml syringe was used to flush the follicular cavity up to three times with Dulbecco’s PBS. One oocyte was recovered out of four follicles.
Cook et al.5 performed a study comparing single- and double-lumen needles. The 12-gauge double-lumen resulted in the highest recovery rate (84%) of preovulatory follicles compared with the single lumen needle (52%). The double-lumen needle allowed the fluid to drip continuously into the follicle while suction was being applied.
The aspiration of immature follicles increases the number of follicles punctured. Cook et al.19 aspirated oocytes on days 7 to 9 of diestrus when an average of four follicles measuring 10 to 25 mm was detected. A total of 135 diestrus follicles were aspirated and 25 oocytes (18.5%) were recovered,20 indicating that follicle size significantly influenced the COC recovery. More oocytes were collected from small follicles measuring 5 to 15 mm (52%) than from subordinate follicles measuring 20 to 27 mm (22%). The scraping technique of the follicular wall is easier to perform when the follicle diameter is smaller.21,22 Although there are abundant data in the literature concerning the aspiration of immature oocytes, very little is known about the developmental potential of such oocytes for producing horse embryos. Furthermore, comparisons and data interpretation are difficult because of the great variation in procedures between laboratories. For example, Squires22 reported a range between 8% and 31% embryo development, while Bezard et al.21 reported between 22% and 52% and Galli et al.2 reported between 26.7% and 71.1%.
The pregnant mare has also been considered a source of oocytes. Meintjes et al.23 Ginther,24 and Ginther and Bergfelt25 have described the occurrence of follicular waves during early pregnancy in mares. Follicular activity is variable among early pregnant mares, ranging from periodically to sporadically occurring major waves (largest follicle ≥35 mm) in some mares to only minor waves (largest follicle ≤35 mm) in others. Oocyte recovery rates from pregnant mares seem to be higher compared with cycling mares. Goudet et al.26 reported 54% oocyte recovery rates in pregnant mares vs. 47% in cyclic mares. Meintjes et al.23 reported 75.8% oocyte recovery for pregnant mares vs. 42.9% for pre-ovulatory follicles used as control. In a first study by Meintjes et al.,23 eleven aspirations were performed per mares between days 22 and 66 of gestation with a maximum of seven oocytes recovered per aspiration session. In a second study, the same authors27 extended the aspirations until day 150 of gestation. The mean number of aspirations per mare was around 7.6 and the average number of oocytes obtained was 18.9 per mare. They concluded that an average of 2.5 oocytes could be collected every 7 to 10 days from pregnant mares. It was estimated that 19 oocytes could be retrieved from a mare between days 21 and 150 of gestation compared with 12 collected during 130 days in cycling mares without any hormonal treatment. In another study, Cochran et al.28 reported an average of 13 follicles per procedure, and an oocyte recovery rate of 66% for 20 aspirations performed between days 14 and 70 of pregnancy.
Oocyte Maturation
After the oocytes are collected and selected on the basis of cumulus morphology, they are transferred to a maturation medium and allowed to mature in vitro for 24 to 36 hours. Oocyte maturation consists of nuclear and cytoplasmic modifications that prepare the oocyte for fertilization. In vivo, maturation is a process coinciding with follicular development, changing hormone levels, and meiotic progression. Nuclear maturation is referred to as the finalization of the meiotic process with the reduction of DNA content in the oocyte. Resumption of meiosis is marked by extrusion of the first polar body and formation of a metaphase II plate. In most species, the resumption of meiosis is mediated by LH-receptor interaction in the cumulus cell membrane, mediating the increase of the MPF in the ooplasm.29,30
A detailed description of meiotic changes was reported by Grondahl et al.,31 who used transmission electron microscopy to visualize the process. According to the stage of nuclear maturation observed using light microscopy, oocytes are classified as follows:
The occurrence of meiotic maturation is accompanied, and probably regulated, by changes in the phosphorylation patterns of various cellular proteins. One such protein is the MPF reported by Masui and Makert,32 which is known to be a universal cell cycle regulator of both mitosis and meiosis.33 Active MPF induces chromosome condensation, nuclear envelope breakdown, and cytoplasmic reorganization with entry into M-phase of either mitotic or meiotic cell cycle.34–36 During oocyte meiotic maturation of mammalian species, MPF activity is very low in the germinal vesicle stage and peaks at metaphase I and II stages (mouse: Hashimoto and Kishimoto,37 Choi et al.38; rabbit: Naito and Toyoda39; goat: Jelinkova et al.40; pig: Dedieu et al.41; bovine: Wu et al.42) (Fig. 18-3). Other kinases are also involved in the regulation of meiotic events, such as mitogen-activated protein kinase (MAPK).103
Cytoplasmic maturation is characterized by several changes in the shape and localization of the organelles. Grøndahl et al.31 described a breakdown of the intermediate junctions between the cumulus cell projections and the oolemma with an enlargement of the peri-vitelinic space. In addition, they described the formation and arrangement of a large number of cortical granules immediately beneath the cytoplasmic membrane and the structural change of the mitochondria to a round shape. The migration of the cortical granules is believed to be an important step in cytoplasmic maturation43 and can be used to assess general oocyte maturity.23,44 The redistribution of the cortical granules provides the ovum the capacity to initiate the block of polyspermy and to induce sperm nuclear decondensation.43
Factors that may affect cytoplasmic maturation include presence of cumulus cells, addition of gonadotropins to the maturation media, and time of culture. The structure of the oocyte and its associated granulosa cells is collectively called the cumulus oocyte complex (COC). The presence of granulosa cells during in vitro maturation has proven to be beneficial in humans,45 rabbits, and cows.46 The inner layer of the COC is called the corona radiata and is connected with the oocyte membrane, permitting the transfer of molecules from the outer granulosa cells to the oocyte.47 The association between germinal cells and somatic granulosa cells regulates the level of synthesis of specific proteins and the pattern of protein phosphorylation in growing oocytes, thus directly regulating their metabolism.
The in vitro maturation of equine oocytes reviewed by Galli et al.2 ranges from 20% to 85%, and comparisons between in vitro maturation procedures in horses are difficult because each study has a different protocol.
An interesting aspect, peculiar to the horse oocyte, is that oocytes with expanded cumulus mature have normal developmental competence. In other species such as ruminants and pigs, the presence of expanded cumulus is linked to the collection of oocytes from atretic follicles, and these oocytes are generally discarded immediately because of their extremely low developmental capacity.48 Hinrichs et al.,49–51 in a series of studies demonstrated that oocytes with expanded cumulus were more capable of complete maturation than oocytes with compact cumulus.
Another aspect is that the maturation rate will be directly linked with the quality of the ovaries used and especially with the time between slaughter and collection of the oocytes. Better maturation rates are obtained when oocytes are transferred directly into the maturation media after aspiration. Delaying this process will result in reduced maturation rates.52 In our laboratory, ovaries must be transported 500 km and the total time between slaughter and beginning of maturation is about 8 to 9 hours. It is important to mention that it is not always clear from the literature how the maturation rate of equine oocytes is calculated and whether degenerated oocytes are taken into account. In our laboratory, degenerating oocytes identified after in vitro maturation are taken into account to establish our maturational rates.
The majority of studies in equine oocyte IVM use TCM-199 as a culture medium with the addition of serum, follicular fluid, or hormones (LH, FSH, and estradiol 17β).50,53–56 However, the source and levels of LH and FSH have not been optimized. LH of ovine,53 bovine,53,57,58 equine origin,20,23 and equine pituitary extract (EPE)59 have been used, but none of these has increased the efficiency of conventional equine IVF.
OOCYTE TRANSFER IN MARES
The OT procedure involves several steps where the recipient reproductive tract will provide a healthy environment for sperm survival and transport, oocyte fertilization, and embryo development. The first successful OT in the horse was performed in 1988 by McKinnon et al.,60 and 10 years later the first commercial program was established at Colorado State University.61 Since then, more than 200 pregnancies from very valuable mares have been obtained.62
Results from this program have demonstrated that pregnancies can be obtained consistently from older, subfertile mares using OT. In spite of a low efficiency (30% to 40% pregnancy per transferred oocyte), at least one pregnancy from 80% of the mares has been achieved in the last 11 years.62 For mares <15 years, higher pregnancy rates (60% to 70%) per OT are reported.63 OT is usually performed in valuable mares from which embryos cannot be collected because of poor oocyte viability, high incidence of ovulatory failure in mares of advanced age (>18 years), oviductal pathology, and advanced uterine or cervical pathology. Most OT candidates are older mares whose expected embryo recovery rate is around 30%. Carnevale et al.62 reported that in mares with histories of repeated ovulatory dysfunction, if aspirations are done before the luteinization and/or echogenic debris on follicular fluid is observed, the retrieved oocyte quality is better, thus increasing the chances of pregnancy.
OOCYTE TRANSFER TECHNIQUE
Preparation of the Donor
Difficulties in the retrieval of oocytes from immature follicles, as well as in vivo and in vitro maturation techniques, have forced investigators and clinicians to collect oocytes from pre-ovulatory follicles. Ovulation of donor mares is induced with a combination of hCG (1500 to 2000 UI IV) and deslorelin acetate (1 mg IM) when a pre-ovulatory follicle is observed. The combination of these two drugs appears to induce a better follicle response and better oocyte quality in old mares.61 However, it is important to remember that response to ovulation-inducing drugs is more variable in old mares, and sometimes the oocyte aspiration must be performed before the expected ovulation time. This is particularly evident in mares with a history of ovulatory problems.
Oocyte Collection and Culture
Several approaches have been used for follicular aspiration in the live mare. These include flank laparotomy, transcutaneous flank puncture, or TVA. The method of choice for oocyte retrieval is TVA using a linear or a sector ultrasound (see Fig. 18-1). TVA has the advantage of being a non-surgical procedure, allowing repeated aspiration attempts on consecutive cycles. Donor mares are sedated with a combination of butorphanol (0.01 mg/kg) and xylazine (0.33 mg/kg). In addition, 0.04 mg/kg of propantheline bromide can also be used to promote rectum relaxation. During the TVA, the ultrasound transducer is placed in a plastic casing containing a needle guide and is inserted into the vagina. The ovary is positioned over the ultrasound transducer by rectal manipulation, and the follicle from which the oocyte will be collected is visualized. A double-lumen needle is advanced and punctures the follicular wall. The fluid is aspirated by gentle suction (150 mmHg) and the follicular cavity is rinsed with 50 to 100 ml of Dulbecco’s phosphate buffer saline supplemented with 10 IU/ml of heparin. After the aspiration process is finished, the recovered fluid is transferred to a Petri dish and searched for the COC. Although the presence of the large cumulus cell mass makes the COC easy to visualize in the blood-tinged fluid, the large mass makes it more difficult to manipulate the oocyte.
The interval between ovulation induction and OT must be between 36 and 44 hours. Most oocytes collected at 24 hours after hCG injection are in metaphase I and require a supplementary culture time to complete maturation.62 After collection and classification, recovered oocytes are transferred to tissue culture medium TCM-199 supplemented with 10% fetal calf serum and 0.2 mM of pyruvate, and then incubated at 38.5° to 39°C in an atmosphere containing 5% to 6% CO2 in air for 12 to 18 hours before transfer. Most oocytes are expected to recover at 36 hours after hCG administration are in metaphase II and can be transferred immediately into a recipient’s oviduct. In 2002 Carnevale et al.64 reported similar oocyte collection rates and embryo development rates for oocytes collected at 24 or 36 hours after hCG administration and transferred directly. Furthermore, no differences were detected when oocytes were aspirated 24 hours after hCG injection and immediately transferred or incubated in vitro during the next 12 to 16 hours.64 These results eliminate the necessity of an expensive CO2 incubator, making the procedure more practical and possible to be performed under clinical conditions.
Preparation of Recipient Mares
During OT, sperm transport, capacitation, fertilization, and embryo development occur within the recipient’s reproductive tract; therefore, it is very important that a selection of good quality recipient mares be used in an OT program. Young mares (3 to 10 years) are selected after a complete clinical and reproductive examination. During the reproductive examination, it is important to evaluate the length of the broad ligaments to determine if the ovaries can be easily exposed during OT. Oocyte recipients can be cyclic or non-cyclic mares. Use of cyclic mares as oocyte recipients involves estrous cycle synchronization of donor and recipient mares and the removal of the recipient’s oocytes to be sure that the pregnancy will result from fertilization of the donor oocyte. Recipient mares receive 2000 IU of hCG at the same time as the donors, and the recipient’s oocyte is collected approximately 24 hours after hCG administration. Only recipient mares from which an oocyte is collected are used as oocyte recipients. Use of non-cyclic recipients eliminates the need to synchronize donors and recipients and eliminates the need to retrieve the pre-ovulatory oocytes from the recipients before transfers. Non-cyclic recipients receive 3 mg of estradiol benzoate daily for approximately 2 to 5 days before transfer. Following the estradiol treatment 200 mg per day of injectable progesterone in oil or 0.044 mg/kg of oral progestagen (Altrenogest), supplementation is required until OT. Regardless of whether the mares are cycling or not, progesterone supplementation must be continued for pregnancy maintenance until day 110 to 120. Although a corpus luteum forms after aspiration of the preovulatory follicle,65 progesterone secretion can be delayed or reduced in cyclic mares. In non-cyclic mares, the absence of corpus luteum obviously requires progesterone supplementation.
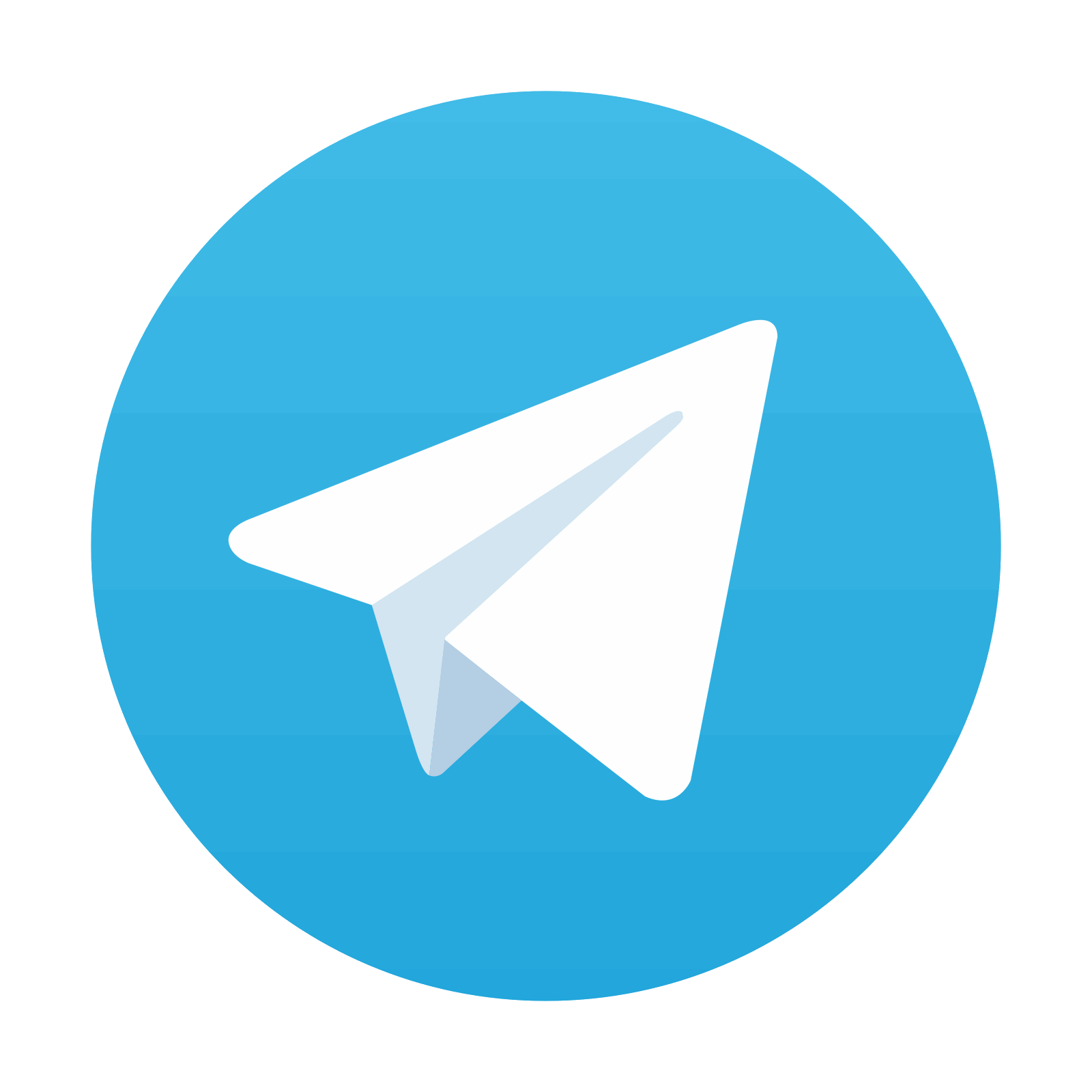
Stay updated, free articles. Join our Telegram channel

Full access? Get Clinical Tree
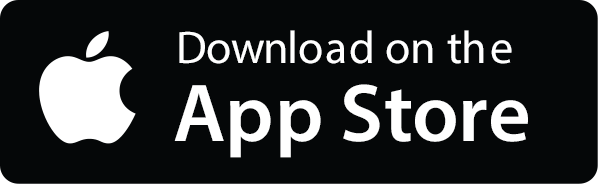
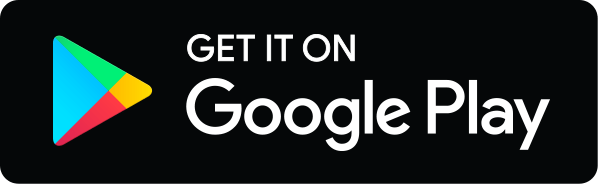