17 Mark G. Papich Treatment of seizure disorders first requires that an accurate diagnosis is made and underlying problems ruled out, such as metabolic disorders, neoplasia, congenital disorders, and intoxication. If a diagnosis of idiopathic epilepsy is made, treatment is usually initiated with one of the maintenance anticonvulsants described in this chapter. Fortunately, excellent current guidelines are available from experts that help guide drug selection (Podell et al., 2016; Bhatti et al., 2015). An evidence-based analysis of anticonvulsant studies in animals provides veterinarians information to make decisions about selection of drugs (Charalambous et al., 2014). Occasionally, animals are presented to veterinary hospitals with continuous seizures – status epilepticus. These animals require prompt treatment with one of the rapidly acting anticonvulsants. The methods used to classify and diagnosis seizure disorders will not be discussed in this chapter. One should rely on a reputable internal medicine textbook, review paper, or reference that specifically addresses seizure disorders in animals (e.g., Podell, 2013; Muñana, 2013). In recent Task Force and specialty Consensus Statement Reports (Podell et al., 2016; Bhatti et al., 2015) these drugs are referred to as antiepileptic drugs (AED). Here, we will use the term anticonvulsant drugs, which for the purpose of this chapter will be considered interchangeable terms. Anticonvulsant drugs act to limit either the initialization or spread of the seizure focus in the central nervous system. These drugs act to suppress nerve conduction, stabilize neurons, or enhance and potentiate the action of the inhibitory neurotransmitter γ-aminobutyric acid (GABA). For a few drugs, the exact mechanism of action is still unknown. Some drugs, such as the barbiturates, are used for other indications as well. Some barbiturates are better at providing an anticonvulsant effect, and others are better at producing anesthesia. An anticonvulsant is distinguished from an anesthetic by an action that suppresses seizure activity, without producing unconsciousness. Table 17.1 lists anticonvulsant drugs used in veterinary medicine. Some are no longer in use, or are rarely used. Table 17.1 Anticonvulsants that have been used in veterinary medicine The application of pharmacokinetic principles to treatment of epilepsy in animals has been a major contribution to veterinary neurology. Therefore, the focus of many research publications has been on the pharmacokinetics of the anticonvulsant medications. The pharmacokinetics of several anticonvulsants in dogs, with comparison to humans, was reported by Frey and Löscher (1985), and other papers over the years have followed this pattern with the intent of examining the pharmacokinetics of human-labeled drugs in animals for the purpose of evaluating their potential for veterinary anticonvulsant use. Other relevant papers will be discussed in this chapter among individual drugs. In the paper by Frey and Löscher (1985), they reported significant differences for some drugs, with generally much more rapid clearance in dogs than humans. Protein binding was generally similar for drugs in dogs and people, with the exception of valproate and phenytoin. The most recent consensus papers and task force reports by experts on this topic (Podell et al., 2016; Potschka et al., 2015; Bhatti et al., 2015) have helped tremendously to guide veterinarians on the best drugs to consider for initial use, the dosages, adverse effects, and evidence to support the clinical use. Drugs most often listed in these guidelines for seizure management include: phenobarbital, bromide, primidone, imepitoin, levetiracetam, and zonisamide. Imepitoin is not approved in the United States, but is available in other countries. Phenobarbital is the most widely used anticonvulsant drug in small animals. Although it is classified as a barbiturate (Figure 17.1) and shares properties with barbiturates discussed in Chapter 12, phenobarbital is unique in its ability to produce an anticonvulsant effect at doses below those that produce anesthesia. Figure 17.1 Structure of common anticonvulsant drugs used in veterinary medicine. Phenobarbital increases the seizure threshold and decreases the electrical activity of the seizure focus by potentiating the action of the neurotransmitter GABA. Potentiating the GABAA subunit increases chloride conductance in neurons, stabilizes electrical activity, and raises the potential necessary for depolarization. Barbiturates also decrease the influx of calcium into nerve cells and thereby decrease release of neurotransmitters. This property may be more important at high phenobarbital levels than at the concentrations that produce an anticonvulsant effect. The pharmacokinetics of phenobarbital have been studied in a variety of animals (Frey and Löscher, 1985). Protein binding in serum is 24–30% in sheep, 34–52% in goats, 31% in horses, 19–28% in rabbits, 10–25% in pigs, and 4–23% in cows (Bailey, 1992). This compares with a value of 30–38% in humans. Phenobarbital is well absorbed with systemic availability that is almost complete in monogastric animals (Pedersoli et al., 1987). The volume of distribution is large and is similar to total body water at 600–700 ml/kg. The high lipophilicity produces penetration across lipid membranes, including the blood–brain barrier of the central nervous system (CNS). Phenobarbital clearance is low in dogs (0.09–0.1 ml/kg/min) (Pedersoli et al., 1987), but it is highly metabolized and relies on hepatic cytochrome P450 enzymes for biotransformation. In people the half-life is in the range of 70–100 hours. In dogs the range has been from 37 to 75 hours (average 53 hours) (Ravis et al., 1984), 89 ± 20 hours (Ravis et al., 1989), and 92.6 (± 24) hours (Pedersoli et al., 1987). In a study that examined variations during the dosing interval in epileptics, the range in half-life among dogs was 20–140 hours, with a mean of 65 hours (Levitski and Trepanier, 2000). As demonstrated from these studies, the half-lives can vary considerably among animals, which may explain a range of plasma concentrations despite similar doses among dogs. This degree of variability supports individualized dosing based on therapeutic drug monitoring. Changes in half-life and clearance that occur during multiple dosing is caused by autoinduction of hepatic metabolism (Ravis et al., 1989; Hojo et al., 2002), and may also be influenced by diet (Maguire et al., 2000). With multiple dosing, the half-life decreased from 89 hours (± 19.6) on day 1 to 47 hours (± 11) at day 90. After multiple dosings produce autoinduction of hepatic enzymes, clearance almost doubled in dogs (Ravis et al., 1989). After multiple oral treatments with phenobarbital, the liver cytochrome P450 content was twofold higher and plasma concentrations lower, compared to untreated dogs (Hojo et al., 2002). The activity of CYP1A, 2C, and 3A were increased by two- to fourfold compared to untreated dogs. In cats the half-life ranges from 35 to 56 hours (average 43 hours) and from 59 to 76 hours, depending on the study (Cochrane et al., 1990a, 1990b). In horses the half-life is approximately 18–19 hours; however, one study showed a half-life of 24 hours after a single dose but 11.2 hours after multiple doses (Knox et al., 1992; Duran et al., 1987; Ravis et al., 1987). In foals the mean half-life was 13 hours (Spehar et al., 1984). These values for horses indicate a more rapid clearance in horses than in other species. In birds the half-life is short, which makes it difficult to maintain effective concentrations. Despite high doses in parrots, the half-life was only 1.4–1.7 hours, with low peak concentrations (Powers and Papich, 2011). Phenobarbital is a well-known inducer of microsomal cytochrome P450 (CYP450) enzymes (microsomal enzymes) that metabolize drugs (Hojo et al., 2002). Phenobarbital is an inducer of canine CYP2B11, CYP3A12, CYP2C21/2C41, and possibly others (Martinez et al., 2013). Increased amounts of CYP450 enzymes have been documented and are found in the endoplasmic reticulum of hepatocytes. Induction of microsomal enzymes may cause enhanced biotransformation of other drugs, which can diminish the pharmacological effect for a drug that is administered concurrently (e.g., digoxin, corticosteroids, phenylbutazone, some anesthetics, and antipyrine). When phenobarbital was administered with another anticonvulsant to dogs, levetiracetam, the clearance and half-life of levetiracetam was significantly altered, producing a shorter half-life and faster clearance in dogs (Moore et al., 2011). The authors of the study concluded that concurrent phenobarbital administration significantly alters the pharmacokinetics of levetiracetam in dogs, indicating that dosage adjustments might be necessary when the drug is administered with phenobarbital. Phenobarbital will also increase the clearance of zonisamide by at least 50%, requiring an adjustment of the dose of zonisamide if it is administered with phenobarbital (Orito et al., 2008). (Zonisamide and levetiracetam are discussed later in this chapter.) It was also observed that if a canine patient has been receiving maintenance therapy with phenobarbital, the concentration of diazepam and metabolites were lower after intravenous and rectal administration compared to diazepam administration prior to phenobarbital treatment. Therefore, it may require higher doses of diazepam in some dogs to control periodic seizures because of increased clearance caused by enzyme induction (Wagner et al., 1998). Enzyme induction will also increase phenobarbital’s own metabolism. In one study (Maguire et al., 2000), the half-life at the beginning of the study was 47–49 hours in dogs. But, after chronic treatment for 2 months, the phenobarbital half-life decreased to 24–33 hours. In another study, autoinduction of enzymes significantly lowered the phenobarbital plasma drug concentration in dogs compared to the expected level that would ordinarily occur from multiple dosing (Hojo et al., 2002). Other drugs that are microsomal enzyme inhibitors (CYP450 inhibitors) may inhibit the metabolism of phenobarbital and cause toxicity if the two drugs are administered concurrently. Chloramphenicol and ketoconazole are examples of these CYP450 enzyme inhibitors (Martinez et al., 2013). Side effects that one may expect include sedation, polyphagia (with associated weight gain), polyuria/ polydipsia, and mild behavior changes. These may subside somewhat after the first few weeks of treatment because both pharmacokinetic and pharmacodynamic tolerance develops with chronic therapy. Pharmacokinetic tolerance is caused by enhanced metabolism owing to induction of the hepatic enzymes (i.e., phenobarbital increases its own metabolism) with chronic dosing that was discussed above. Pharmacodynamic tolerance is determined by the sensitivity of receptors. Elevated hepatic enzymes have been observed after chronic therapy in healthy animals (ALT, alanine aminotransferase; ALP, alkaline phosphatase) without an association with hepatic injury (Aitken et al., 2003; Gieger et al., 2000; Müller et al., 2000a; Chauvet et al., 1995). The highest elevations occur with serum ALP. Elevations in hepatic enzymes that are not associated with liver pathology are reported to be caused by induction of the enzymes by the drug and are expected to return to normal 3–5 weeks after the drug is discontinued (Gieger et al., 2000). In one study of 95 epileptic dogs controlled with phenobarbital, the increases in liver enzymes were related to the phenobarbital plasma concentration (Aitken et al., 2003). Dogs with the highest levels of phenobarbital also had the highest ALT increases. Hepatotoxicosis also is possible from phenobarbital. It has been described as both an intrinsic and idiosyncratic hepatic injury. In clinical reports, most affected dogs had high serum phenobarbital concentrations (>40 μg/ml) (Dayrell-Hart et al., 1991). Animals with phenobarbital-induced hepatotoxicosis had bilirubin increases that were disproportionately elevated in comparison to the ALP. There also may be elevations in bile acids or other signs of hepatic disease. Liver lesions include chronic fibrosis with nodular regeneration, biliary hyperplasia, necrosis, and cirrhosis (March et al., 2004). Animals receiving high doses of phenobarbital to control seizures should be examined periodically because they may be at a higher risk of developing liver disease. Although hepatic injury has been an important concern associated with administration of phenobarbital to dogs, this has not been a documented problem in cats. As a consequence of phenobarbital-induced liver disease, the skin also may be affected. This has been described as superficial necrolytic dermatitis, more commonly known as hepatocutaneous syndrome. The hepatocutaneous syndrome has been associated with phenobarbital administration in some dogs (March et al., 2004). Prolonged phenobarbital exposure and high plasma drug levels may contribute to this disease in dogs. Anemia, thrombocytopenia, and neutropenia have been described as a result of phenobarbital administration (Jacobs et al., 1998). It is rare, and probably idiosyncratic, but should be considered in a patient that shows signs of a blood disorder in association with phenobarbital treatment. Phenobarbital administration may alter thyroid hormone levels (Gieger et al., 2000; Kantrowitz et al., 1999). After multiple doses, it may decrease thyroxine (T4) and free T4 in dogs, but not T3 (Kantrowitz et al., 1999). In one study, T3 was increased slightly (Müller et al., 2000b). Thyroid stimulating hormone (TSH) has been mildly elevated in some studies, but not others (Müller et al., 2000b; Gieger et al., 2000). Thyroid hormones and TSH return to normal within 4 weeks after discontinuing phenobarbital treatment. The mechanism for the effect on thyroid hormone is probably via an increase in T4 metabolism induced by phenobarbital (Kantrowitz et al., 1999; Gieger et al., 2000). In experimental animals and humans phenobarbital accelerates steroid metabolism. This may decrease the therapeutic effects of corticosteroid administration. However, there is no evidence that phenobarbital treatment induces adrenal disease in dogs. The effect of phenobarbital administration to dogs on adrenal cortex diagnostic tests has been examined (Dyer et al., 1994; Chauvet et al., 1995). Phenobarbital administration to dogs did not affect response to exogenous adrenocorticotropic hormone (ACTH) or the levels of endogenous ACTH. Phenobarbital is considered the first drug of choice for long-term treatment of seizure disorders in dogs and cats (Podell et al., 2016). Its efficacy has been estimated to be approximately 60–90%. It also has been used to treat some behavior disorders in dogs and cats. Daily dosages of 4–16 mg/kg/day have been used, but dogs are usually started on 2.5–3 mg/kg q 12 h and adjusted up to 6–8 mg/kg q 12 h, gradually – if necessary. Even though the half-life is long in dogs, most animals are initially treated with phenobarbital on an every-12-hour schedule to decrease side effects and ensure minimal peak–trough fluctuations. In some patients, however, once-a-day dosing may be effective. The typical starting dose for cats is 1.5–2.5 mg/kg, q 12 h, PO (Quesnel et al., 1997; Platt, 2001; Finnerty et al., 2014). In some cats, doses are increased to 2–4 mg/kg, q 12 h, PO. A common dose in cats is 7.5 mg per cat, q 12 h (half of a 15-mg tablet), and the dose may be adjusted by 7.5-mg increments. In horses, the initial doses are 11 mg/kg q 24 h. When chronic therapy has been needed the dose may be increased to 25 mg/kg q 24 hr. Phenobarbital may be injected in animals for the treatment of status epilepticus. One of the consensus documents (Bhatti et al., 2015) recommends a loading dose of 15–20 mg/kg IV, or IM, followed by additional doses in increments of 3–5 mg/kg over 24–48 hours until seizure control is maintained, or therapeutic plasma/serum concentrations are attained. One author recommends that in naïve animals (not previously on anticonvulsant maintenance medication), a loading dose of 12 mg/kg IV should be used, followed by 2–4 mg/kg increments every 20–30 minutes until seizure control is achieved (Muñana, 2013). Phenobarbital infusions also have been administered at the rate of 3–6 mg/dog per hour, IV (Bateman and Parent, 2000). Phenobarbital does not cross the blood–brain barrier as fast as diazepam; therefore, it may take several minutes for an anticonvulsant effect to treat status epilepticus. In severe cases, pentobarbital has been administered to effect, starting with doses of 4–20 mg/kg IV. Pentobarbital is not a good anticonvulsant; its use in this instance is simply to anesthetize the patient until additional therapy can be provided. Typically, phenobarbital tablets are the preferred dose formulation. There is also an injection formulation for IV use, and a liquid elixir. Because the elixir is bitter for many animals, phenobarbital occasionally has been compounded into an oral liquid for administration to small dogs and cats. These compounded formulations have been stable for 115 days. Because cats may be difficult to medicate orally, a compounded transdermal formulation has been prepared in a polymer gel or proprietary compounding base to enhance absorption across the skin and applied to the ears of cats (Gasper et al., 2015; Krull et al., 2014). However, in one study the formulation in a pluronic lecithin organogel (PLO) matrix had inconsistent and low potency and at a dose of 6 mg/kg twice daily produced measurable, but subtherapeutic, plasma concentrations in cats (Krull et al., 2014). Another study using a compounded transdermal formulation showed that effective concentrations can be achieved in cats if a high dose of 9 mg/kg every 12 hours was administered (Gasper et al., 2015).There are no commercial forms available for transdermal application. Consistency of the formulations and long-term safety have not been evaluated. To adjust dose, monitor compliance, and assess toxicity it is the usual practice to monitor plasma or serum phenobarbital concentrations regularly during therapy. Assays are available at most diagnostic laboratories. This can be done initially after the first 2 weeks of starting treatment and then every 6–12 months, or as needed to assess treatment. The recommended plasma/serum therapeutic concentration for dogs is 15–40 μg/ml (65–180 mmol/l). Some neurologists list this range as 20–45 μg/ml and 15–45 μg/ml, and a consensus panel listed 25–35 μg/ml (Bhatti et al., 2015) and 15–35 μg/ml (Podell et al., 2016) as the effective range. If dogs are also receiving bromide (see Section Bromide (Potassium and Sodium Bromide)), phenobarbital concentrations in the range of 10–36 μg/ml have been reported as therapeutic (Trepanier et al., 1998). For cats, the plasma concentrations can be variable, but the optimum range has been cited as 23–30 μg/ml (Quesnel et al., 1997). In another study in cats, seizure control was achieved in 93% of cats when concentrations were between 15 and 45 μg/ml (Finnerty et al., 2014). In the cited study by Quesnel et al. (1997), cats were more unstable than dogs, possibly because of more serious CNS disease and required more frequent monitoring. Because phenobarbital has such a long half-life, fluctuations between plasma peaks and troughs are minimized when it is administered on an every-12-hour schedule. Therefore, the timing of samples usually makes no difference when a sample is collected during a 12-hour schedule for the assessment of plasma phenobarbital concentrations (Levitski and Trepanier, 2000). However, in one study the trough concentrations were lower than at other times during the day when dogs received greater than 10 mg/kg per day, and they recommended always monitoring each individual patient at the same time after dosing to obtain consistent results (Monteiro et al., 2009). Primidone (Mylepsin, Mysoline) is a barbiturate derivative metabolized to phenobarbital and phenylethylmalonamide (PEMA). Primidone and its two major metabolites have anticonvulsant activity but at least 85% of the pharmacological activity is derived from phenobarbital. Primidone has been around for a long time and was once more widely used in dogs (but rarely in cats). But, since the 1980s, there has been little new information generated for primidone and its use has declined. In addition to the differences in potency, PEMA and primidone do not persist in the plasma for as long as phenobarbital, which raises questions regarding the activity of PEMA and primidone throughout a long dosing interval (Frey et al., 1979). When primidone was administered to dogs, the half-life (mean value) of PEMA was 7.1 hours, the half-life of primidone was 1.85 hours, and the half-life of phenobarbital was 41 hours (Yeary, 1980). After multiple dosing in this study the primidone concentrations decreased, probably caused by accelerated metabolism. Although seizures can be controlled with primidone in dogs (Schwartz-Porsche et al., 1982), primidone has little advantage over phenobarbital. Control of seizures in dogs is correlated with the plasma concentrations of phenobarbital, rather than primidone (Cunningham et al., 1983). In a comparison between primidone and phenobarbital in epileptic dogs (Schwartz-Porsche et al., 1982), there was no significant difference between phenobarbital and primidone with respect to seizure control, and primidone appeared more likely to induce liver injury than phenobarbital. The authors concluded that phenobarbital, rather than primidone, should be the drug of first choice for treatment of canine epilepsy. However, there may be rare cases that respond to primidone when phenobarbital alone has not been effective (1 out of 15 according to Farnbach, 1984). Primidone is more expensive than phenobarbital, but it is not classified as a controlled drug in the USA; therefore, it is not subject to the same degree of regulation as phenobarbital. Most adverse effects and side effects are the same as those listed for phenobarbital; however, primidone administration may be associated with a higher incidence of hepatotoxicity. Hepatic necrosis, fibrosis, and cirrhosis have been associated with chronic use of primidone (Bunch, 1989). Intrahepatic cholestasis has occurred in dogs in which primidone was combined with phenytoin. Manufacturers do not recommend its use in cats; in fact, it is stated on the package insert that its use should be cautioned in cats. However, studies in cats have demonstrated that primidone is probably safe. Initial dosages in dogs are 3–5 mg/kg q 8–12 h, but have been increased up to 12 mg/kg q 8 h. If one is converting a patient from primidone to phenobarbital or vice versa, the conversion is the following: 65 mg phenobarbital = 250 mg primidone. The effects of primidone are associated with the concentrations of phenobarbital; therefore, clinical monitoring should rely on phenobarbital concentrations (see recommended concentrations listed in Section Phenobarbital, Clinical Monitoring). Measurements of PEMA or primidone are not available in most laboratories. Phenytoin (Dilantin, formerly diphenylhydantoin) is one of the most commonly prescribed anticonvulsants in human medicine. In veterinary medicine, its use is rare because of pharmacokinetic differences and susceptibility to adverse effects. It is not a recommended anticonvulsant for dogs or cats. It is used occasionally in horses for muscle disorders or as an antiarrhythmic agent. Phenytoin stabilizes neuronal membranes and limits the spread of neuronal or seizure activity from the focus. It blocks inward movement of Na+ and stabilizes excitable tissue (this drug is also used as a Class I antiarrhythmic agent, see Chapter 22). Phenytoin also decreases Ca+2 inward flow during depolarization, thus, inhibiting Ca+2-dependent release of neurotransmitters (presynaptic). There are important interspecies differences among animals and between animals and humans that cause difficulty in maintaining effective concentrations. Phenytoin is eliminated much more rapidly in dogs than in people (Frey and Löscher, 1985). Because of its rapid elimination, phenytoin is not recommended as an anticonvulsant in small animals. In cats, the elimination is very slow, and toxicity has been a concern. In horses the pharmacokinetics have been described (Soma et al., 2001), but are highly variable among horses. The half-life was approximately 12–13 hours, depending on the route of administration, but the oral absorption ranged from 14 to 85% among horses. Phenytoin is available in 25 mg/ml oral suspension; 30 and 100-mg capsules (sodium salt); and 50 mg/ml injection (sodium salt). For dogs, these formulation sizes result in an impractical dose (several capsules per day) for a large-size dog. Nevertheless, a dose recommended for dogs is 20–35 mg/kg q 8 h and the antiarrhythmic dose is 30 mg/kg q 8 h PO or 10 mg/kg IV over 5 minutes. It should not be used in cats. Phenytoin has been used in horses for tying-up syndrome. This is a use primarily employed on the racetrack in athletic horses. The use is not related to the anticonvulsant property. In horses, at high doses recumbency and excitement have been observed. Sedation in horses may be an initial sign of high plasma phenytoin concentrations. Phenytoin pharmacokinetics are highly variable in horses, particularly the extent of absorption (Soma et al., 2001). Because of this variability it is difficult to maintain consistent plasma concentrations. Monitoring plasma concentrations may be necessary to adjust the dose to maintain an optimum level and prevent adverse effects. Suggested doses for horses are to administer an initial bolus of 20 mg/kg q 12 h PO, for four doses, followed by 10–15 mg/kg q 12 h PO. A single IV dose in horses of 7.5–8.8 mg/kg can be used, followed by oral maintenance doses. Therapeutic drug monitoring can be performed; however, therapeutic concentrations have not been established for dogs and cats. Effective plasma concentrations listed for people are between 5 and 20 μg/ml. In the absence of other data, an average peak concentration of 15 μg/ml has been used as a target for animals. In horses, effective plasma concentrations are 5–20 μg/ml (average 8.8 μg/ml ± 2 μg/ml) (Soma et al., 2001). Therapy should be aimed at producing concentrations above 5 μg/ml in horses, or a peak concentration of 15 μg/ml. Valproic acid (Depakene®) has been used in dogs primarily when they are refractory to other medications. The use has declined after other drugs became available (see Sections Zonisamide, Gabapentin, and Levetiracetam), but it has been used in some dogs successfully. The mechanism of action is unknown. It may interfere with GABA metabolism. Absorption from oral valproate is high in dogs, with values for the immediate-release tablets of 100% (Bialer et al., 1984) and sustained-release preparations of approximately 80%. The half-life in dogs is 1.0–2.8 hours (15–20 hours in people), or approximately 1.4 hours in another study in dogs (Bialer et al., 1984). This short half-life severely limits its therapeutic effectiveness unless frequent dosing schedules are used or sustained-release products are employed. But, some evidence suggests that the anticonvulsant effects of valproate persist long after the drug is eliminated from the plasma. According to some studies in dogs (Bialer et al., 1984; Bialer, 1992), the sustained-release tablets provide a more sustained plasma concentration than conventional tablets. However, half-life and extent of absorption may be different between valproate pharmacokinetics in dogs and humans; therefore, the human extended-release dosage forms may be applied to dogs, but should be administered more frequently than in humans (Bialer, 1992; Bialer et al., 1986). Divalproex sodium is composed of equal parts of valproic acid and valproate. It is pharmacologically equivalent to valproic acid and is available in delayed-release tablets of 125 and 500 mg (Depakote®, Epival®). Although valproate is not commonly used, some neurologists have reported it to be “reasonably effective” and well tolerated. Combining this drug with another anticonvulsant such as phenobarbital may increase its efficacy. Dosages that have been used in dogs range from 75 to 200 mg/kg q 8 h when used alone, or 30 to 40 mg/kg/day with phenobarbital (phenobarbital dose is usually decreased). Drug assays exist for therapeutic monitoring, but this is not commonly performed for animals. The therapeutic concentrations are reported to be 350–830 μmol/ml (50–120 μg/ml), but the true “therapeutic range” for valproate in animals is not known. Imepitoin is approved for treating epilepsy in dogs in Europe, but not the USA. In Europe, the brand name is Pexion, it was approved in 2013, and is considered a first-line treatment for idiopathic epilepsy in dogs. Veterinarians in the USA have not been familiar with imepitoin. The mechanism of action of imepitoin is to decrease seizures by potentiating the GABAA receptor-mediated inhibitory effects. Although structurally it is not classified as a benzodiazepine, these actions are similar to diazepam and other benzodiazepines (Figure 17.1). The similar action to benzodiazepines is caused by its low-affinity binding for the benzodiazepine binding site of GABAA, but it has 600 times less affinity for the binding site compared to diazepam. It also may have a weak calcium channel blocking effect, which may contribute to clinical effects. The approved dose for dogs of imepitoin is 10–30 mg/kg oral twice daily. It is available in 100 and 400-mg tablets, which can be split if necessary. It is recommended to start with 10 mg/kg, then increase dose to obtain the desired response. There have been no guidelines thus far for plasma/serum concentration monitoring; therefore, dose adjustment should be made on the basis of clinical response. The dose can be increased by 50 to 100% increments up to a maximum dosage of 30 mg/kg administered twice daily. In a US field trial it was compared to primidone in dogs. Imepitoin efficacy was lower than primidone, but was judged to be not inferior, and produced fewer adverse effects in dogs compared to primidone. In the field trials that led to the approval for use in Europe, the efficacy of imepitoin was compared to phenobarbital in dogs with idiopathic epilepsy. The European agency concluded that although there was lower overall efficacy for imepitoin compared to phenobarbital, some dogs were well-controlled with imepitoin treatment. They stated that although efficacy may not be complete with imepitoin treatment in dogs, it is considered a “suitable treatment” in some dogs because of its safety profile. The ACVIM consensus panel (Podell et al., 2016) gave it a high rating for use as single-drug monotherapy, but a rating of low as an add-on medication. In the published study in which it was compared with phenobarbital for idiopathic epilepsy in dogs (Tipold et al., 2015), there were significantly fewer adverse events with imepitoin compared to phenobarbital. Although the efficacy was lower for imepitoin than phenobarbital, the analysis showed imepitoin to be statistically noninferior. It has not been evaluated for treatment of status epilepticus. The use as an add-on treatment with other drugs has not been sufficiently tested, except in a pilot study in which there were add-on benefits (Rieck et al., 2006). There have been no studies reported in cats or other animals. Studies in dogs indicate that half-life of imepitoin in Beagle dogs was relatively short compared to other anticonvulsant drugs. The half-life was 1.5–2 hours, but it can be administered only twice daily (Rundfeldt and Löscher, 2014). Most of the elimination is by the feces. The authors of the study suggested that it may have shorter half-life in Beagle dogs than other breeds, but those studies may not be representative of clinical patients. Concentration profiles were not much different in fed versus fasted Beagle dogs. The total area-under-the-curve (AUC) was higher in the fasted dogs, even though this may not be enough of a difference to be clinically relevant. The protein binding was 56–57%, but no protein-binding drug interactions have been identified. The most common adverse effects from imepitoin reported in field trials were lethargy/sedation, polyuria/polydipsia, and increased appetite. Most reactions are mild and generally transient. Other reported effects are hyperactivity, hypersalivation, emesis, ataxia, diarrhea, and sensitivity to sound. In the clinical studies it produced fewer adverse effects compared to primidone or phenobarbital. In clinical studies, although phenobarbital was associated with increasing levels of the liver enzymes ALT, ALP, aspartate aminotransferase (AST), γ-glutamyl transferase (GGT), and glutamate dehydrogenase (GLDH), none of these enzymes increased with imepitoin. Although it is a partial agonist for the GABAA binding site, there is no evidence of tolerance or dependence that develop from repeated administration of imepitoin. Imepitoin is metabolized by the liver enzymes; therefore, interactions with other drugs that affect cytochrome P450 enzymes are possible, but have not been studied in dogs. It did not affect activity of most human P450 isoforms but inhibition of CYP1A1 is possible (Rundfeldt and Löscher, 2014). Imepitoin had no effect on enzyme induction compared to phenobarbital (Rundfeldt and Löscher, 2014). It is a partial agonist for the benzodiazepine receptor, but there is no evidence that it will prevent the activity of coadministered benzodiazepines used for acute treatment (e.g., status epilepticus). In a pilot study (Rieck et al., 2006), there was no evidence of interaction with coadministered phenobarbital in dogs.
Anticonvulsant Drugs
Introduction
Barbiturates
Phenobarbital
Mephobarbital
Primidone
Hydantoins
Phenytoin
Succinimides
Ethosuximide
Benzodiazepines
Diazepam
Clonazepam
Oxazepam
Midazolam
Lorazepam
Oxazoldinediones
Trimethadione
Paramethadione
Others
Imepitoin
Valproate
Carbamazepine
Potassium bromide
Levetiracetam
Gabapentin
Pregabalin
Zonisamide
Phenobarbital
Mechanism of Action
Pharmacokinetics
Drug Interactions
Side Effects, Adverse Effects, and Tolerance
Liver:
Hepatocutaneous disease:
Blood disorders:
Effect on hormones:
Corticosteroid metabolism:
Clinical Use
Treatment of status epilepticus:
Formulations:
Clinical Monitoring
Primidone
Pharmacokinetics
Clinical Efficacy
Adverse Effects
Clinical Use
Therapeutic Monitoring
Phenytoin
Mechanism of Action
Pharmacokinetics
Clinical Use
Horses:
Therapeutic Monitoring
Valproic Acid (Valproate)
Pharmacokinetics
Clinical Use
Imepitoin
Clinical Use in Dogs
Pharmacokinetics
Adverse Effects
Drug Interactions
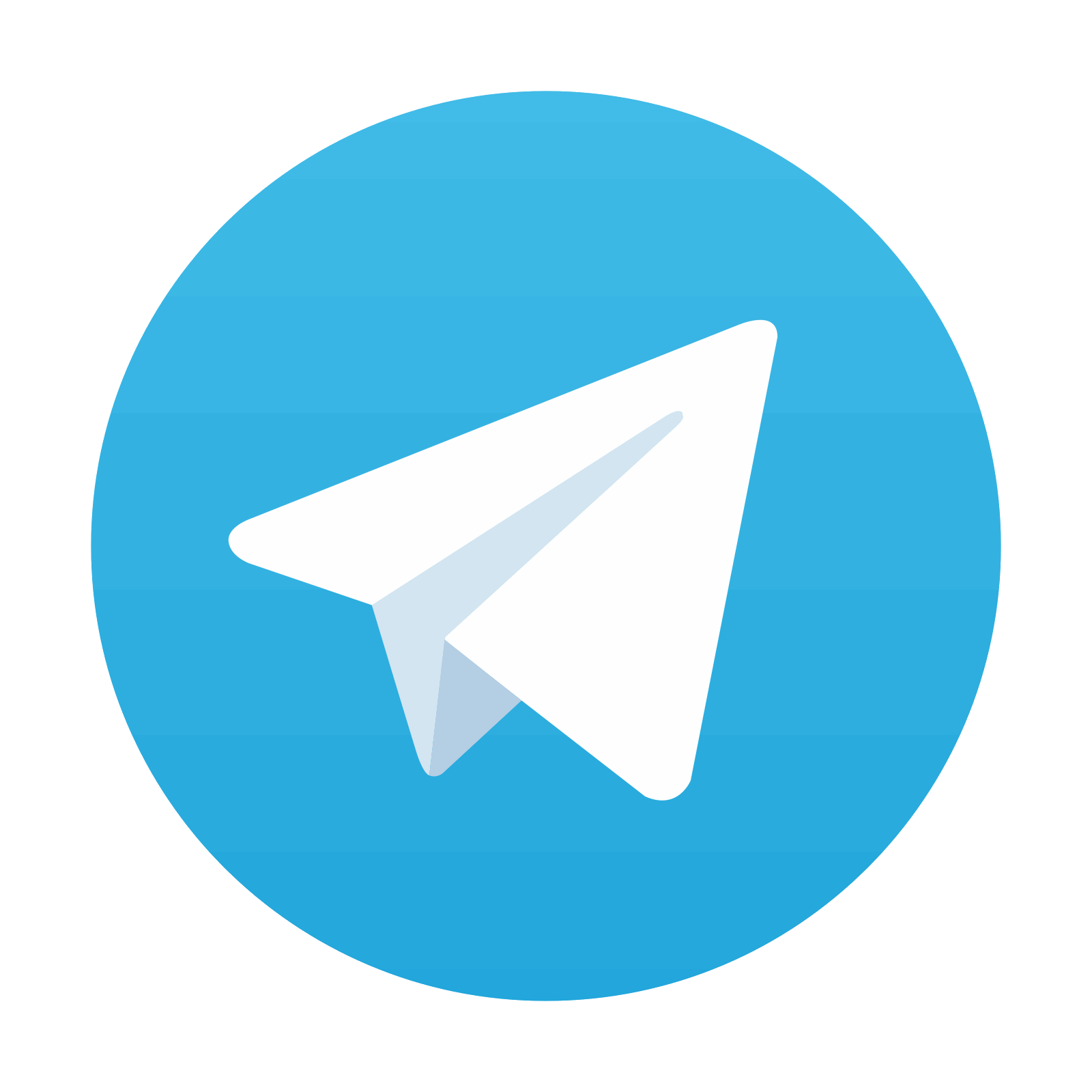
Stay updated, free articles. Join our Telegram channel

Full access? Get Clinical Tree
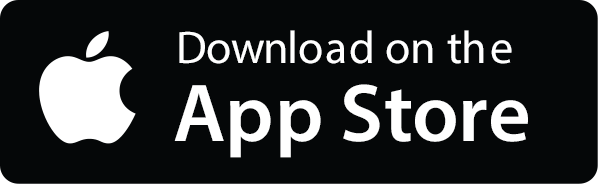
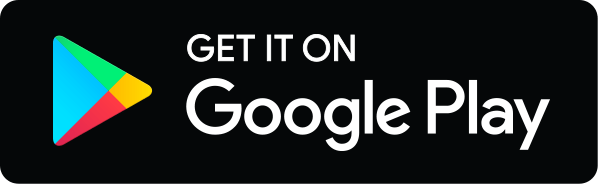