10 Diane E. Mason and Christopher L. Norkus The authors thank HR Adams for the original chapter upon which this is based. Neuromuscular blocking agents used in clinical medicine act by interfering with the action of the endogenous neurotransmitter acetylcholine (ACh) on the nicotinic cholinergic receptor at the neuromuscular junction (NMJ), thereby inhibiting receptor-coupled transmembrane ion movements necessary to initiate muscle contraction (Bouzat et al., 2004; Unwin, 2005). The end result of this action is skeletal muscle paralysis and muscular relaxation (Bowman, 2006). Neuromuscular blocking (NMB) agents are used in veterinary medicine as adjuncts to general anesthesia. The most common indications for a NMB agent in veterinary medicine are to aid in the placement of an endotracheal tube, to be used concurrently with sedative/hypnotic agents to facilitate mechanical ventilation, to enhance muscle relaxation for a variety of surgical conditions (e.g. ophthalmological procedures), or as part of a balanced anesthetic technique to reduce the amount of inhalation anesthetic required (Keegan, 2015). The sporadic use of NMB agents in veterinary medicine is in stark contrast to the widespread use of NMB agents in human anesthesia and is largely a result of interspecies differences. For example, most species seen in veterinary medicine can be easily intubated with an endotracheal tube after administration of an intravenous anesthetic induction agent without the additional need of a NMB agent, while intubation in man is often more difficult and may necessitate deeper muscle relaxation (Bozeman et al., 2006). Development of NMB drugs represents a colorful history in the field of pharmacology. Interesting reviews of the course of these events have been presented by Betcher (1977), Bisset (1992), and Lee (2005). Neuromuscular blocking drugs originated with the discovery of curare, a tarlike mixture of plant material used as a poison by South American Indians. The actual ingredients of the poison for arrows, blowgun darts, and spears were known only to a local “pharmacist,” who was often the tribal medicine man of a region. Thus the botanical preparations obtained by explorers could not be identified as to content; they were simply classified according to the containers in which they were packaged. Tubo-, para-, or bamboo-curare was contained in cutoff bamboo tubes; this mixture was usually obtained from southern Amazon tribes. The plant origin of tube-curare preparations was primarily Menispermaceae (Chondrodendron tomentosum). Calabash-curare was packaged in hollow gourds or calabashes; it was the most active preparation. Pot-curare came in small earthenware pottery from the central part of the Amazon basin; this concoction often contained plants other than Menispermaceae (McIntyre, 1972). The most important constituent isolated from curare was d-tubocurarine (Wintersteiner and Dutcher, 1943). Original studies in the 19th century by Claude Bernard (1856) (Bowman, 2006) demonstrated that curare prevented the muscle contraction elicited by stimulation of the motor nerve. It did not, however, affect the central nervous system (CNS), prevent response to direct stimulation of the muscle, or depress axonal conductance. It was proposed that curare acted at the nerve–muscle junction. Reports since then have substantiated, clarified, and extended observations concerning the neuromuscular blocking properties of curare alkaloids. Early results stimulated active research into the chemical structural requirements of curare-like compounds, leading to the discovery of other types of NMB agents. Neuromuscular blocking agents possess chemical structural groups that allow interaction of these agents with the nicotinic cholinergic receptor (Brejc et al., 2001). However, these drugs cause distinctly different effects from the endogenous mediator ACh. According to the drug’s mechanism of action at the nicotinic postjunctional receptor, NMB drugs are classified as either competitive nondepolarizing agents or as depolarizing agents. Competitive nondepolarizing NMB agents occupy the receptor so that ACh cannot access its binding site and upon binding these compounds fail to trigger transmembrane ion movement, resulting in muscle paralysis for the duration of their effect. Depolarizing agents act in a more complicated manner and initially cause membrane depolarization, often characterized by muscle fasciculation, before blockade and muscle paralysis occur (Hibbs and Zambon, 2011). Prior to discussing individual NMB agents, impulse transmission at the somatic NMJ will be reviewed in relation to sites of action of different drugs. General concepts of cholinergic transmission are discussed in detail in Chapters 6 and 8. A representation of a somatic NMJ synapse and proposed sites of drug actions are shown in Figure 10.1. Terminal branches of a motor axon lose their myelin sheath and embed within invaginations of the cell membrane of the skeletal muscle cell; these invaginations are termed synaptic gutters. A synaptic gutter, in turn, has many microinvaginations or infoldings, called junctional folds. The space within the synaptic gutter between the nerve ending and the muscle cell is called the synaptic cleft. Presynaptic refers to nerve axon elements, whereas postsynaptic refers to constituents of the muscle cell. Figure 10.1 Schematic representation of a somatic neuromuscular junction (synapse), related physiological pathways, and proposed sites of action of various pharmacological agents. An axonal action potential (AP) is characterized by an influx of Na+ and an efflux of K+. Tetrodotoxin and saxitoxin inactivate Na+ pathways. Local anesthetics block Na+ and K+ pathways. Choline uptake into the neuron is blocked by hemicholinium; synthesis of ACh is prevented. As the AP arrives at the nerve terminal, it instigates inward movement of Ca++; this triggers discharge of ACh into the junctional cleft. A lack of Ca++ or an excess of Mg++ decreases release of ACh. Aminoglycoside antibiotics also interfere with Ca++ -dependent release of ACh. Botulinum toxin inhibits ACh release. Succinylcholine (depolarizing neuromuscular blocking agents) cause persistent depolarization block of the motor end-plate region, as does excess ACh and nicotine. Curare and atracurium (competitive neuromuscular blocking agents) compete with ACh for postsynaptic receptors but do not cause depolarization. Aminoglycoside antibiotics decrease sensitivity of the postsynaptic membrane to ACh. Catabolism of ACh by acetylcholinesterase is inhibited by reversible and irreversible anticholinesterase agents; ACh accumulates. Source: Modified from Hibbs and Zambon, 2011; Couteaux, 1972. Vesicular structures localized within cholinergic nerve terminals represent storage sites for ACh (see Chapter 6). As an axonal action potential arrives at the nerve terminal, it increases the release of ACh from the storage vesicles into the synaptic cleft. This step (excitation–secretion coupling) is dependent upon an action potential activating voltage-gated Ca++ channels that shift extracellular Ca++ into the neuron and/or release Ca++ bound to superficial membranes of the nerve terminal. The rise in intracellular Ca++ triggers fusion of the storage vesicles with the plasma membrane, and neurotransmitter release. The ACh released into the synaptic cleft binds to specialized receptor sites on the postsynaptic membrane and causes depolarization of the muscle cell. The exclusively nicotinic cholinergic receptors located on the plasma membrane surface of the muscle cell are clustered in high density in the junctional folds of the postsynaptic membrane (Huh and Fuhrer, 2002). It requires the activation of a large number of nicotinic cholinergic receptors to excite a single muscle fiber and stimulate muscle contraction. At the same time the process requires a very rapid termination of response. A single synaptic vesicle contains roughly 7000–12,000 molecules of ACh and a single motor axon action potential may trigger the fusion of 40–300 vesicles depending on the species or type of NMJ studied (Steinbach and Wu, 2004). After release into the synaptic cleft the ACh reaches high concentration rapidly, where it can bind to cholinergic receptors. However, ACh released into the synaptic cleft also can bind to the enzyme acetylcholinesterase (AChE), which hydrolyzes ACh to choline and acetate thus inactivating it. All unbound, extraneuronal ACh is rapidly metabolized by the AChE enzyme, which is localized in the motor end-plate region. Although AChE may be bound in part to presynaptic elements, it is concentrated at the postsynaptic membrane (Inestrosa and Perelman, 1990; Hucho et al., 1991). The relative ratio of binding sites for ACh on the nicotinic cholinergic receptor to AChE binding sites at the NMJ is approximately 10 : 1 (Steinbach and Wu, 2004). Due to the rapid rate of hydrolysis of ACh by AChE and the comparatively slow release of ACh from relatively large numbers of nicotinic receptors at the motor end-plate, the concentration of free ACh within the synaptic cleft is rapidly reduced after a single action potential. The nicotinic cholinergic receptor is a pentameric molecule of about 290 kilodaltons that spans the bilayer of the postsynaptic membrane at the NMJ (Figure 10.2). The receptor comprises five individual subunits in a stoichiometric ratio of α2βγδ; the γ-subunit is replaced by an ϵ-subunit in muscle from adult animals. Each subunit presents an extracellular and intracellular surface and also contains sequences of hydrophobic amino acids that are the likely regions embedded within the membrane bilayer (Hibbs and Zambon, 2011). The five subunits of each individual receptor complex are elongated perpendicular to the postsynaptic membrane and are arranged circumferentially to form a rosette around a central lumen (Figure 10.2). This central transmembrane channel of the receptor complex represents the membrane pore for ion fluxes instigated by agonist activation of the receptor (Unwin, 2005). Agonist and antagonist binding sites are restricted to the α-subunits (Kistler et al., 1982). Whereas ACh evokes receptor activation upon binding to the α-subunits, occupation of these same sites by antagonists prevents effective receptor activation (Karlin, 2002). The muscle becomes paralyzed, whether in response to a competitive nondepolarizing blocking agent or to transient activation by a depolarizing blocking agent. Chemical structures of several commonly used NMB agents are shown in Figure 10.3 to demonstrate structural differences of the competitive, nondepolarizing and the depolarizing types of NMB agents. Figure 10.2 Schematic representation of the nicotinic cholinergic receptor. The receptor is embedded across the cell membrane lipid bilayer, presenting a synaptic face to the neuroeffector junction between the neuron and innervated cell, and an intracellular face within the cytoplasm. The receptor comprises a pentameric configuration of four separate subunits with a stoichiometric ratio of α2βγδ; in adult muscle α2βϵδ. The α subunits contain the primary ligand binding sites for recognition of acetylcholine and related agents. Subunit arrangement forms an internal pore that allows passage of select ions upon receptor activation and resulting membrane depolarization (see text). Source: Redrawn from Unwin et al. (1988) by Dr. Gheorghe M. Constantinescu, University of Missouri. Figure 10.3 Chemical structures of agents interacting with the nicotinic cholinergic receptor at the neuromuscular junction. The cholinergic neurotransmitter, acetylcholine, contains a cationic nitrogen moiety that forms a bond with receptor binding site. Two molecules of acetylcholine must bind to a nicotinic receptor to activate the ion channel. The depolarizing neuromuscular blocking agent, succinylcholine, is depicted. The chemical structure of succinylcholine is equivalent to two acetylcholine molecules fused in sequence. A single molecule of succinylcholine can bind to both of the nicotinic binding sites on the cholinergic receptor inactivating the receptor. Competitive nondepolarizing NMB agents from the benzylisoquinolinium family (atracurium) and the aminosteroid group (vecuronium) are represented. These compounds are complex bulky molecules but contain the important dual cationic nitrogen moieties in a rigid configuration that allows occupation of both of the nicotinic binding sites on the cholinergic receptor and effectively inactivating the receptor. Based on general chemical structural characteristics, Bovet (1951) placed neuromuscular blocking agents into two basic categories. One group is characterized by large, bulky, rigid molecules; members of this group include d-tubocurarine, vecuronium, atracurium, and pancuronium, all of which are the competitive, nondepolarizing neuromuscular blockers. The other group is characterized by long, slender, flexible molecules that allow free bond rotation. Decamethonium and succinylcholine are in this group; these agents represent member of the depolarizing NMB agents. The dichotomy in basic structural arrangement of competitive, nondepolarizing and depolarizing agents has been offered as a partial explanation for dissimilar effects evoked by interaction of these agents with the nicotinic cholinergic receptor. Among other requirements, receptors contain two anionic (negatively charged) binding sites separated by set distances. These sites are essential for electrostatic bonding of the cationic (positively charged) nitrogen moiety of ACh (and exogenous chemicals) to the receptors (Brejc et al., 2001). All NMB agents, are quaternary ammonium compounds, containing the cationic nitrogen regions necessary to interact at the ACh binding site. Despite the variety of chemical structures represented among the modern nondepolarizing NMB agents, a maximum bond distance between the two quaternary groups present in any of these molecules is typically fixed at 1.0 ± 0.1 nm. Depolarizing NMB agents that vary in length and have free bond rotation may have a distance between quaternary ammonium groups up to a maximum bond distance of 1.45 nm (Hibbs and Zambon, 2011). Occupation of negatively charged binding sites on the receptor by the neurotransmitter, ACh activates influx of Na+ and flux of K+ along their respective concentration gradients, resulting in membrane excitation. Occupation of these sites by the molecularly rigid competitive agents stabilizes the receptor so that the membrane channel is not activated. Depolarizing agents initially act similarly to ACh. Because of their flexible structure, they allow initial channel activation and ion flow but for some reason result in a persistent interruption in ion flow through the receptor so that additional changes in electrical potential are not achieved. The NMJ is quite susceptible to alteration by selective pharmacological agents. Various drugs, toxins, electrolytes, and other agents alter in different manners the synthesis, storage, release, receptor interactions, and catabolism of ACh. Several important factors affecting cholinergic transmission are outlined in Figure 10.1. Hemicholinium is a choline-transport inhibitor that interferes with choline reuptake into cholinergic neurons; although this agent has no current clinical application, it is used widely in research application (Inazu et al., 2013). Acetylcholine synthesis is prevented by lack of choline, classified as an essential nutrient in the B vitamin complex family (Ferguson and Blakely, 2004). Existing vesicular stores of ACh are exhausted upon nerve stimulation, and a gradual weakening and eventual paralysis will result without reuptake and synthesis. Nerve conduction is affected by only a few substances. Local anesthetics in immediate contact with the motor nerve axon act to stabilize the nerve by inactivating both Na+ and K+ channels so that axonal action potential propagation is halted. The puffer fish poison tetrodotoxin and the shellfish poison saxitoxin decrease the permeability of excitable membranes to Na+ (but not K+); thus axonal action potentials are not generated and paralysis results. These toxins do not cause an initial depolarization of nerves; they act noncompetitively, are approximately 100,000 times more potent than cocaine or procaine, and are frequently used in research. Clinical cases of fatal food poisoning have also been attributed to ingestion of these substances from their natural source (Cusick and Sayler, 2013). Botulinum toxin is a potent substance (lethal dose for a mouse is 4 × 107 molecules) produced by Clostridium botulinum. It is ingested rarely by humans, more often in cattle, horses, poultry and waterfowl and often is fatal (Johnson et al., 2010). It acts within the motor axon to prevent ACh release into the NMJ by interfering with fusion of cholinergic synaptic vesicles with the plasma membrane (Dressler et al., 2005). Magnesium ions (Mg++) interfere with release of ACh from the nerve terminal by competing for the transport mechanisms responsible for mobilization of Ca++ into the nerve. Magnesium uncouples the excitation–secretion coupling process. An insufficient concentration of Ca++ produces similar effects. Magnesium also acts postsynaptically to decrease the effectiveness of ACh to activate receptors. Aminoglycoside antibiotics (i.e., neomycin–streptomycin group) inhibit release of ACh from motor nerves by decreasing availability of Ca++ at superficial membrane binding sites of the axonal terminal, thereby inhibiting the excitation–secretion coupling process. These antibiotics also reduce sensitivity of the postsynaptic membrane to ACh (Adams, 1984). Cholinesterase inhibitors (see Chapter 8) decrease the hydrolytic activity of AChE and pseudocholinesterase (Taylor, 1991; Hibbs and Zambon, 2011). ACh rapidly accumulates at receptor sites. Muscle fasciculations, spasms, convulsions, and eventually apnea occur after overdosage with cholinesterase inhibitors. The pharmacological effects of clinically useful NMB drugs are a result of their direct alteration of the ability of ACh to activate postsynaptic nicotinic cholinergic receptors (Hibbs and Zambon, 2011). Nondepolarizing NMB agents compete with ACh for available nicotinic cholinergic receptors at the neuromuscular postsynaptic membrane and, once occupying these receptors, prevent the ability of Ach to produce a motor response. The historical prototype of this group of drugs is d-tubocurarine (tubocurarine chloride, USP, Tubarine), a naturally occurring monoquaternary alkaloid obtained from the bark of the South American plant Chondrodendron tomentosum. Today d-tubocurarine is not used clinically and has long been replaced with similarly acting agents, including atracurium, cisatracurium, pancuronium, vecuronium, rocuronium, mivacurium, doxacurium, and, most recently, gantacurium. Pharmacological characteristics of representative NMB agents in modern use are summarized in Table 10.1. Table 10.1 Characteristics of neuromuscular blocking agents Species indicated are canine (C), feline (F) and equine (E). Ultra refined experimental techniques helped verify the primary site of action of competitive blocking agents (Bowen, 1972; Hubbard and Quastel, 1973). Although d-tubocurarine binds to cholinergic receptors in the same region as ACh, and appears to have the same or similar affinity as ACh for cholinergic receptors, d-tubocurarine does not exhibit receptor-activating properties, it has no depolarizing activity, and therefore does not cause a motor end-plate potential. Moreover, the d-tubocurarine–receptor interaction renders affected receptors unavailable for interaction with ACh. ACh-induced end-plate potentials are reduced to subthreshold levels or abolished in curarized muscles. In the absence of induced end-plate potentials and subsequent muscle action potentials, the muscle relaxes and is, in fact, paralyzed. Based on competitive interaction between nondepolarizing agents and ACh, cholinesterase inhibitors were found to be effective in antagonizing the effects of these blocking agents (Taylor, 2011). Cholinesterase inhibitors prevent the enzymatic catabolism of ACh. More ACh is available for interaction with cholinergic receptors and thereby decreases effectiveness of competitive blocking agents. This relationship has been exploited clinically in successful efforts to terminate the effects of nondepolarizing agents. However, cholinesterase inhibitors do not antagonize effects of the other class of neuromuscular blockers, the depolarizing drugs. Nondepolarizing NMB agents are classified as pachycurares, or bulky molecules having their amine functions incorporated into rigid ring structures. Two groups of synthetic pachycurares contain the drugs in common use in medicine today: the aminosteroids and the benzylisoquinoliniums. The aminosteroids maintain their interonium distance by an androstane skeleton while the benzylisoquinoliniums maintain this atomic distance by linear diester-containing chains (Lee, 2001). Atracurium is a bis-benzyltetrahydroisoquinolinium with isoquinolinium nitrogens connected by a diester-containing hydrocarbon chain. Its action is intermediate with a dose-dependent onset of action of approximately 5 minutes and duration of approximately 30 minutes in dogs (Jones, 1983). Repeated doses are generally not cumulative, so longer-term maintenance can be achieved via a constant-rate infusion (Playfor et al., 2000). Atracurium undergoes ester hydrolysis, Hofmann elimination reaction, and likely other nonhepatic routes for biotransformation, making the drug appropriate for use in hepatic or patients with renal insufficiency. In a Hofmann elimination reaction, a quaternary ammonium group is converted to a tertiary amine by cleavage of a carbon–nitrogen bond. This process does not require enzymatic activity. Hofmann elimination is both a pH and temperature-sensitive reaction in which higher pH and temperatures favor drug breakdown. Clinical consequences of this are important in that patient acidemia and hypothermia may hinder drug breakdown (Playfor et al., 2000). Additionally, the drug should be kept refrigerated and is supplied at a pH of 3.25–3.65 to slow degradation. The marketed atracurium product has ten isomers. These isomers are separated into three geometric isomer groups that have been designated cis–cis, cis–trans, and trans–trans according to their configuration about the tetrahydroisoquinoline ring system (Wastila et al., 1996). Cisatracurium is the 1R cis-1’R cis isomer of atracurium and represents approximately 15% of the marketed atracurium product’s weight yet produces roughly 50% of atracurium’s neuromuscular blocking activity. Cisatracurium (Nimbex) is available as a stand-alone product separate from atracurium. Cisatracurium is metabolized by Hofmann elimination entirely. The presence of portosystemic shunt and hepatic insufficiency did not affect the rate of onset or duration of action of cisatracurium in dogs receiving 0.1 mg/kg IV followed by repeat doses of 0.03 mg/kg IV (Adams et al., 2006). In general, cisatracurium has a similar duration and clinical effects to atracurium. Following intravenous injection, atracurium spontaneously decomposes into laudanosine and a quaternary monoacrylate. Laudanosine in high quantities is a CNS stimulant and may result in seizures, hypotension, and tachycardia (Chapple et al., 1987). Laudanosine, unlike atracurium, is dependent upon hepatic clearance, so theoretically laudanosine plasma concentrations may become elevated in patients with hepatic dysfunction. Practically, laudanosine-induced toxicity is unlikely in clinical patients unless atracurium is used in large doses and/or for prolonged periods (Chapple et al., 1987). Nonetheless, another aminosteroid may be selected to avoid this remote issue entirely. d-Tubocurarine, the prototypical benzoisoquinolone NMB agent, is associated with histamine release and resulting hypotension. Although atracurium has the potential to result in histamine release, this requires several times the ED95 dose (effective dose producing a 95% reduction in twitch height) before appreciable amounts of histamine are released, making problems such as hypotension and tachycardia rarely observed in clinical cases (Scott et al., 1986; Hackett et al., 1989). In isoflurane-anesthetized dogs, 0.2 mg/kg IV atracurium did not result in significant changes to intraocular pressure, mean arterial pressure, heart rate, or central venous pressure (McMurphy et al., 2004). A notable exception to atracurium and cisatracurium’s minimal cardiovascular profile may be in dogs with X-linked muscular dystrophy. Affected golden retrievers in one retrospective study that received 0.1 mg/kg cisatracurium had marked increases in heart rate (115 ± 64%) and blood pressure (33.5 ± 31%) that lasted 10 and 30 minutes, respectively (Staffieri et al., 2011). Atracurium has been widely used with positive clinical success across a variety of species including, but not limited to, dogs, cats, and horses. In horses and dogs, the use of atracurium in conjunction with aminoglycoside antibiotics, specifically gentamycin, has been shown to augment neuromuscular blockade; however, this effect appears minimal and has not been reported to impact recovery quality (Hildebrand and Hill, 1994; Martinez et al., 1996). Atracurium has also been investigated for use in a urethral flushing solution in cats and dogs to help facilitate manual bladder expression in patients with spinal cord injuries and in obstructive urethral plugs (Galluzzi et al., 2012; Galluzzi et al., 2015). Two additional benzylisoquinolinium compounds, mivacurium and doxacurium, are reported in the veterinary literature but are infrequently used in clinical practice today. Mivacurium (Mivacron) is a short-acting NMB agent in humans (15–20 minutes’ duration); however, this duration of effect appears markedly prolonged in dogs. Although mivacurium resulted in minimal hemodynamic changes, in dogs administered 0.05 mg/kg IV it appeared to have a long half-life and slow clearance under halothane anesthesia with duration of effect up to 151.0 ± 38.50 minutes (Smith et al., 1999a, 1999b). Clinical observations in cats suggest that mivacurium has a shorter duration of action compared to that in dogs. Mivacurium is unique amongst the benzylisoquinolinium compounds in that it is metabolized by plasma cholinesterases to a monoester and this attribute, as a result of interspecies differences in plasma cholinesterases, may explain its variable duration of action between species. At the time of writing, mivacurium is not currently available within the United States. Doxacurium (Nuromax) has a slow onset of action and long duration (>50 minute) of effect in man. Doxacurium may result in an increased propensity for histamine release and cardiovascular side effects when compared to other benzylisoquinolinium compounds. Work in dogs would suggest that 2.1 μg/kg IV approximates an ED50 while 3.5 μg/kg IV approximates an ED95 with duration of action at 108 ± 31 minutes (Martinez et al., 1996). Due to increased cost, minimal reported advantage, and lack of availability and clinical experience, atracurium is typically selected over other available benzylisoquinolinium compound for veterinary patients. Pancuronium (Pavulon) was the first aminosteroid to be introduced as a NMB agent. The drug has a dose-dependent onset of approximately 5 minutes and long duration of action, up to 60 minutes in the dog (Gleed and Jones, 1982). Because repeated doses have a cumulative effect, constant-rate infusions are avoided. A large proportion of pancuronium is excreted by the kidney. The remainder is metabolized via the liver, making the duration of action increased in patients with renal and hepatic dysfunction. In addition to exerting its effect on postsynaptic nicotinic receptors at the NMJ, pancuronium also has a vagolytic effect by blocking cardiac muscarinic action, which may result in an increase in heart rate. Decreases to systemic vascular resistance, pulmonary vascular resistance, and coronary and renal artery dilation via prostaglandin I2 release have also been noted in the dog (Hackett et al., 1989; Sai, 1998). In dogs, cats, pigs, and horses doses between 0.06 and 0.12 mg/kg IV have been reported (Gleed and Jones, 1982; Hildebrand et al., 1989; Miller et al., 1978; Veres-Nyéki et al., 2012). Pancuronium is stable at room temperature for 6 months. Removal of a single positively charged methyl group from pancuronium creates vecuronium, an aminosteroid NMB agent that is essentially devoid of cardiovascular effects (Morris et al., 1983; Jones, 1985b). This molecular modification has several other important clinical implications, including molecular instability in solution which necessitates vecuronium be kept as a lyophilized powder and only reconstituted immediately prior to administration with stability of only 24 hours once reconstituted. Additionally, vecuronium has a shorter duration of action than pancuronium and an increase in lipid solubility, which results in greater biliary elimination than with pancuronium (Hill et al., 1994). Vecuronium has a dose-dependent onset of action of approximately 5 minutes and generally an intermediate duration of action of approximately 30 minutes, making its clinical utility similar to atracurium. Vecuronium undergoes both hepatic metabolism, biliary clearance from the liver as parent compound, and about 25% undergoes renal elimination. Patients with renal or hepatic insufficiency may experience prolonged recovery if increased doses of vecuronium are administered. Dogs with diabetes mellitus have been shown to have a shorter duration of effect from vecuronium based upon both tactile train-of-four (TOF) and electromyography (Clark et al., 2012). The potency and duration of vecuronium does not, however, appear to be altered in dogs with autosomal recessive centronuclear myopathy (Martin-Flores et al., 2015b). In horses, it appears that a dose greater than 0.1 mg/kg IV would be needed for complete neuromuscular blockade and that this effect would have a uniquely long duration in this species, lasting over 120 minutes (Martin-Flores et al., 2012a, 2012b). Additionally, one case report documents that a dosage of 0.5 mg/kg IV edrophonium failed to completely reverse prolonged vecuronium-induced neuromuscular blockade in anesthetized dog as judged by peripheral nerve stimulation (Martin-Flores et al., 2011). Rocuronium bromide (Zemuron), another member of the aminosteroid NMB agent family, lacks the acetyl ester that is found in the steroid nucleus of pancuronium and vecuronium and is less potent but similar in molecular weight to vecuronium. Rocuronium has a more rapid onset compared with atracurium and vecuronium, but similar duration of action (Carson et al., 1990; Gyermek et al., 2002). Additionally, rocuronium appears to be largely devoid of cardiovascular side effects, including no significant histamine release (Hudson et al., 1998). The drug is eliminated primarily via hepatic clearance while a small fraction is eliminated via the kidney. Detectable levels of rocuronium metabolites are not found. Rocuronium is stable for 60 days at room temperature. Rocuronium at clinically relevant concentrations may also bias the accuracy of lithium dilution cardiac output monitoring by interacting with the LiDCO sensor (Ambrisko et al., 2013). In cats, a dosage of 0.6 mg/kg IV rocuronium had an onset time of 46 ± 11 seconds, produced no change to subject heart rate, and took 20.7 ± 5.4 minutes for TOF ratios to return to 0.9, a level consistent with full recovery from neuromuscular blockade (Auer and Mosing, 2006; McGrath and Hunter, 2006). Sixty seconds after rocuronium administration in cats, paralysis of the internal laryngeal muscles and conditions suitable for endotracheal intubation occurred that were comparable to topical lidocaine (Moreno-Sala et al., 2013). In dogs, rocuronium at 0.3 mg/kg and 0.6 mg/kg IV resulted in an onset of neuromuscular blockade in 2 ± 0.9 minutes and 1.1 ± 0.6 minutes with total recovery occurring at 23.8 ± 6.6 minutes and 31.9 ± 6.5 minutes respectively (Auer, 2007). Following a loading dose of 0.5 mg/kg IV, rocuronium was found to be suitable for constant-rate infusion at 0.2 mg/kg/h for up to 146 minutes (Alderson et al., 2007). Doses of rocuronium as low as 0.03–0.075 mg/kg IV in isoflurane-anesthetized dogs resulted in a centralized globe position and acceptable conditions for ophthalmic procedures (Briganti et al., 2015). In horses undergoing ophthalmic surgery, a dose of 0.3 mg/kg IV rocuronium produced effective neuromuscular blockade in 2.3 ± 2 minutes with a central globe position in 31 ± 2.8 seconds and a clinical duration of 32 ± 18.6 minutes in all 20 horses studied (Auer and Moens, 2011). Gantacurium represents a new class of nondepolarizing NMB agents called asymmetric mixed-onium chlorofumarates, which are structurally distinct from the traditional aminosteroids and benzylisoquinolinium compounds. Gantacurium has an ultrashort duration of action and is degraded in plasma by pH sensitive chemical hydrolysis and inactivation by cysteine adduction. It does not undergo Hofmann elimination. In dogs anesthetized with thiopental, nitrous oxide, and isoflurane, the ED95 was 0.06 mg/kg IV with an onset time of 107 seconds and duration of action of 5.2 minutes (Heerdt et al., 2004). In cats following dexmedetomidine and propofol, IV gantacurium at 0.5 mg/kg abolished laryngospasm in 100% of cats and induced apnea for 3 ± 1.5 minutes (Martin-Flores et al., 2015a). At clinical doses, cats and dogs do not appear to have appreciable cardiovascular effects; however, in humans, transient cardiovascular side effects were observed at doses beginning at three times the ED95 and were consistent with histamine release (Belmont et al., 2004). Recovery is accelerated with AChE inhibitors such as edrophonium. In humans, exogenous administration of cysteine can also accelerate the antagonism of gantacurium-induced neuromuscular blockade. Gantacurium is not stable in aqueous solution and, like vecuronium, is provided as a lyophilized powder that is reconstituted prior to administration.
Neuromuscular Blocking Agents
Development
Impulse Transmission at the Somatic Neuromuscular Junction
Physiological and Anatomic Considerations
The Nicotinic Receptor and Structure–Activity Relationships
Pharmacological Considerations
Postjunctional Mechanisms of Neuromuscular Blockade
Competitive Nondepolarizing Agents
Drug
Onset
Duration
Elimination
Dosing
Constant rate infusion
Depolarizing agents
Succinylcholine
1–2 min
6–10 min
Hydrolysis by plasma cholinesterase
0.3–0.4 mg/kg (C) 0.1–0.2 mg/kg (F) 0.12–0.15 mg/kg (E)
Competitive nondepolarizing agents
Atracurium
5 min (C)
30–40 min (C)
Ester hydrolysis, Hofmann elimination
0.1–0.2 mg/kg (C) 0.1–0.25 mg/kg (F) 0.07–0.15 mg/kg (E)
0.18–0.36 mg/kg/h (C) 0.17 mg/kg/h (E)
Cisatracurium
5 min (C)
30–40 min (C)
Hofmann elimination
0.075–0.3 mg/kg (C) 0.05–0.3 mg/kg (F)
Doxacurium
40 min (C)
100–120 min (C)
Renal and biliary elimination of unchanged drug
3.5 μg/kg (C)
Vecuronium
5 min (C)
30–40 min (C)
Hepatic metabolism biliary and renal excretion
0.1 mg/kg (C) 0.025–0.1 mg/kg (F) 0.1 mg/kg (E)
Rocuronium
1–2 min (C)
20–30 min (C)
Hepatic uptake and Biliary excretion
0.1–0.6 mg/kg (C) 0.1–0.6 mg/kg (F) 0.3–0.6 mg/kg (E)
0.2 mg/kg/h (C)
Pancuronium
5 min (C)
30–60 min (C)
Hepatic metabolism and renal elimination of active metabolites
0.07–0.1 mg/kg (C) 0.06–0.1 mg/kg (F) 0.12 mg/kg (E)
Gantacurium
1–2 min (C)
5 min (C)
Ester hydrolysis, cysteine adduction
0.06 mg/kg (C) 0.06 mg/kg (F)
Benzylisoquinolinium Compounds
Aminosteroid Neuromuscular Blocking Agents
Asymmetric Mixed-onium Chlorofumarates
Trisquaternary Ether Neuromuscular Blocking Agents
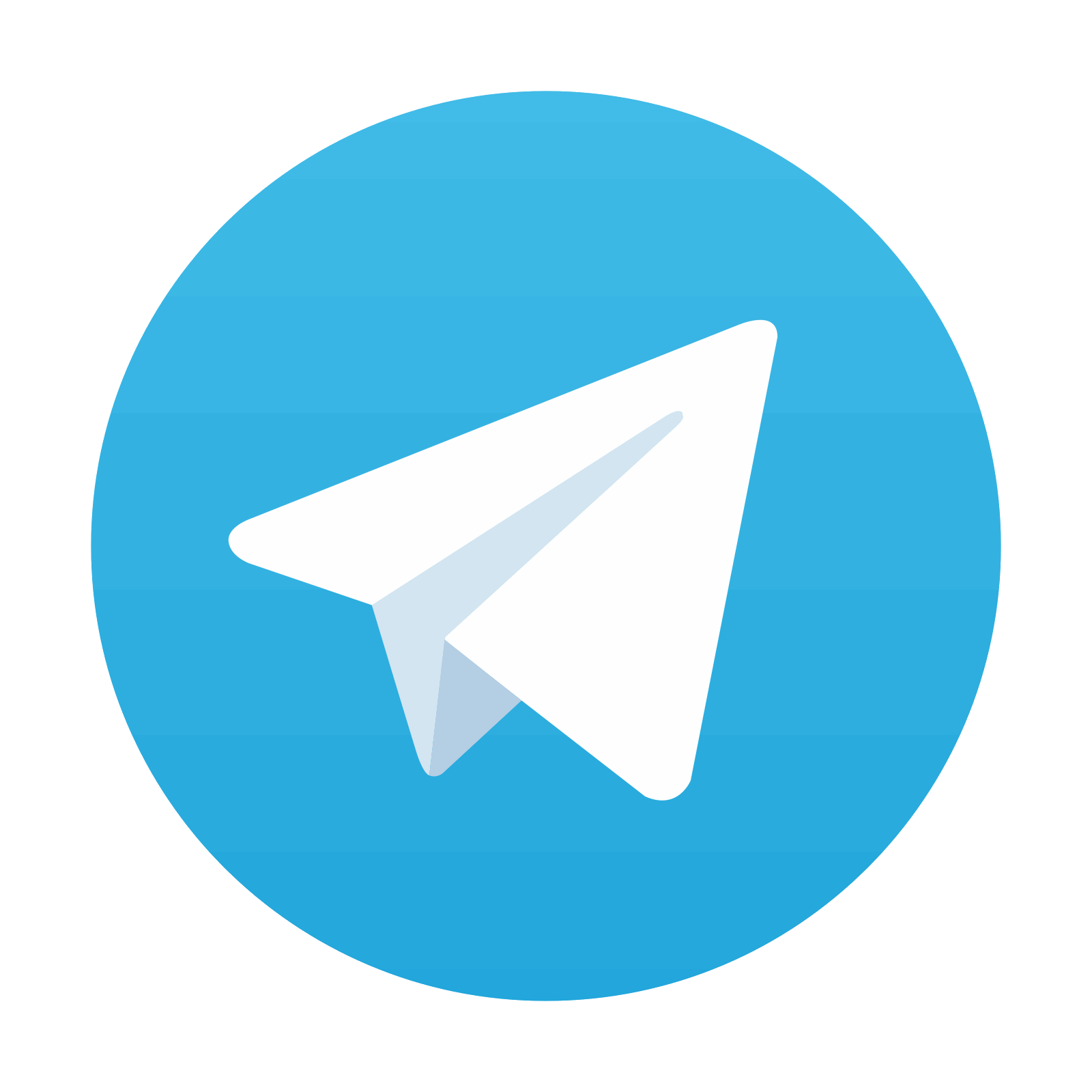
Stay updated, free articles. Join our Telegram channel

Full access? Get Clinical Tree
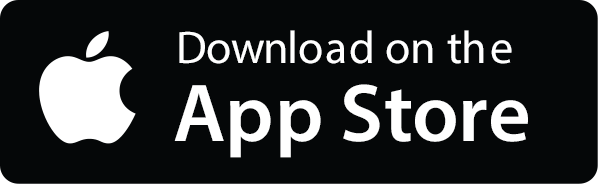
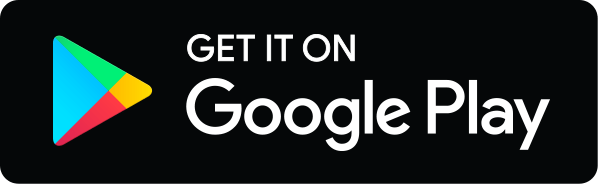