Chapter 2 REPRODUCTIVE ENDOCRINOLOGY OF THE STALLION
It is well established in many mammalian species, including the stallion, that normal spermatogenesis depends on a functional hypothalamic-pituitary-testicular (HPT) axis, which involves secretion of pituitary hormones into the peripheral circulation that act at the level of the testis. Subsequently, testicular hormones are secreted back into the blood and act as classic feedback regulators on hypothalamic and pituitary hormones.1,2 Historically, the major hormones involved have been gonadotropin-releasing hormone (GnRH) produced and secreted from the hypothalamus; luteinizing hormone (LH) and follicle-stimulating hormone (FSH), produced and secreted from the anterior pituitary; androgens and estrogens produced and secreted from the testicular Leydig cells; and estrogens and inhibin produced and secreted from the testicular Sertoli cells. However, recent evidence suggests that a paracrine–autocrine system at the level of the testes is also involved in normal reproductive function.3–8 The specific nature and relative contribution of the various factors involved in the endocrine–paracrine–autocrine control of reproductive function in normal stallions are not well defined, nor have they been elucidated in the idiopathic subfertile or infertile stallion.9 My laboratory has been engaged in characterizing the HPT in fertile, subfertile (idiopathic oligospermia), and infertile (idiopathic azoospermia) stallions.10–24 Our studies have not only identified endocrine factors and mechanisms important for normal reproductive function, but also demonstrated specific hormonal alterations in pituitary and testicular function among fertile, subfertile, and infertile stallions. Although these observations suggest both a pituitary and testicular dysfunction of an endocrine nature, until recently it has not been apparent which reproductive organ has the primary defect or whether paracrine–autocrine factors play a role in reproductive function. Studies in my laboratory suggest that the primary defect is at the level of the testes but that the nature of the dysfunction is not related to a change in LH receptor–binding kinetics.20,21,23 Associated with receptor activity and signal transduction is the paracrine–autocrine modulation of these endocrine events. Paracrine–autocrine systems in the testes have been well characterized in other species and appear to be an important component of normal testicular function, including regulation of steroidogenesis and spermatogenesis.3–8 This chapter summarizes current information regarding the nature of the multiple endocrine–paracrine–autocrine systems that may be necessary for normal reproductive function in the stallion and their impact on stallion fertility.
THE PINEAL GLAND AND THE HYPOTHALAMIC–PITUITARY–TESTICULAR AXIS
Sharp and Cleaver25 have written an excellent review chapter on the pineal gland and melatonin, a hormone that regulates GnRH production and secretion in the stallion. In addition, an outstanding review of the physiology and endocrinology of the stallion by Amann2 describes the involvement and interaction of the classic hormones that make up the HPT axis to control spermatogenesis in this species. Findings in recent studies have added to our knowledge of the dynamics of the pineal gland–HPT axis, including additional hormones that may be involved in controlling testicular function in the stallion.26 The interactions of the pineal gland, brain opioids, thyroid gland, and the HPT axis of the stallion is presented in Fig. 2-1.
Pineal Gland and Melatonin
The classic reproductive endocrine system in a seasonal breeder, such as the stallion, starts at the level of the pineal gland and the secretion of melatonin.25 Melatonin is controlled by light signals received by the retina and transmitted via the optic nerve to the pineal gland.25 The final action of this indoleamine at the level of the central nervous system is a modulation of GnRH secretion, but it does not act directly on GnRH neurons; rather, its action involves a complex neural circuit of interneurons that includes at least dopaminergic, serotoninergic, and aminoacidergic neurons.27 In addition, this network appears to undergo morphological changes between seasons.27 With decreasing light, the pineal gland produces more melatonin, which inhibits the release of GnRH, resulting in a decrease in the gonadotropins, steroids, and testicular activity.25 The endocrine events leading to maximum reproductive capacity in the stallion are initiated when day lengths are still short, albeit increasing.28 Increasing the period of light suppresses melatonin synthesis by modulating the activity of its biosynthetic enzymes29; in turn, GnRH, LH, and FSH are released and testicular activity resumes.30 Cleaver and co-authors31 reported that exposure of mares to constant light resulted in a marked decrease in circulating melatonin concentrations and an increase in hypothalamic GnRH content. It has been demonstrated that exogenous melatonin decreases plasma testosterone concentrations in stallions.32 Elevated melatonin levels have been found in men with primary hypogonadism or infertility with oligospermia or azoospermia.33,34 Acute suppression of LH levels has been reported in healthy men as well.35 Inhibition of sperm motility in normal semen has also been observed following melatonin administration in vitro and in vivo.36,37
HPT Axis
As observed in other species, under appropriate stimuli, the hypothalamus of the stallion releases GnRH in a pulsatile fashion, which, in turn, stimulates the production and pulsatile release of the gonadotropins.38,39 LH stimulates the production and release of both testosterone (T) and estrogens from the Leydig cells in the adult stallion.19 In contrast and as demonstrated in the stallion and other species, FSH binds to the Sertoli cell to release estrogen, inhibin, activin, androgen-binding protein (ABP), transferrin, insulin-like growth factor-1 (IGF-1) and other factors needed for spermatogenesis.2,40–43 Although it has been reported that prolactin plays a role in testicular function in the boar,44 ram,45 rodent,46,47 and human,47 it is unclear whether it has any function in the stallion testes.19
Testicular proteins and steroid hormones feed back to the hypothalamus and pituitary via the peripheral circulation to modulate the discharge of GnRH, LH, and FSH.1,2 A study by Muyan and co-authors15 clearly demonstrated that estrogens, particularly estradiol-17β (E2), and not the androgens, modulate LH release from stallion pituitary cells. The results demonstrated that estradiol significantly enhanced the GnRH-induced LH release from equine pituitary cells incubated in culture, whereas testosterone and dihydrotestosterone had no effect. Most likely, the major role of testosterone in the stallion is a negative feedback effect on the release of GnRH at the level of the hypothalamus, as has been demonstrated in other male species.48 In terms of estrogen, its function at the hypothalamus in the stallion is still unclear. Although it has been shown to have both negative and positive feedback effects in other species,48,49 its mechanism of action at the hypothalamus in the stallion is yet to be revealed. Thompson and co-authors50 demonstrated that treatment with estradiol decreased circulating FSH levels, increased circulating LH levels, and decreased GnRH-induced FSH secretion in long-term geldings. Thompson and Honey51 further demonstrated a role of estradiol on FSH secretion when they reported that immunization of colts against estradiol increased circulating FSH levels without changing LH levels. These studies do not identify the site of action of estradiol, however. Estradiol could be acting at the hypothalamus to change the amplitude and frequency of GnRH and thus the ratio of LH and FSH secreted from the pituitary as observed in the rat and primate,52,53 or estradiol could be acting at the level of the pituitary to modulate GnRH-induced LH and FSH release as observed in other species, including the horse.15,49,54 Because inhibin inhibits FSH secretion at the level of the pituitary in several mammalian species,55 inhibin may act in conjunction with estrogen or testosterone in the stallion to control FSH secretion. In the stallion, both FSH and inhibin exhibit similar seasonal changes; peripheral FSH and inhibin increase in the spring and decrease in the fall.16,56 Inhibin and activins are members of the transforming growth factor super family of growth and differentiation factors.57 They consist of glycosylated polypeptide dimers involving subunits linked by a disulfide bond. In the case of inhibin, the subunits consist of an alpha (α) subunit linked to a beta (β) subunit, either βA or βB. Activin consists of two β subunits linked by a disulfide bond, either βAβA, βBβB, or βAβB. The subunits have been localized in both the Leydig and Sertoli cells by immunocytochemistry.55–57 Not much is known about the role of activin in the stallion. In other species, activin appears to be stimulated by FSH from Sertoli cells and to act at the level of the pituitary to release FSH.55 Inhibin/activin βA subunit mRNA activity has been demonstrated to be expressed in the endometrial glands of the mare during pregnancy.58 One could surmise that activin is also localized in the testicular somatic cells of the stallion given the fact that activins like inhibins consist of β subunits that have been localized in equine Leydig and Sertoli cells.56 Studies investigating activin activity at the level of the hypothalamus, pituitary gland, and testes in the stallion have not been done.
Prolactin, Thyroid Hormone, and Growth Hormone
Prolactin, thyroid hormone, and growth hormone (GH) have been reported to affect testicular function in other species. Prolactin appears to play a role in inducing transcription of the estrogen receptor.59 It has been shown to affect androgen-sensitive tissues and, together with LH and GH, control synthesis of LH receptors in the testes, activate androgen synthesis, and affect spermatogenesis.44–46 Thyroid hormone is crucial to the onset of adult Leydig cell and Sertoli cell differentiation.60,61 Hypothyroidism during testicular development leads to proliferation of the testicular somatic cells and larger testes.60,61 One critical molecular target of thyroid hormone in the Leydig cell may be the steroidogenic acute regulatory (StAR) protein, which is responsible for cholesterol transport across the outer mitochondrial membrane in Leydig cells.61 Lack of thyroid hormone leads to a decrease in StAR mRNA and protein, causing a decrease in testosterone production.61 In Sertoli cells, thyroid hormone stimulates lactate secretion as well as mRNA expression of Müllerian-inhibiting substance, aromatase, estradiol receptor, and androgen-binding protein.62 Thyroid hormone receptors have been identified in sperm, in developing germ cells, and in Sertoli, Leydig, and peritubular myoid cells.62 GH appears to affect testicular function by modulating gonadal steroid synthesis and gametogenesis.63 It has been demonstrated that GH mediates the release of pituitary LH as well as the synthesis and release of testicular IGF-1.64 GH deficiency is associated with abnormally small testes,65 and in humans can result in reduced or absent sperm motility. GH treatment can increase plasma IGF-1 concentrations and restore sperm motility.66 GH resistance is associated with reduced fertility in men.67 GH may alter gametogenesis by affecting testosterone synthesis, since testosterone is necessary for sperm production and mRNA-encoding GH receptors are present in rat Leydig cells.68 GH increases basal or hCG-stimulated testosterone production in GH-deficient men.69
Although prolactin and thyroid hormone fluctuate with the season in the stallion and mare, respectively,70,71 the role of these hormones on reproductive function in the stallion is yet to be determined. Prolactin did not appear to stimulate testosterone production in equine Leydig cells in culture,19 and the seasonal changes and effects of thyroid hormone have not been investigated in the stallion. In the mare, thyroid function was not associated with infertility.72 GH does not appear to fluctuate with season in the stallion.73 However, treatment with a somatostatin analogue (GH inhibitor) caused a transient decrease in semen motility and hCG-induced testosterone release, suggesting a role for GH in the regulation of testicular function in stallions.73 A direct effect of GH on Leydig cell steroidogenesis in culture was not observed.74
Opioids
In the stallion as well as other species, it appears that seasonal changes in GnRH/LH release are partly regulated by opioids.75 Opioids are secreted from the brain, travel to the hypothalamus, and cause a decrease in GnRH release during the winter months.75 Aurich and co-authors76 showed that treatment with an opioid antagonist naloxone caused an acute LH release in stallions outside of, but not during, the breeding season. The opioidergic regulation of LH release appears to require the presence of the gonads.77
ENDOCRINE AND TESTICULAR CHANGES ASSOCIATED WITH SEASON
Historically, the stallion has been classified as a “long-day breeder” because maximum reproductive capacity is attained during periods of increasing day length. During the spring and summer, there is an increase in testicular size and weight, sperm production and output, libido, and plasma concentrations of LH, FSH, testosterone, E2, inhibin, and prolactin.16,56,71,78–81 The endocrine events involved in initiating testicular recrudescence in the stallion have not been adequately characterized but appear to start with a photoinducible decrease in melatonin from the pineal gland, which, in turn, allows for an increase in hypothalamic GnRH and a subsequent increase in pituitary LH and FSH release into the peripheral circulation, although reports on seasonal or photoinducible changes in FSH release are conflicting.25,81–85 Increasing plasma concentrations of gonadotropins, and perhaps prolactin and thyroid hormone, act on the testicular Leydig and Sertoli cells to increase production of T, E2, and inhibin and spermatogenesis in the stallion. Although the hypothalamic-pituitary-gonadal (HPG) axis of the stallion is stimulated by photoperiod in a similar fashion as the HPG of the mare,86 it is unclear why ~75% of mares shut down completely while stallions have the capacity to continue to breed throughout the non-breeding season, albeit with lower sperm numbers per ejaculate.87 In the stallion, opioidergic neuromodulators affect GnRH and dopaminergic neuromodulators affect prolactin release.88 Major interactions between dopaminergic and opioidergic systems in the regulation of LH and prolactin release do not seem to exist in the male horse.88 The signaling pathway of these neuromodulators could be different between the mare and stallion. In addition, follicular development, oocyte maturation, and ovulation require an intricate network of shifting hormones and local factors whereas the dynamics involved in the process of spermatogenesis require a more subtle input. Perhaps from an evolutionary standpoint, the stallion’s HPT axis is less sensitive to changes in day length in order to ensure maximum breeding efficiency throughout the year.
A comprehensive 2-year study by Clay and Clay85 on the effects of season and photoperiod on endocrine and testicular function in stallions has revealed that the stallion, like other mammalian species, has an endogenous circannual cycle in testicular function that is entrained by an external environmental rhythm or zeitgeber (time-giver), or photoperiod.82 In this study, stallions exposed to 8 hours of light and 16 hours of dark (8:16) for 20 months starting in July continued to display a significant seasonal cycle in testicular size, sperm output, and peripheral hormone levels similar to the control group exposed to naturally occurring changes in day length. Thus, stallions maintained on continuous short days have the capacity to recrudesce despite the lack of exposure to a stimulatory photoperiod. On the other hand, testicular recrudescence was accelerated earlier in the season compared with control subjects when stallions were exposed to an 8:16 photoperiod for 20 weeks beginning in July and then 16:8 for 15 months thereafter, indicating that seasonal sexual recrudescence can be photoinducible. In addition, the study demonstrated that continued exposure to a stimulatory photoperiod does not maintain a heightened reproductive capacity indefinitely and testicular regression does ensue. Cox and co-authors89 found that increasing day length using artificial lights at the end of the breeding season in the northern hemisphere significantly decreased testosterone concentrations, suggesting a negative impact on the fertility of stallions being transported to the southern hemisphere. Therefore, shorter day length in autumn may provide a critical trigger in resensitizing the stallion to the photoinducible events of long days. This concept may be important to keep in mind when shipping stallions, especially those with marginal fertility, from the northern hemisphere to the southern hemisphere for breeding purposes. To maintain peak reproductive performance in these stallions throughout the year, it may be important to put them on an intermittent lighting regimen by alternating intervals of short and long days. Further research in this area is warranted.
SPERMATOGENESIS, STEROIDOGENESIS, AND TESTICULAR FUNCTION: ENDOCRINE-PARACRINE-AUTOCRINE FACTORS
Endocrine Factor
The process of spermatogenesis, steroidogenesis, and testicular function is regulated by a complex interplay of endocrine, paracrine, and autocrine signals.3–8,90–99 The master control is GnRH that signals the pituitary to produce LH and FSH. LH binds to receptors on testicular Leydig cells to produce testosterone, a steroid hormone that diffuses into the seminiferous tubules. Within the seminiferous tubules only Sertoli cells possess receptors for testosterone and FSH and thus these cells are the major targets of the ultimate hormonal signals that regulate Sertoli cell factors that support spermatogenesis. Reports clearly indicate that testosterone is essential for maintenance and restoration of spermatogenesis in the adult testes.99 Although there is general agreement that FSH is of critical importance in the initiation and expansion of spermatogenesis in mammals during puberty,100 the role of FSH in the regulation of spermatogenesis in the adult mammal is still controversial.101 Spermatogenesis has been shown to be maintained or restored quantitatively by testosterone alone in adult rats actively immunized against GnRH, suggesting that FSH is not required for those events in the adult rat.102 However, there is strong evidence to suggest that, in the human and non-human primate, under certain conditions, FSH synergizes with testosterone to maintain quantitatively normal spermatogenesis in the adult.1,103 The controversy has led to a very basic physiological question: Why have two hormones to regulate spermatogenesis, rather than just one? One reason may be that each hormone has differential effects on sperm production. According to Zirkin and co-authors,101 in nearly all mammals, testosterone alone acting as a local factor is capable of maintaining qualitatively complete spermatogenesis, whereas the role of FSH may be to influence the quantity of spermatozoa produced at the premeiotic and postmeiotic levels, depending on the species. In addition, the increasing volume of data with regard to the involvement of local paracrine-autocrine modulation of spermatogenesis not only suggests a more complicated story, but also supports the conceptual argument that one hormone or local testicular factor alone cannot do the job as efficiently as several. Other hormones such as insulin, vasopressin, and oxytocin have been implicated as endocrine factors that regulate testicular function.90 Estrogen, a product of testosterone, has recently been determined to have significant importance in regulating steroidogenesis and spermatogenesis.93,94
The role of FSH and testosterone in the development and maintenance of spermatogenesis in the stallion is not entirely clear. Endocrine profiles of colts prior to and during puberty suggest a differential role of FSH and testosterone on spermatogenesis.2 Prior to puberty, serum FSH concentrations in the colt peaked at about 40 weeks of age, remained relatively high, and then increased again at the time of puberty (~83 weeks). Interestingly, serum concentrations of LH also peaked at around 40 weeks of age, declined to baseline, and then rose again at the time of puberty. Testosterone levels prior to puberty remained low and did not increase at 40 weeks in conjunction with the LH or FSH rise but then rapidly peaked at the time of puberty. These findings suggest that FSH in the colt may be the hormone critical for Sertoli cell development and proliferation prior to puberty, whereas testosterone triggers Sertoli cell differentiation and maturation necessary for gene expression of various paracrine factors important for germ cell development. In adult stallions FSH may have more of a minor role on maintenance of spermatogenesis since it has been reported that FSH receptor numbers in rats decline in the adult Sertoli cell,104 and seasonal changes of FSH are not always apparent in the stallion.81,84,85 In the adult stallion, estrogen and not testosterone may play a major role in Sertoli and germ cell function. When stallions were given a GnRH antagonist, testosterone levels were not affected and only the decline in LH, FSH, and estradiol concentrations could be correlated with morphological evidence of testicular degeneration.105 The concept that testosterone may not be the critical hormone in supporting spermatogenesis in the stallion is also substantiated by a study carried out investigating the plasma hormone concentrations of fertile, subfertile, and infertile stallions. Plasma inhibin, E2, and estrogen conjugates (ECs) were significantly lower and gonadotropins significantly higher in idiopathic infertile stallions, whereas plasma T levels did not change.22
Paracrine-Autocrine Factors
In addition to the endocrine control of LH and FSH on testicular function and sperm production, local testicular steroids, proteins, and peptides called paracrine-autocrine factors coordinate the various functions of the different testicular cell types and/or modulate the testicular actions of pituitary gonadotropins according to local conditions and requirements (Fig. 2-2). Paracrine factors are produced by one cell and act on a different cell type, whereas autocrine factors are produced by one cell and act on the same cell type. Potential paracrine-autocrine regulators of testicular function and spermatogenesis of proven physiological significance include such factors as estrogen, testosterone, inhibin, activin, GnRH-like peptides, growth factors like IGF-1and transforming growth factor alpha and beta (TGF-α and β),oxytocin, vasopressin, pro-enkephalins, enkephalins, proopiomelanocortin (POMC), and POMC-derived peptides such as β-endorphins (β-EPs), cytokines, transferrin, sulfated glycoprotein-l (sGP-l) and sGP-2, insulin-like peptide 3 (INSL3) and PmodS, a paracrine factor secreted from peritubular myoid cells that modulates Sertoli cell function.3–8,90–99 It is beyond the scope of this chapter to discuss the roles of each of these local factors, so only those that have been investigated in the stallion will be reviewed: IGF-1, transferrin, inhibin, activin, estrogen, testosterone, INSL3, POMC, and β-EPsand oxytocin.
Insulin-Like Growth Factor-1(IGF-1)
A publication by Gnessi and co-authors7 provides an overview of the expression, localization, production, and testicular function of IGF-1 in mammalian species. IGF-1, a single-chain polypeptide, is present in peritubular myoid cells, Sertoli cells, and Leydig cells. Receptors for IGF-1 have been found on the cell membrane of germ cells, Leydig and Sertoli cells, and myoid cells. IGF-1 receptors can be up-regulated by LH, FSH, and GH, with LH being the most important factor. The actions of IGF-1 can be modified by its binding protein (IGFBP), which may enhance or inhibit the effects of IGF-1. IGF-1 stimulates the proliferation of prepubertal Sertoli cells and has a small mitogenic effect on immature Leydig cells. IGF-1 stimulates testosterone production and potentiates hCG-induced testosterone formation. Furthermore, IGF-1 is believed to be involved in spermatogenesis. IGF-1 stimulates spermatogonial DNA synthesis and has a maintaining effect on premeiotic DNA synthesis in the rat under in vitro conditions, and IGF-1 induces the differentiation of mouse type A spermatogonia. More recently, IGF-1 has been shown to have anti-apoptotic effects on Leydig cells and germ cells.106,107
IGF-1 has been identified in testicular tissue and in seminal fluid in the stallion.24,108 Hess and Roser24 reported that in colts less than 2 years of age, IGF-1 concentrations in plasma and testicular extracts were higher than in the other age groups and were higher in the breeding season than in the non-breeding season. Seasonal changes in IGF-1 were not observed in stallions older than 2 years of age. No significant differences in plasma or testicular extract concentrations of IGF-1 were found among fertility groups. The results of this study demonstrate that plasma and testicular IGF-1 levels are high in stallions younger than 2 years of age and then decline and plateau in stallions older than 5 years of age, suggesting that IGF-1 may be involved in testicular development. Circulating and intra-testicular levels of IGF-1do not appear to correlate with declining fertility in stallions tested, suggesting that IGF-1 may not be a reliable biomarker for the diagnosis of subfertility and infertility using those biological samples.24 However, there is some evidence to suggest that measurement of IGF-1 in seminal plasma may be a good indicator of fertility.108 A positive but variable relationship between IGF-1 and sperm morphology and motility was noted. Stallions with high levels of IGF-1 in seminal plasma achieved higher pregnancy rates in mares, suggesting that IGF-1 may play a role in sperm function. Using equine testicular cell culture techniques, Roser43 observed that the highest IGF-1 production rates in Leydig cells appears to be during puberty, whereas highest production rates in Sertoli cells occur prior to puberty. Although LH and GH do not appear to regulate IGF-1 in Leydig cells, FSH does appear to stimulate IGF-1 from Sertoli cells but only prior to puberty.43 IGF-1 does not appear to stimulate a steroidogenic response from equine Leydig cells in culture.74 Preliminary studies in our laboratory indicate that IGF-1 may have anti-apoptotic effects on equine Leydig and Sertoli cells.
Transferrin
An excellent review by Sylvester and Griswold109 discusses the physiology of transferrin in the mammalian testes. Transferrin is an iron-binding protein that delivers iron as a nutritional component to germ cells. Sertoli cells synthesize transferrin and, when bound to iron, secrete the complex into the adluminal space. The adluminal testicular iron-bound transferrin is then available to receptors on spermatocytes. Histological examination of testes from mutant mice lacking normal transferrin revealed a decreased number of germ cells and a greatly reduced level of spermiation. Transferrin has been identified in human seminal plasma, and evidence indicates that the measurement of seminal fluid transferrin may be an indicator of the function of Sertoli cells in intact human males. In bulls there was a strong positive correlation between sperm output and transferrin concentrations in seminal fluid, suggesting that transferrin production should be a good indicator of Sertoli function and spermatogenic potential in bulls.110
In the stallion, transferrin is produced by Sertoli cells with the highest production rate occurring at the time of puberty.42 Ongoing studies in our laboratory in collaboration with Dr. Joyce Parlevliet (Utrecht University, The Netherlands) are investigating seminal plasma transferrin concentrations in stallions with various degrees of fertility.
Androgens and Estrogens
In other species it has been demonstrated that androgens and estrogens regulate the function of Sertoli cells, Leydig cells peritubular myoid cells, and germ cells by binding to their respective receptors and eliciting a cellular response.8,94,111–114 By acting through its receptors in Sertoli cells, testosterone appears to be responsible for maintaining an adequate blood-testis barrier function115 and inducing meiosis and postmeiotic development of germ cells.8,113 Testosterone has been shown to inhibit germ cell apoptosis.116 There also appears to be an androgen action on Sertoli cells that modulates their gene expression, proliferation, and differentiation.8,113 Studies using a tissue-specific knock-out mouse with the androgen receptor gene deleted demonstrated an alteration in the expression of several key steroidogenic enzymes in Leydig cells, suggesting that testosterone is an autocrine factor regulating its own production.117
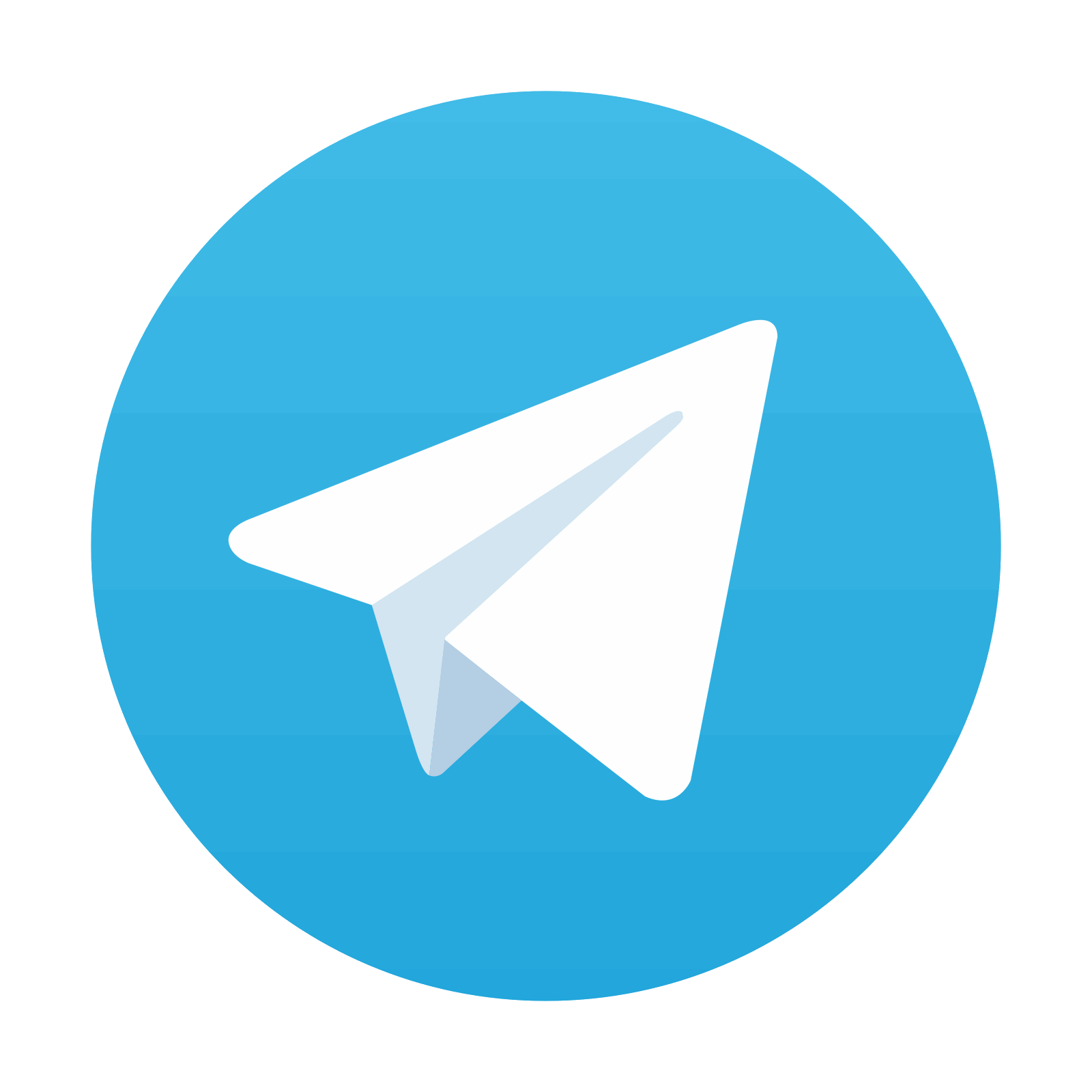
Stay updated, free articles. Join our Telegram channel

Full access? Get Clinical Tree
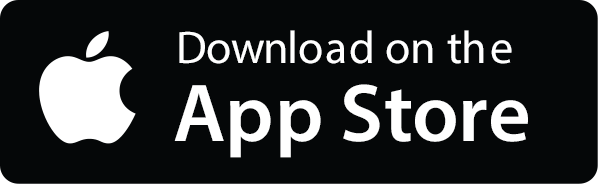
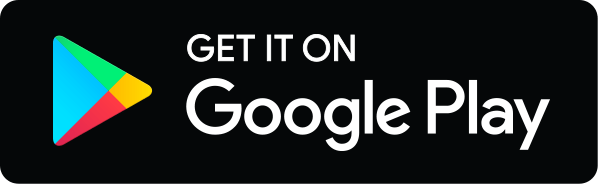