Chapter 11 Neutrophil Function A. Adherence and Chemoattractants D. Neutrophil-Mediated Amplification of Inflammation III. VIRULENCE FACTORS PREVENTING NEUTROPHILMEDIATED KILLING IV. ACQUIRED NEUTROPHIL FUNCTION DEFECTS A. Neutrophil Dysfunction in Periparturient Dairy Cattle B. Neutrophil Dysfunction in Neonatal Animals C. Neutrophil Dysfunction Associated with Viral Infection D. Effects of Nutrition on Neutrophil Function E. Effects of Stress on Neutrophil Function V. NEUTROPHIL-MEDIATED TISSUE INJURY A. Neutrophil-Mediated Injury at Sites of Inflammation B. Ischemia/Reperfusion Injury C. Neutrophil-Mediated Injury at Distant Sites D. Role of Neutrophils in Microvascular Thrombosis Pluripotential hematopoietic stem cells in the bone marrow differentiating in response to specific growth factors give rise to committed progenitors and then neutrophils. These cells are characterized by their multilobed nuclei and abundant granules in the cytoplasm (polymorphonuclear neutrophilic granulocytes). Except in disease states, only functionally mature neutrophils are released into the blood from the bone marrow, and these cells contain much of their effector molecules prepackaged in cytoplasmic granules or in the plasma membrane. Mature neutrophils do not multiply but instead are continuously replaced. Neutrophils circulate in the blood for only a few hours and survive in the tissue for only a few days; however, this can be extended by a day or two upon their exposure to various activating agents at sites of inflammation. In any case, neutrophils eventually succumb to programmed cell death by an intrinsic or extrinsic apoptotic pathway. Once neutrophils are recruited into the underlying tissue from the blood and encounter a microorganism, they phagocytize and kill the pathogen to eliminate the infection. This chapter provides an overview of the mechanisms of neutrophil adherence, chemotaxis, phagocytosis, and organism killing. It also discusses congenital and acquired defects in neutrophil function that predispose animals to infection. Finally, this chapter discusses neutrophil-mediated tissue injury. The recruitment of neutrophils to sites of tissue injury and infection is a hallmark of the acute inflammatory response. Efficient neutrophil extravasation at sites of inflammation requires a coordinated cascade of adhesive and signaling events. Neutrophils leave the flowing blood stream by first tethering and then rolling on the inflamed endothelium lining the blood vessel lumen. In the systemic circulation, this occurs primarily as neutrophils enter the venule from the capillaries (postcapillary venules) and in the collecting venules (Atherton, 1972; Schmid-Schonbein et al., 1980). With the appropriate chemoattractants, rolling neutrophils will arrest (stop rolling) becoming firmly adherent to the endothelial surface and then migrate across the endothelium. Estimates from in vitro studies of leukocyte extravasation show the process to be rapid, with transmigration being completed in <2 minutes (Beesley et al., 1978; Burns et al., 1997). Neutrophil transendothelial migration can occur through distinct routes, either paracellular (between endothelial cells) or transcellular (through endothelial cells) (Feng et al., 1998; Yang et al., 2005). Some of the first observations of leukocyte interactions with the vascular wall come from Albrecht von Haller (1756), Rudolf Virchow (1871), and Rudolf Wagner (1839). Early mechanistic insights into leukocyte transmigration came from Julius Conheim (1877, 1889) and Elle Metchnikoff (1893), who attributed leukocyte emigration to activities primarily by endothelial cells or leukocytes, respectively. Research since the 1980s has greatly enhanced our understanding of the molecular basis for the regulation of leukocyte interactions with the vascular endothelium at sites of inflammation. The following is a general overview of the various physiological parameters, adhesion molecules, and chemoattractants that play a critical role in neutrophil capture/rolling, activation, and arrest/locomotion. Neutrophil accumulation predominantly occurs along the vascular endothelium of venules as a result of several physiological factors. Neutrophil capture by the endothelium is enhanced by red blood cells, those lined up behind neutrophils as they leave the capillaries as well as by red blood cells occupying the center of the bloodstream, which force neutrophils toward the venular endothelium (Nobis et al., 1985; Schmid-Schonbein et al., 1980). Endothelial cells lining postcapillary venules in secondary lymphoid organs of humans and mice (high endothelial cell venules) bulge into the luminal space, thus increasing their collision with lymphocytes (Von Andrian, 1996). Though endothelial cells lining venules in nonlymphoid tissues tend to be flat, attached neutrophils at sites of inflammation appear to provide temporary extensions into the blood vessel lumen, causing collisions and capture of free-flowing neutrophils. This process is referred to as indirect or secondary capture (tethering) and was observed as early as 1955 when Allison and colleagues reported that “leukocytes were frequently seen to stick to one another, indicating that the increased adhesiveness characteristic of the inflammatory response is not limited to the endothelium” (Allison et al., 1955). Indeed, neutrophils individually and as a monolayer facilitate indirect capture, and this appears to accelerate and sustain their accumulation (Bargatze et al., 1994; Eriksson et al., 2001; Sperandio et al., 2003; St. Hill et al., 2003; Walcheck et al., 1996). In addition, because leukocyte accumulation on activated endothelium at sites of inflammation shows spatial variability (Kunkel et al., 1998), indirect capture may be a mechanism for compacting (pavementing) leukocytes within discrete sites of activated endothelium, as illustrated in Figure 11-1. FIGURE 11-1 Indirect (2°) neutrophil capture increases their accumulation rate and density on the vascular endothelium. Direct neutrophil binding to the endothelium (e.g., via P-selectin) occurs randomly (open circles). Next, neutrophil-neutrophil interactions mediate two means of indirect capture. Individual attached neutrophils capture free-flowing neutrophils (gray circles) during initial neutrophil accumulation. In addition, a monolayer of attached neutrophils will capture free-flowing neutrophils, which roll across the neutrophil monolayer (black circles containing arrows) resulting in pavementing by the neutrophils and their compaction in discrete locations. Neutrophils from the flowing blood that adhere to the vascular endothelium incur forces (wall shear stresses) that are affected by several factors, including blood flow velocity, vessel diameter, red blood cell concentration, and plasma viscosity. Shear stresses on adhered neutrophils in venules in vivo range between ∼1 and 35 dynes/cm2, assuming a cell-free, plasma viscosity of 1.1 centipoise (Damiano et al., 1996). In general, leukocyte adhesion and rolling are reduced with increasing wall shear stress (Damiano et al., 1996). The wall shear stress is generally lower in venules than in arterioles of equal size. These forces are balanced by the interaction of adhesion molecules on the leukocyte with those on the endothelium, which is discussed in more detail later. Selectins mediate the initial interactions between neutrophils and the endothelium resulting in their attachment and rolling at velocities considerably below that of cells in the flowing blood. The nature of cell adhesion mediated by selectins differs from that mediated by most other classes of adhesion molecules. Rapid on and off bonding events between selectins and their ligands cause leukocytes attached to the endothelium to roll under blood flow conditions (Fig. 11-2). There are three known selectins, each with a highly specific pattern of cellular expression. Forgoing the listing of all of their earlier more descriptive names, they are known today by single letters designating the cell type by which they were first identified. L-selectin (CD62L) is expressed by leukocytes, E-selectin (CD62E) expression is limited to endothelial cells, and P-selectin (CD62P) is expressed by platelets as well as endothelial cells. FIGURE 11-2 Multistep process of neutrophil effector functions. Neutrophils in flowing blood initially accumulate along the vascular endothelium by direct (1°) or indirect (2°) capture. The tethered neutrophils then roll and upon chemoattractant recognition become activated. This leads to firm adhesion and transendothelial cell migration. Upon entering the underlying tissues, neutrophils phagocytize bacterial pathogens, which induces their programmed cell death (apoptosis). Eventually, the apoptotic neutrophils are recognized and engulfed by macrophages to down-regulate the inflammatory response. Additionally, this figure depicts the site of classical leukocyte adhesion deficiency (LAD-1), a deficiency of CD18, and LAD-2, a defect in the selectin-mediated adhesion. The selectins are type I integral membrane proteins composed of distinct tandem protein domains. At the amino-terminus is a C-type (Ca+2-dependent) lectin domain. Following this is a single epidermal growth factor (EGF)-like domain, followed by a series of short-consensus-repeat (SCR) domains. Next are a transmembrane domain and a short cytoplasmic region. The genes for the selectins are arranged in tandem on chromosome 1 for both mouse and human, indicating that they arose by gene-duplication (Watson et al., 1990). Selectin ligands are carbohydrate in nature, and the lectin domain of the selectins is responsible for ligand specificity and recognition (Weis et al., 1998). E-selectin expression is induced on most cultured endothelium as well as most postcapillary endothelium in vivo by a number of inflammatory mediators (Bevilacqua et al., 1989). Induction of E-selectin by cytokines occurs exclusively at the transcriptional level. E-selectin mRNA is first detectable approximately 2 h after initial exposure of endothelial cells to cytokine. In cultured endothelium, E-selectin expression peaks in 4 to 8h, and subsequently declines even in the continued presence of the cytokine. In vivo, however, E-selectin can be chronically expressed at sites of inflammation, especially in the skin (Ley and Kansas, 2004). P-selectin is expressed on both activated platelets and activated endothelium. Unlike E-selectin, P-selectin is expressed in the Weibel-Palade bodies of endothelial cells as well as the α-granules of platelets (Bonfanti et al., 1989; McEver et al., 1989). Exposure of either of these two cell types to various stimuli induces rapid (seconds to minutes) fusion of the P-selectin-containing granules with the outer membrane of the cell, exposing P-selectin at the cell surface. In the case of endothelial cells, histamine released from tissue mast cells in response to an inflammatory insult is one of the physiological inducers of rapid P-selectin expression. Interestingly, P-selectin-deficient mice have a defect in leukocyte rolling immediately following tissue exteriorization, as determined by intravital microscopy, and neutrophil recruitment into inflammatory lesions is delayed by about 2h (Mayadas et al., 1993). In addition to its rapid surface expression, P-selectin is also transcriptionally induced (Weller et al., 1992). Moreover, P-selectin can be chronically expressed at sites of long-standing inflammation, such as atherosclerotic vessels, rheumatoid arthritis, and psoriasis (Grober et al., 1993; Johnson-Tidey et al., 1994; Terajima et al., 1998). Neutrophils uniformly and constitutively express L-selectin. This selectin arguably plays the broadest and most significant role of the family members in neutrophil accumulation on the endothelium as it mediates both direct and indirect capture. In an assortment of inflammatory models, leukocyte accumulation along the microvasculature and recruitment into the tissue were greatly reduced (∼60% to 85%) upon blocking L-selectin function with antibodies or in L-selectin-deficient mice (Davenpeck et al., 1997; Jung et al., 1998; Kanwar et al., 1999; Ley et al., 1993; Tedder et al., 1995; Von Andrian et al., 1991, 1992, 1993). Consistent with L-selectin’s broad role in neutrophil recruitment, it is tightly regulated. For instance, neutrophils down-regulate L-selectin expression by ectodomain proteolysis (shedding) in a very efficient and rapid manner upon their stimulation by cytokines and chemoattractants (Alexander et al., 2000; Kishimoto et al., 1989; Rizoli et al., 1999; Walcheck et al., 1996). Studies performed in vitro and in vivo indicate that L-selectin shedding affects leukocyte adhesiveness and recruitment (Hafezi-Moghadam and Ley, 1999; Venturi et al., 2003, Walcheck et al., 1996). In addition, homeostatic L-selectin shedding maintains high levels of soluble L-selectin in the serum (∼1 to 3 μg/ml) (Kishimoto et al., 1995; Palecanda et al., 1992), which is functional and can diminish leukocyte-endothelium interactions. Soluble L-selectin may thus serve as an adhesion buffer to suppress neutrophil accumulation below a certain inflammatory threshold. Interestingly, a reduced level of serum L-selectin correlates with susceptibility to inflammatory disease (Donnelly et al., 1994; Seidelin et al., 2002). TNF-α converting enzyme (TACE or ADAM17) appears to be a key sheddase of L-selectin upon overt cell activation. The selectin family of adhesion proteins is highly selective lectins that recognize specific glycan structures displayed in most cases on an appropriate protein backbone. The main glycoprotein ligand for P-selectin on neutrophils is a homodimeric sialomucin designated PSGL-1 (P-selectin glycoprotein ligand-1) (Moore et al., 1995; Sako et al., 1993). PSGL-1 also serves as the primary ligand for L-selectin during leukocyte-leukocyte facilitated indirect neutrophil accumulation along the endothelium (Walcheck et al., 1996). Importantly, specific posttranslational modifications of PSGL-1 are required for L- and P-selectin recognition. PSGL-l has a tyrosine sulfate motif at its amino-terminus, and mutational analyses have revealed that at least one of the three tyrosines in the motif must be sulfated for recognition (Pouyani and Seed, 1995; Sako et al., 1995). In addition, a threonine residue just downstream from the tyrosine sulfate motif must be modified by sialylated, fucosylated oligosaccharides, such as a core 2 O-glycan terminated by sialyl Lewis X (sLeX) (Leppanen et al., 1999, 2003). PSGL-1 has also been reported to function as a ligand for E-selectin (Asa et al., 1995; Sako et al., 1993). However, leukocytes derived from PSGL-l knockout mice demonstrated no obvious defect in rolling on E-selectin (Yang et al., 1999). Altogether, various studies suggest that PSGL-1 expression by neutrophils is not as critical for E-selectin binding as it is for L- and P-selectin. Indeed, other E-selectin ligands on neutrophils have been described, including E-selectin ligand-1 (ESL-1), glycolipids, and L-selectin (Alon et al., 1995; Steegmaier et al., 1995). In addition to recognizing its leukocyte ligand PSGL-1, L-selectin on neutrophils also mediates their direct accumulation on the endothelium, yet these endothelial ligands remain poorly characterized. Chemoattractants are small soluble molecules that bind to receptors on leukocytes causing their stimulation, polarization, and locomotion, in part through the activation of the integrin adhesion molecules. Leukocyte locomotion toward higher concentrations of a chemoattractant is referred to as chemotaxis, and this can occur in a hierarchal manner. For instance, neutrophils become less sensitive to an initially encountered chemoattractant gradient, allowing them to then respond to a newly encountered chemoattractant (Foxman et al., 1997, 1999). This process allows neutrophils to find their ultimate target through a complex stimulant environment. Different classes of chemoattractants include chemokines, lipid mediators, complement factors, and other peptides. The largest class of chemoattractants constitutes the chemokines, a superfamily of small, secreted proteins (8 to 10 kDa). The chemokines are structurally homologous and are subdivided into four subfamilies based on the position of conserved cysteine residues near the amino-terminus of the molecules (Rot and von Andrian, 2004). CXC chemokines (α-chemokines) are characterized by the presence of two cysteine residues that are separated by one amino acid, CC chemokines (β-chemokines) have two cysteine residues that are adjacent, C chemokines have one cysteine residue near the amino-terminus, and the CXXXC subfamily is characterized by cysteines being separated by three amino acids. The CC and CXC chemokines represent the largest of the chemokine families and contain many members. The C and CXXXC subfamilies together consist of three members at this time. The CXC chemokines can be further subdivided based on the presence or absence of a glutamatic acid-lysine-arginine (ELR) sequence preceding the CXC motif. This structural difference determines separate functional activities of chemokines in this subfamily. CXC chemokines that contain an ELR sequence (e.g., IL-8) are chemotactic for neutrophils, whereas non-ELR-containing CXC chemokines and CC chemokines appear not to be active on neutrophils (DeVries et al., 1999). Chemokines induce leukocyte activation and locomotion by binding to specific G-protein-coupled cell surface receptors. Most chemokine receptors recognize more than one chemokine, and several chemokines can bind to more than one receptor. However, there is receptor-ligand specificity within chemokine subfamilies, with CXC chemokines binding exclusively to CXC receptors and CC chemokines binding to CC receptors (Rot and von Andrian, 2004). Integrins mediate neutrophil firm adhesion and locomotion by interacting with ligands on cells and in the extracellular matrix with a dependence on divalent cations (Fig. 11-2). Integrins are noncovalent heterodimers (α and β subunits) of type I transmembrane glycoproteins, and are made up of various subfamilies that are organized around a particular subunit (Harris et al., 2000). For instance, the β2 (CD18) subfamily is expressed on all cells of hematopoietic origin and plays a critical role in neutrophil transendothelial cell migration (Simon and Green, 2005) and other key effector activities, such as phagocytosis and apoptosis (Mayadas and Cullere, 2005). CD18 integrins are present in an inactive state on circulating leukocytes; however, upon cell stimulation with various stimuli (e.g., chemoattractants) and ligand interactions, they undergo rapid affinity and valency changes required for optimal integrin function (Carman and Springer, 2003). There are four known α (CD11) subunits that can combine with the CD18 subunit to form unique receptors. The α subunits are defined, in part, as CD11a, b, c, and d. The α and β subunits are expressed from different genes and can be independently expressed on different cell types. CD11a/CD18 (αLβ2 or lymphocyte function-associated antigen [LFA-1]) is the most broadly expressed leukocyte integrin. It is expressed on early hematopoietic progenitor cells and on all mature leukocytes. LFA-1 interacts with cell surface ligands known as intercellular adhesion molecules (ICAMs). ICAMs are type I transmembrane glycoproteins and belong to the immunoglobulin superfamily (Kishimoto and Rothlein, 1994). ICAM-l is expressed on resting endothelium and is induced on many cell types by inflammatory cytokines. LFA-1 also binds to ICAM-2 or ICAM-3, which are mainly expressed on endothelial cells and leukocytes, respectively. ICAM-l is a more active LFA-l ligand than ICAM-2 or ICAM-3. CD11b/CD18 (αMβ2 or Mac-1) is constitutively expressed by neutrophils, monocytes, and macrophages. Mac-l is stored in endosome-like vesicles in neutrophils that are translocated to the surface following certain types of activation, allowing increased Mac-l surface expression (Borregaard et al., 1987). Mac-1, like LFA-l, is less active on resting cells than activated cells. Mac-l also interacts with ICAM-l, but at a site distinct from LFA-l (Diamond et al., 1991). Both of these CD18 subfamily members function as the primary integrins that mediate arrest and transmigration at sites of inflammation. Mac-1 interacts with a wider variety of ligands compared to LFA-l, including fibronectin, fibrinogen, vitronectin, laminin, collagen, ICAM-2, albumin, myeloperoxidase, kininogen, elastase, heparin, and zymosan (Kishimoto and Rothlein, 1994; Simon and Green, 2005). Mac-l also participates in a number of adhesion reactions where the nature of the ligand is not known, such as adhesion of neutrophils to plastic or glass surfaces and binding to a variety of denatured proteins, suggesting a scavenger receptor-like function. Of particular importance is that Mac-1, also referred to as complement receptor 3, binds to C3bi. This complement fragment bonds to surfaces when triggered by immunoglobulin or microbial surfaces, and particles coated with C3bi (opsonization) are readily phagocytized by neutrophils (Mayadas and Cullere, 2005). CD11c/CD18 (αXβ2 or P150,95) is highly homologous to Mac-1, but it is expressed at lower levels on neutrophils and not nearly as well studied. Like Mac-1, CD11c/CD18 binds to some of the same ligands, including C3bi as well as binding to many pathogens directly to promote their phagocytosis, fibrinogen, and a variety of denatured proteins (Kishimoto and Rothlein, 1994; Mayadas and Cullere, 2005). CD11d/CD18 (αDβ2) is the most recently defined member of the leukocyte integrin family and is expressed on tissue macrophages, dendritic cells, and eosinophils and binds to ICAM-3 and vascular cell adhesion molecule-l (VCAM-l) (Danilenko et al., 1995; Grayson et al., 1998; Van der Vieren et al., 1995). The later binding interaction may contribute to recruitment of eosinophils to inflamed airways. Leukocyte adhesion deficiency (LAD) syndromes (LAD-1, LAD-1 variants, and LAD-2) result in immunodeficiency disorders caused by an impaired extravasation of neutrophils into sites of inflammation because of dysfunctional selectin- or integrin-mediated adhesion events (Fig. 11-2). The LAD syndromes are relatively uncommon; however, the clinical consequences are often severe and lethal and include recurrent or unresolved localized infections, systemic sepsis, and impaired wound surveillance and repair. Classical leukocyte adhesion deficiency type I (LAD-1) is an autosomal recessive disorder arising from germline mutations in the gene encoding CD18 and thus affects all CD18 subfamilies. LAD-1 has been described in humans, Holstein cattle, and dogs (Anderson and Springer, 1987; Gu et al., 2004; Nagahata, 2004). In humans, the CD18-integrin subunit is either not synthesized or unable to associate with the respective CD11 subunits, and the resulting LAD can be characterized as severe to moderate. Children with severe deficiency typically display less than 1% of normal levels of CD11/CD18, and children with moderate deficiency typically express 5% to 10% of normal levels of the integrin. Bovine leukocyte adhesion deficiency (BLAD) has been extensively investigated (Nagahata, 2004; Shuster et al., 1992). The molecular basis of BLAD is a single point mutation that results in an aspartic acid to glycine substitution at amino acid 128 of the CD18 polypeptide. Interestingly, expression of L-selectin on neutrophils from BLAD calves appears to be reduced as well. Neutrophil adhesion is markedly impaired and chemotactic responses are diminished. The hallmark clinical findings in BLAD include recurrent or chronic diarrhea, chronic pneumonia, ulcerative stomatitis, gingivitis, chronic dermatitis, stunted growth, and impaired wound healing. Persistent and severe neutrophilia is the most prominent hematological finding; however, neutrophil morphology is normal. Pathological lesions are primarily located in the lungs and intestinal tract. Neutrophils are present within the lung lesions but not within intestinal ulcers or lesions in other tissues. Therefore, neutrophils can enter the lung by integrin-independent processes (Ackermann et al., 1996). Canine leukocyte adhesion deficiency (CLAD) occurs in Irish setter and Irish setter-cross-bred dogs (Giger et al., 1987; Gu et al., 2004; Kijas et al., 1999; Renshaw et al., 1975). As with BLAD, the molecular basis of CLAD is due to a single point mutation; however, in the dog, a cysteine at amino acid 36 is substituted for a serine. This cysteine is a highly conserved residue in the extracellular domain of CD18, and the structural defect from the mutation results in the failure to express the CD11/CD18 complex on the leukocyte surface. Affected dogs develop severe bacterial infections shortly after birth and usually die or are euthanized. More recently, variant forms of LAD-1 have been reported in humans in which CD18 integrins are expressed on the surface of leukocytes at normal levels but fail to support their adhesion (Wehrle-Haller and Imhof, 2003). In one case, LAD-1 was caused by a point mutation in the β2-integrin, rendering it nonresponsive to inside-out activation of leukocytes (Hogg et al., 1999). In other cases, the leukocyte adhesion deficiency was extended to various integrin families as well, including β1-, β2-, and β3-integrin function. Interestingly, these latter cases result from a failure by intracellular factor(s) or signaling pathway(s) essential for integrin activation/clustering. Under physiological shear stress, these LAD-affected leukocytes are unable to generate firm adhesion and arrest. Another human adhesion deficiency (LAD-2) was first described in 1992 in two children of Arab origin (Etzioni et al., 1992). LAD-2 is a rare congenital disease resulting in mutations in a GDP-fucose transporter and a generalized fucosylation defect that affects α1,2-, α1,3-, α1,4-, and α1,6-linkages of fucose in glycoproteins and glycolipids (Wild et al., 2002). Among other effects, LAD-2 neutrophils demonstrate strongly reduced sLeX expression, which diminishes their adhesivity with the selectin family members and their accumulation on endothelium (Fig. 11-2). Thus, this disorder disrupts leukocyte extravasation at an earlier stage than LAD-1. The process of phagocytosis has been extensively studied in macrophages but less well studied in neutrophils. Therefore, we will present a general overview of phagocytosis based on knowledge gained primarily from macrophages. Phagocytic cells ingest bacteria and other particulate material by receptor-mediated phagocytosis (Peyron et al., 2001; Stossel, 1974). This process forms a vesicle around the organism and internalizes it (Fig. 11-3). After internalization, the vesicle containing the organism undergoes a stepwise process of maturation in which the vesicle fuses with early and late endosomes and lysosomes to create a phagolysosome. Within the phagolysosome, lysosomal enzymes and reactive oxygen radicals digest organisms. The process of phagolysosome fusion and organism killing is complex, and the details are still being investigated. Fusion of early endosomes coincides with initiation of vesicle acidification. This phase is marked by the presence of high levels of the GTP-binding protein Rab5 on the phagosome membrane. It is not clear whether Rab5 is brought to phagosome by the early endosome or is acquired from the cytoplasm (Via et al., 1997). Fusion of the phagosome with late endosomes is marked by acquisition of Rab7 and loss of Rab5. Subsequent fusion of lysosomes to the late phagosome is characterized by acquisition of lysosome-associated membrane protein (LAMP)-1 and LAMP-2. FIGURE 11-3 Receptor-mediated phagocytosis of organisms by leukocytes. After binding of organisms to cell membrane receptors, organisms are endocytosed into a phagosome. After fusion with early endosomes, the early phagosome is characterized by the presence of Rab5. Acidification of the phagosome occurs at this stage. Thereafter, fusion with late endosomes results in acquisition of Rab7 and shedding of Rab5. The late phagosome then fuses with lysosomes, forming the phagolysosome that is characterized by the presence of Rab7 and Lamp-1. Neutrophils contain primary, secondary, and, in some species, tertiary granules in addition to lysosomes (Stossel, 1974). Primary granules contain oxygen-dependent killing mechanisms and a variety of cationic proteins and bactericidal/permeability-increasing (BPI) protein (Klebanoff and Clark, 1978). Secondary granules contain most of the lysozyme and lactoferrin. Secondary granules appear to fuse first followed by primary granules (Klebanoff and Clark, 1978). Phagosome-to-endosome fusion is a highly controlled process. The fusion system is typically composed of specific receptor-mediated processes. An ATPase N-ethyl-maleimide-sensitive factor (NSF) and α soluble NSF attachment protein (αSNAP) interact with vesicle and target integral membrane SNAP receptors (v- and t-SNAREs) that serve as docking and fusion devices (Vieira et al., 2002). v- and t-SNAREs form complexes spontaneously, and NSF and SNAP provide the energy necessary to separate the v- and t-SNAREs. When separated, SNAREs from apposed membranes are paired resulting in fusion of the lipid bilayers. However, fusion occurs in a highly controlled manner being regulated primarily by an Src family of RAB GTPases (Peyron et al., 2001). Phagolysosome fusion is a critical event in killing of pathogenic organisms. Defective phagolysosome fusion occurs in Chediak-Higashi syndrome. Chediak-Higashi syndrome has been described in several breeds of cattle, Persian cats, foxes, mink, and a killer whale (Ayers et al., 1988; Colgan et al., 1992). Humans and animals have partial oculocutaneous albinism, frequent infections, and mild bleeding diatheses. Neutrophils, monocytes, and melanocytes contain giant granules that result of abnormal fusion events that appear to be due to defects in lysosomal docking proteins. Granule membrane fusion is defective and discharge of granule contents into the phagosome is delayed (Mills and Noya, 1993). The mature phagolysosome has multiple mechanisms to kill microorganisms. These have generally been divided into oxygen-dependent and oxygen-independent mechanisms (Klebanoff, 1975). Oxygen-dependent mechanisms are located within primary granules (Babior, 1984; Babior et al., 2002). These mechanisms are initiated by the process of phagocytosis or by perturbation of the cell membrane. A membrane-bound nicotinamide-adenine dinucleotide phosphate (NADPH) oxidase consists of five essential protein components; two of these are in the membrane and three are in the cytosol (Fig. 11-4). The membrane-associated component is a flavocytochrome b558 that consists of a heterodimer composed of 91 (gp91phox) and 22 (gp22phox) kDa proteins. Flavocytochrome b558 is distributed in the cell membrane and in the membrane of the granules and is incorporated into the wall of phagocytic vacuoles. The cytosolic components consist of a 40-kDa protein (p40-phox), a 47-kDa protein (p47-phox) and a 67-kDa component (p67-phox). When stimulated, the cytosolic components are translocated to the membrane. Both the assembly of the NADPH oxidase complex and the electron flow are dependent on the influence of three GTP-binding proteins; Rac1, Rac2, and Rap1A. The NADPH oxidase complex catalyzes the reduction of molecular oxygen to superoxide anion FIGURE 11-4 The NADPH oxidase complex consists of a membrane-bound flavocytochrome b558 consisting of two subunits (22 and 91 kDa) and three cytosolic molecules (p40, p47, and p67). The assembly of NADPH and electron flow are dependent on three GTP-binding proteins (Rac). Products of the respiratory burst are toxic to cells as well as to invading organisms. A variety of antioxidant systems exist to protect cells from the harmful effect of these oxidants (VanSteenhouse, 1987). These systems include cytosolic superoxide dismutase, glutathione peroxidase, glutathione reductase, and catalase, all of which convert oxygen radicals to water. Other compounds with oxygen radical scavenging potential including vitamin E, vitamin C, selenium, transferrin, and cysteine (VanSteenhouse, 1987). The glutathione needed for the reaction is maintained in the reduced state by reduced NADPH that is supplied by the hexose monophosphate pathway. Several defects in the capacity of neutrophils to generate oxygen radicals have been described. These include chronic granulomatous disease, glutathione peroxidase deficiency, glucose-6-phosphatase deficiency, and myeloperoxidase deficiency (Malech and Nauseef, 1997). Chronic granulomatous disease is caused by mutations in genes encoding components of NADPH oxidase. In excess of 40 specific mutations have been reported in human patients (Cross et al., 2000). Chronic granulomatous disease is characterized by recurrent severe, but usually not fatal, bacterial and fungal infections beginning in early childhood (Malech and Nauseef, 1997). Neutrophils have an inability to mount a respiratory burst because of an inability to activate the NADPH oxidase system. A neutrophil bactericidal dysfunction with decreased capacity to mount a respiratory burst has been described in eight closely related Doberman pinschers (Breitschwerdt et al., 1987). However, the defect in oxygen radical generation was not as severe as in humans, and it is not clear if the defect is in NADPH or in another pathway such as myeloperoxidase deficiency.
I. INTRODUCTION
II. NEUTROPHIL FUNCTIONS
A. Adherence and Chemoattractants
1. Overview
2. Physiological Factors Contributing to
Neutrophil Adherence
3. Selectins
4. Chemoattractants
5. Integrins
6. Leukocyte Adhesion Deficiency
B. Phagocytosis
C. Bactericidal Mechanisms
1. Oxygen-Dependent Mechanisms
. The associated rapid consumption of oxygen has been termed the “respiratory burst.” Superoxide anions are rapidly dismutated to hydrogen peroxide (H2O2). Superoxide anions also can be converted to hydroxyl radicals (OH.) in the presence of catalytic metals such as iron according to the Haber-Weiss reaction (Fe2+ + H2O2→Fe3+ + OH– + OH· + O2). Myeloperoxidase catalyzes the conversion of H2O2 and halide ions to hypohalous acids with hypochlorous acid being the primary acid produced. Finally H2O2 interacts with hypohalous acids to produce singlet oxygen (1O2). Singlet oxygen is a high-energy form of oxygen that can attack double bonds. These reactive oxygen intermediates interact with unsaturated lipids, carbon bonds, sulfhydryl and amino groups, nucleic acids, pyrimidine nucleotides, and enzymes within organisms. The extent to which these events contribute directly to disruption of organism function and organism killing is controversial (Segal, 2005; this subject is discussed in more detail in the next section). Myeloperoxidase is not essential for antimicrobial activity because humans with myeloperoxidase deficiency do not have severe bacterial infections. Additionally chicken heterophils lack myeloperoxidase.
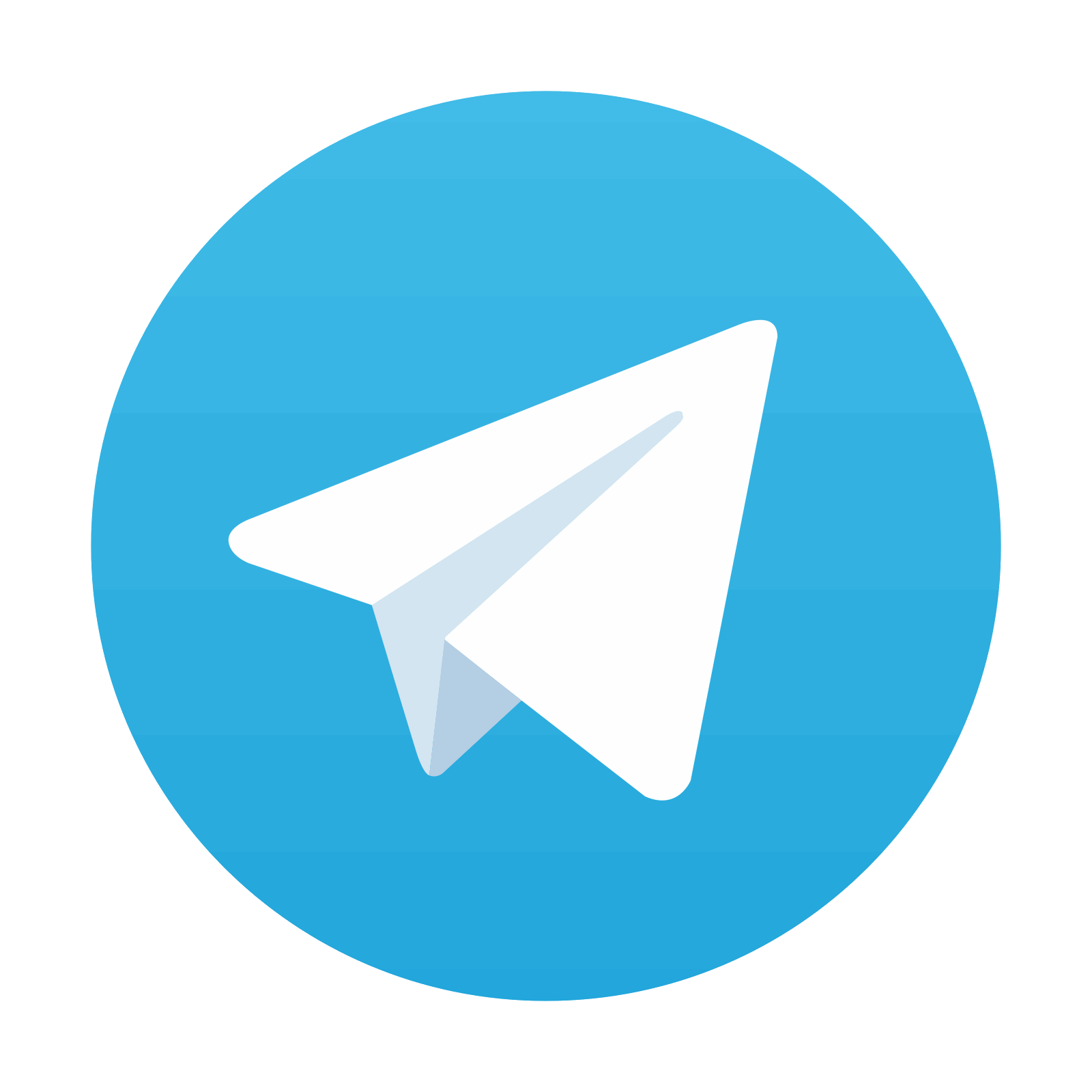
Stay updated, free articles. Join our Telegram channel

Full access? Get Clinical Tree
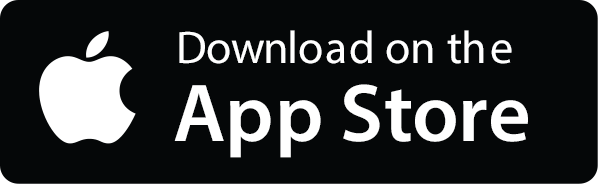
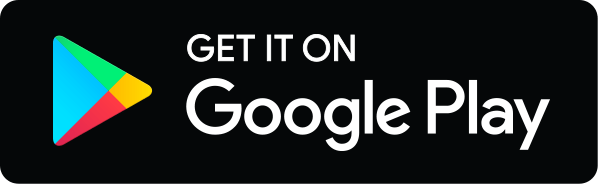