Karren M. Plain, Karen Stevenson, Richard J. Whittington, and Nathalie Winter The Mycobacterium genus includes diverse pathogenic and non‐pathogenic species. Non‐tuberculous mycobacteria are prevalent in the environment and can cause opportunistic tuberculosis‐like infections in many animals, including humans. Non‐tuberculous mycobacteria infections are important as they can cause false‐positive test results due to cross immune reactions and may not be distinguished from obligate pathogenic mycobacteria. The Mycobacterium tuberculosis complex (MTC) responsible for tuberculosis (TB) includes M. tuberculosis (Mtb), Mycobacterium africanum, Mycobacterium canettii, Mycobacterium bovis (Mb), Mycobacterium caprae, Mycobacterium pinnipedii, and Mycobacterium microti. While Mtb is largely responsible for TB in humans and 1.7 million deaths/year, Mb, responsible for bovine TB (bTB) is present in cattle and wildlife. Mb displays zoonotic potential; human TB cases acquired from infected animals are underestimated due to lack of resources to distinguish these genetically closely related species. Control of TB is a real challenge today and requires “one health” approaches, considering that the health of animals, humans, and the environment are connected. The vaccine Bacillus Calmette–Guérin (BCG) was obtained in 1921 by attenuation of a virulent Mb isolate. Today, this vaccine has been administered to more than three billion people and is recommended by the World Health Organization in countries with a high incidence of TB to protect children from the most severe forms of TB. Cattle and wildlife are also affected by Mycobacterium avium subspecies paratuberculosis (Map), which is responsible for paratuberculosis (paraTB) or Johne’s disease; this disease is widespread globally and represents an important source of animal suffering and economic loss. The mycobacteria are a very large and diverse group of predominantly environmental organisms, a small proportion of which have evolved opportunistic pathogenic tendencies and still fewer have become dedicated, obligate, pathogens of humans and animals (Table Table 34.1). Technically, the mycobacteria are Gram‐positive because of their cell‐wall architecture and peptidoglycan chemistry, but more importantly they are characterized by an in vitro characteristic: even when exposed to acid and heat, mycobacterial cell walls do not allow penetration of organic solvent. This enables the retention of a pink dye, carbol fuchsin, which is revealed through light microscopy. This commonly used taxonomic feature makes them “acid fast.” This resistant cell wall makes many disinfectants ineffective, allows ingested organisms to withstand gastric acid, and, through complex interplays with innate immune mechanisms, allows pathogenic mycobacteria to persist within tissues. Perhaps because of their environmental origins in diverse niches, the pathogenic mycobacteria have evolved a range of strategies to perpetuate their lineages in animal hosts: environmental persistence, resistance to lysis within phagocytes, avoidance or perturbation of adaptive immunity, long incubation periods before the health of the host is severely compromised, and exit from the host by a range of routes. Table 34.1 Examples of pathogenic Mycobacterium infections of animals. a) Full host range is unknown for many Mycobacterium species. b) The zoonotic potential of M. avium paratuberculosis is a controversial issue Mycobacterial genomes characteristically have a high G + C content and a small number of ribosomal RNA genes in relation to the size of the genome. Mb and Map have genomes of 4.32 Mb and 5.2 Mb with 66% and 69% G + C, respectively. Mycobacterial genomes contain numerous repeat sequences that present a challenge for sequencing and genome assembly. These include mobile genetic elements such as prophages and insertion sequences (IS) belonging to various families. Some IS have been targeted for diagnosis and molecular typing of mycobacterial species. The most important of these are IS1110 for the MTC, IS1245 and IS1311 for the M. avium complex, IS901 for M.a. avium, IS902 for M.a. silvaticum and IS900 and IS1311 for Map. Mycobacterial genomes encode a large number of genes involved in lipid metabolism, consistent with the presence of cell walls and membranes rich in lipids, glycolipids, lipoglycans and polyketides. Also present are many genes encoding enzymes involved in lipid oxidation pathways used for metabolizing putative degradation products of host cell membranes. Both Mb and Map are perpetuated through an obligate parasitic relationship with animals, but both species can persist in the environment after they have been shed from an infected host. In most cases, the source of obligate pathogenic mycobacterial infection is an infected host, either through direct contact or by indirect contact in a contaminated environment. Spread of mycobacterial infections over larger distances is generally through trade in livestock, facilitated by the cryptic nature of both infections during lengthy subclinical stages. The relative contributions of direct contact versus environmentally acquired infections to persistence of these pathogens in a population of animals will depend on the husbandry system. For example, environmentally acquired infections may be important in barn‐raised calves (Eisenberg et al. 2010). Mb and Map have spilled over into sympatric wildlife populations from their endemic domestic animal life cycles. Mb wildlife reservoirs include white‐tailed deer (Odocoileus virginianus) in North America, the European badger (Meles meles) in France, Ireland, and the UK, brush‐tailed possum (Trichosurus vulpecula) in New Zealand, wild boar (Sus scrofa) and red deer (Cervus elaphus) in the Iberian peninsula, and buffalo (Syncerus caffer) and lechwe antelope (Kobus leche) in Africa. Map circulates in wild deer populations in many parts of the world, and rabbits are a reservoir of Map in regions of Scotland. It is generally believed that this has arisen as a result of spillover infections from domestic livestock. For example, rabbits are a reservoir of Map in regions of Scotland. Evidence for interspecies transmission has been demonstrated by molecular typing and experimental infection. Furthermore, wildlife infections potentially compromise disease control in domestic species because of spillback into livestock. The best‐known examples of this are Mb infection in brush‐tailed possums in New Zealand and badgers in England. Rabbits excrete high numbers of Map bacilli in fecal pellets that can be ingested by grazing livestock. This, combined with a relatively high prevalence of infection, the high population density of rabbits with access to livestock pastures and the lack of avoidance of rabbit feces by grazing livestock presents a risk of Map infection for sympatric livestock. Map can persist on pasture and in soil or water and sediment for months, protection from incident solar radiation favoring longer‐term survival (Whittington et al. 2004, 2005; Eppleston et al. 2014). Mb also can survive in soil and water for months particularly in cooler temperatures (Barbier et al. 2017). Mycobacteria may have acquired parasitic and pathogenic lifestyles by coevolution in the environment with phagocytic amoebae, and both MTC and Map have been found in amoebae in the environment (Drancourt 2014; Samba‐Louaka et al. 2018). Whole‐genome sequencing (WGS) has facilitated construction of the most reliable phylogenetic trees of Mycobacterium strains to date. The evolutionary rate of pathogenic mycobacteria is very slow, ranging from 0.15 to 0.53 substitutions/genome/year for Mb (Crispell et al. 2019) and less than 0.5 for Map (Bryant et al. 2016). Owing to these slow evolutionary rates, just a few single nucleotide polymorphism (SNP) differences could represent decades of evolution. The MTC is thought to have evolved by clonal expansion from a smooth tubercule bacillus population within M. canettii (Galagan 2014). Several major lineages of human adapted pathogenic Mtb (designated L1‐L4, L7) and M. africanum (L5, L6, L9) and a recently discovered earlier lineage (L8) evolved, each primarily associated with a distinct geographical distribution and lineage‐associated differences in virulence, transmission and acquisition of drug resistance. The animal‐adapted members of the MTC, often referred to as ecotypes, and of which there are at least nine, are thought to have derived from an L6 ancestor with the characteristic chromosomal region of difference (RD) deletions RD7 to RD10 and are paraphyletic with some members more closely related to L6 than other animal‐adapted strains. Despite their similar host distribution, Mb and M. caprae ecotypes correspond to two monophyletic groups. The Mb lineage is itself divided into 12 monophyletic groups; four corresponding to the previously described clonal complexes Eu1, Eu2, Af1 and Af2 and eight unclassified groups. Other divergence events occurred from the Mb ancestral strains, but all strains remain classified as Mb. Phylogeography of Mb strains suggests an origin in East Africa and while some groups appear to have remained in East or West Africa, others have spread across the globe (Loiseau et al. 2020). The evolutionary success of Mb is due to its capacity to infect and transmit effectively in cattle, resulting in global spread through cattle trading. Phylogenetic analysis of the M. avium subspecies has shown that Map and M. a. avium evolved independently from M. a. hominissuis. WGS studies have clarified the phylogeny of Map strains. The Map lineage is subdivided into two major sub‐lineages designated S (sheep‐type; MAP‐S) and C (cattle‐type; MAP‐C) (Bryant et al. 2016). Members of these two groups can be distinguished with respect to their cultural characteristics, virulence and pathogenicity (Stevenson 2015). MAP‐S strains have been isolated from sheep, goats and camels whereas MAP‐C strains have a broader host range encompassing all ruminant types, humans and some wildlife. Strain type is not exclusive to host provenance since interspecies transmission can occur where prevalence of disease is high and where different species are in close proximity. MAP‐S can be further subdivided into type I and type III strains and MAP‐C has a subdivision encompassing the “bison‐type” strains. MAP‐S and MAP‐C can be differentiated by genetic polymorphisms, particularly by the presence/absence of large‐sequence polymorphisms that can be the result of insertions or deletions (Stevenson and Ahlstrom 2020) but also by highly conserved SNPs such as in IS1311. MAP‐S strains appear to have retained more ancestral genetic sequences and are more diverse than MAP‐C strains. Like Mb, MAP‐C strains appear to have evolved via a process of deletion of genetic regions, indels and SNPs. They are the predominant strains isolated from cattle, are also very efficient in infecting and transmitting between cattle and have probably been spread around the globe through cattle trading. The high discriminatory power of WGS has revealed greater diversity among virulent mycobacterial strains than previously recognized and has facilitated detection and differentiation of mixed strain infections (concomitant or sequential infections of a single host by genetically distinct strains) and microvariation (where an isolate undergoes intra‐host evolution) (Byrne et al. 2020). The presence of multiple strains with different genotypes can result in altered phenotypes such as antibiotic resistance and virulence and affect clinical outcome and transmission dynamics. WGS data has revealed the close genetic relatedness of cattle and badger isolates in sympatric populations and persistence of bacterial lineages on or near farms for several years despite interim clear herd tests (Biek et al. 2012). Combining Bayesian phylogenetic and machine learning approaches to analyze WGS data, Crispell et al. (2019) were able to infer that transmission of Mb occurred more frequently from badgers to cattle than vice versa and that intraspecies transmission occurred at higher rates than interspecies transmission for both, suggesting that both cattle and badgers need to be targeted in control programs. Current knowledge of virulence factors is derived from mining mycobacterial genomes (Mtb mostly) for homologs of genes encoding factors known to be virulent in other organisms, as well as the construction and analysis of mutant libraries associated with appropriate screens in cellular and animal models. It is not within the scope of this chapter to give a comprehensive account of mycobacterial virulence factors but key factors pertinent to Mb and Map virulence will be briefly described. The pathogenesis of mycobacterial disease largely depends on the immunoregulatory cell envelope. This highly structured and complex entity is a virulence factor per se that comprises unique lipids and glycolipids. The cell envelope also confers on mycobacteria their hydrophobicity and participates in natural resistance to some antibiotics. The cytoplasmic membrane comprises phosphatidylinositol mannosides (PIMs) and lipomannans and lipoarabinomannans (LAM) which both derive from PIMs. LAM from slow‐growing pathogenic mycobacteria, modified by mannose residues, exquisitely manipulate the immune system. LAM inhibits maturation of the phagosome upon entry into macrophages (MPs) by interfering with intracellular calcium concentrations; it antagonizes apoptosis of infected MPs to the benefit of mycobacterial multiplication and downregulates major histocompatibility complex‐II antigen presentation to favor mycobacterial escape from the immune response; and it targets the C‐type lectin receptor DC‐SIGN (dendritic cell‐specific intercellular adhesion molecule‐3‐grabbing non‐integrin) on the surface of dendritic cells (DCs) to downmodulate their maturation and capacity to activate the adaptive immune response (Koul et al. 2004; Dulberger et al. 2020). The external face of the cell envelope is composed of mycolic acids that are essential to the growth of mycobacteria. They can be free or attached to trehalose sugar to give trehalose dimycolate (TDM), also called “cord factor” in reference to the capacity of mycobacteria to grow as serpentine cords, which relies on TDM production. TDM is highly inflammatory and is involved in granuloma evolution and caseation. Free lipids at the surface of the cell envelope include phthiocerol dimycocerosate (PDIM) that is crucial for infection. PDIM masks pathogen‐associated molecular‐patterns (PAMPs) present in the mycobacterial envelope to avoid recognition by PAMP‐receptors and immune signaling (Cambier et al. 2014). The cell wall of Rhodococcus equi (Chapter 33) has similar characteristics and roles in its pathogenesis. ESAT‐6 (early secreted antigenic target of 6 kDa) and CFP‐10 (culture filtrate protein of 10 kDa) are secreted by virulent mycobacteria from the MTC. The history of discovery of these key virulence proteins goes back to the late 1990s when genomic comparison of all BCG vaccine strains used in different countries revealed deletion of one common chromosomal region called RD1. It appeared that RD1 loss was the principal cause of BCG attenuation (Behr et al. 1999). The RD1 locus encodes the ESX‐1 type VII secretion system that allows the ESAT‐6/CFP‐10 heterodimeric complex to be secreted. Both Mtb and Mb genomes encode 5 ESX systems in total but only ESX‐1 and 5 are involved in virulence. ESX‐1 is key to virulence: mutant strains deleted of the system are highly attenuated in mice and other animal models. Together with the cell wall compound PDIM (Augenstreich et al. 2017) ESAT‐6 breaks the phagosomal membrane of the MP to allow escape of bacteria into the cystosol. The ESAT‐6/CFP‐10 heterodimeric protein also drives necrosis of infected cells allowing progression of the disease, interferes with signaling pathways in infected MPs, and downregulates the immune response of the infected host. Since the ESX‐1 system is absent from M. avium and Map, which are also successful pathogens, why the ESX‐1 secretion system contributes to virulence only in some species remains unknown. Mycobacterial genomes contain numerous pe and ppe genes encoding the PE and PPE protein families, named after the conserved proline (P) and glutamic acid (E) residues in their N terminal domains. In Mtb, pe/ppe genes cover up to 10% of the genome. PE/PPE proteins may be membrane nutrient proteins or involved in pathogenesis and are transported across the inner mycobacterial membrane by the type VII secretion systems ESX‐1, ESX‐3, and ESX‐5. PE/PPE proteins can be membrane or cell wall associated, or secreted heterodimers. The PPE protein has three highly conserved N‐terminal alpha helices that bind to its cognate PE protein via hydrophobic interactions, which form a composite type VII secretion signal. The C‐terminals of the proteins are highly variable and have been used to classify the PE and PPE proteins each into five subgroups. They are involved in a number of mycobacterial virulence mechanisms: they inhibit phagocytosis by MPs and prevent acidification and/or maturation of the phagosome; interact with Toll‐like receptor (TLR) 2 and mediate cell apoptosis, cytokine secretion and necrosis as well as maturation and activation of dendritic cells; and inhibit reactive nitrogen species production. Enzymatic functions have been attributed to a few PE proteins; for example, they can function as lipases during starvation. They scavenge and cleave triacylglycerol as a nutrient source and have a role in iron homeostasis through mycobactin‐mediated and heme‐iron acquisition. Different members of the PE/PPE family express this large range of functions. Finally, all PE and PPE proteins possess many predicted and experimentally validated immunogenic epitopes that are of interest for development of diagnostics and vaccines. Of particular note are PPE18IV and PPE42V, which form part of the M72/AS01E and ID93 subunit vaccines against human TB, in development (Schrager et al. 2020). The number of mammalian cell entry (Mce) proteins in Mycobacterium spp. varies from 6 to 66. The genes encoding these proteins are organized into operons usually composed of two yrbE genes and six mce genes (mceA‐mceF). The number of operons varies but all mycobacteria possess the mce1operon. Mtb has four (mce1‐mce4), Mb has three (mce1, mce2, and mce4) and Map has eight (mce1‐mce4 and two copies each of mce5 and mce7). The operons do not appear to be co‐regulated and may function at different stages of infection. The Mce1, Mce2 and Mce4 proteins show 99.6–100% homology between Mtb and Mb. The homology between M. avium Mce proteins and the respective individual Mtb Mce proteins ranges from 56.2 to 85.5%. Alignment of Mtb and M. smegmatis Mce proteins reveals 58.5–68.5% identity. Since Mce proteins are present in non‐pathogenic mycobacteria and deletion mutants of some mce genes are still pathogenic, Mce proteins are also likely to have roles in mechanisms other than virulence. Our knowledge of the function of Mce proteins is derived from studies with Mtb. Mce1 proteins have been most studied for their role in mammalian cell invasion. They are functionally and structurally similar to ATP‐binding cassette transporters and are involved in the transportation of fatty acids and mycolic acids. Deletion of the mce1 operon leads to a decrease in the expression of genes required for lipid transport and metabolism, affecting the cell wall. Mce2 proteins may be involved in the metabolism and import of sulfolipids and deletion mutants of the mce2 operon in Mb are attenuated. Mce3 proteins also appear to be involved in adhesion and penetration of cells, are immunogenic and mutations have been associated with drug resistance. Mce4 proteins are involved in the catabolism of cholesterol, cell invasion and formation of granulomas. Mce proteins also play a role in modulating host cell signaling (Fenn et al. 2020). Successful infection by pathogenic mycobacteria relies on their ability to adapt to their extracellular and intracellular living conditions. They need to adapt to harsh environments, such as exposure to reactive oxygen species and reactive nitrogen species that are abundantly produced by the host cell, low pH, hypoxia in the granuloma, nutrient starvation, or change in carbon sources. Moreover, these conditions vary during the lengthy infectious process and so mycobacteria constantly adapt, doing so through several transcription factors and two‐component systems that sense the environment and upregulate or downregulate specific genetic programs. This two‐component system, composed of the PhoP sensor that activates the PhoR transcriptional regulator, is central to Mtb and Mb virulence. PhoP controls production of key virulence factors such as ESAT‐6 (Frigui et al. 2008) and the lipids from the cell wall (Walters et al. 2006). Deletion of the PhoPR system in Mb also attenuates virulence because bacteria become less resistant to acidic stress and display reduced capacity to arrest phagosomal maturation in the MP (García et al. 2018). Interestingly, the PhoPR system allows fine tuning of virulence depending on the targeted host: in animal‐adapted Mb and M. africanum strains, the PhoPR system induces low secretion of ESAT‐6 and lipids, which is compensated for by other mutations in the genome to allow productive infection. Humans are normally spill‐over hosts for Mb infections. However, there was an exceptional outbreak of Mb infections transmitted between humans in Spain in the 1990s which was due to overexpression of the PhoPR two‐component system (Gonzalo‐Asensio et al. 2014).
34
Mycobacterium
Introduction
Characteristics of the Organism
Mycobacteria Represent a Large Family with Few Successful Pathogens
Mycobacterium species
Natural host(s)a)
Infection/disease characteristics
M. avium avium/M. avium silvaticum
Birds
Avian tuberculosis
Ruminants
Enteric disease similar to paratuberculosis
Cats
Panniculitis, skin lesions/ulcers, disseminated infection
Macropods
Granulomas in liver, lymph nodes, spleen
M. avium hominissuis
Pigs
Granulomatous lesions in lymph nodes (neck) and gastrointestinal tract
Humans
Pulmonary infections, submandibular adenopathies, disseminated infections in patients suffering from acquired immunodeficiency syndrome
M. avium paratuberculosis
Ruminants, camelids, lagomorphs
Johne’s disease/paratuberculosis; chronic granulomatous enteritis
Humans?b)
Crohn’s disease in humans?
M. tuberculosis/M. africanum/M. canettii
Humans
Tuberculosis, primarily respiratory infection but can disseminate to other organs.
M. bovis
Ruminants, wild boar, mustelids, possums, cats, dogs, humans
Bovine tuberculosis: chronic granulomatous caseous‐necrotizing inflammation affecting primarily lymph nodes and lungs but can disseminate
M. caprae
Ruminants, pigs, humans
As for bovine tuberculosis
M. microti
Rodents, cats
Tuberculous skin lesions
M. pinnipedii
Flipper‐footed marine mammals
Tuberculous lesions in lymph nodes, lungs, mediastinum, pleura, liver and spleen
M. orygis
Antelope, humans
As for bovine tuberculosis
“Dassie bacillus”
Rock hyrax
Granulomatous lesions in the lungs, liver and spleen
M. mungi
Mongoose
Tuberculous lesions in nose, nasal cavity, skin and lungs
“Chimpanzee bacillus”
Chimpanzees
Granulomas in liver and spleen
M. suricattae
Meerkats
Granulomatous lesions in spleen, liver, head lymph nodes and lungs
M.genevense
Birds
Avian tuberculosis
M. leprae/M. lepromatosis
Humans
Hansen’s disease in humans characterized by skin lesions and infection of peripheral nerves
Eurasian red squirrels, armadillos (M. leprae)
Leprosy‐like skin lesions in Eurasian red squirrels; skin ulcers and infection of peripheral nerves in armadillos
M. lepraemurium
Rodents, cats
Leprosy‐like skin lesions
Non‐tuberculous mycobacteria (NTM):
M. abscessus, M. celatum, M. chelonae, M. fortuitum, M. gordonae, M. intracellulare, M. kansasii, M. marinum, M. nonchromogenicum, M. phlei, M. scrofulaceum, M. szulgai, M. terrae, M. triviale
Environment, various animals
NTM are prevalent in the environment but cause opportunistic tuberculosis‐like infections of many animals, including humans
Genome Peculiarities
Sources of Infection
Source of Infection: Evolution and Epidemiology
Virulence Factors and Pathogenomics
The Cell Wall
ESAT‐6/CFP‐10
PE and PPE Proteins
Mammalian Cell Entry Proteins
Regulation of Virulence
The PhoPR Two‐Component System
The Dos R/S/T Dormancy Regulon
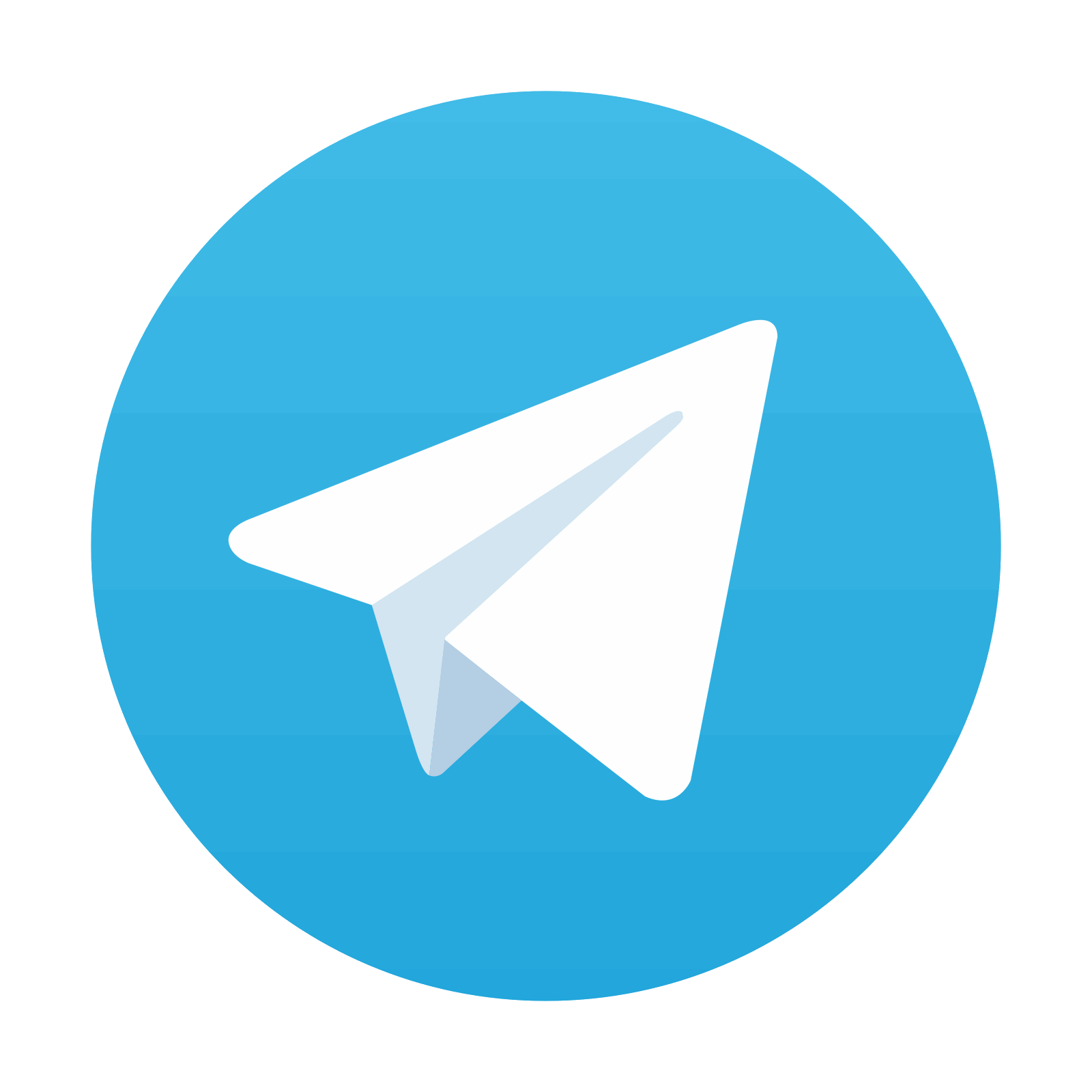
Stay updated, free articles. Join our Telegram channel

Full access? Get Clinical Tree
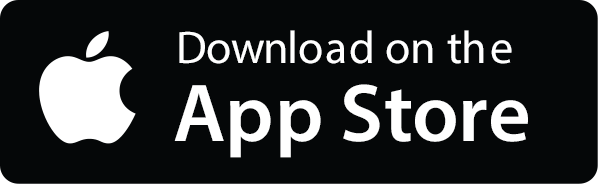
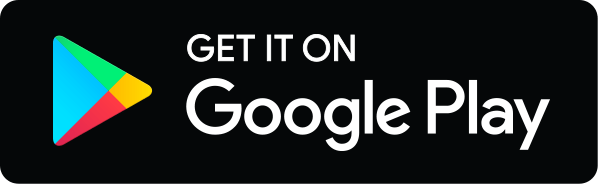