Jeff L. Caswell and Charles J. Czuprynski The genera Mannheimia and Bibersteinia contain mucosal commensals and pathogens for domestic and wild ruminants and other species. The genus Mannheimia contains seven validly published species (Kuhnert et al. 2021), with Mannheimia haemolytica being of the greatest importance. The genus Bibersteinia contains a single species, Bibersteinia trehalosi, which previously was designated biotype T (trehalose fermentation) of Pasteurella haemolytica. M. haemolytica and B. trehalosi are important members of the respiratory disease complex of cattle and domestic sheep, often in combination with other viral and bacterial respiratory pathogens. The genus Mannheimia consists of Gram‐negative non‐motile bacilli that are somewhat variable in morphology (rods and coccobacilli) and gram‐staining characteristics (i.e. bipolar staining). M. haemolytica produces small grayish colonies on blood agar that are weakly hemolytic (sometimes more easily recognized after the colony is removed). Historically, these organisms were included in the genus Pasteurella (e.g. Pasteurella haemolytica), together with Pasteurella multocida. The development of molecular tools revealed that these two species were quite distinct and led to establishment of the genus Mannheimia (Angen et al. 1999). Traditionally, M. haemolytica isolates were divided into 16 serotypes using typing sera for surface polysaccharide antigens. More recently a multiplex polymerase chain reaction assay has been developed that makes serotyping of the most common bovine serotypes 1, 2, and 6 more widely available and not dependent on typing sera (Klima et al. 2017). Sequence analysis can be used to divide M. haemolytica isolates into genotypes 1 and 2 (Clawson et al. 2016). Matrix‐assisted laser desorption ionization–time of flight mass spectrometry can be used to designate genotype at the time colonies are identified (Loy and Clawson 2017). Serotype and genotype are related to pathogenesis. Serotype 1 is most frequently associated with pneumonia, and most serotype 1 isolates are genotype 2. Serotype 2 is thought to be mainly an upper respiratory tract commensal infrequently associated with pneumonia, and most serotype 2 isolates are genotype 1. These relationships raise the possibility that if a genotype 1 (serotype 2) M. haemolytica is isolated from a case of bovine pneumonia, there might be another pathogen involved. The genus Mannheimia contains seven validated species (M. haemolytica, Mannheimia granulomatis, Mannheimia glucosida, Mannheimia ruminalis, Mannheimia varigena, Mannheimia caviae, and Mannheimia pernigra) of which M. haemolytica has by far the greatest impact. Formerly M. haemolytica was named P. haemolytica which contained 2 biotypes based on fermentation of arabinose or trehalose (A and T, respectively) and 16 serotypes based on surface polysaccharide antigens. What was previously known as P. haemolytica biotype T and its associated serotypes (3, 4, and 10) was moved to a new genus and species Bibersteinia trehalosi (Blackall et al. 2007). Separately, serotype 11 M. haemolytica was moved to a new species, M. glucosida, a significant cause of ovine mastitis (Omaleki et al. 2012). This chapter focuses on M. haemolytica and, to a lesser extent, B. trehalosi. M. haemolytica is generally considered to be a commensal of the nasal cavity and nasopharynx of ruminants such as cattle and sheep, and an opportunistic pathogen with the potential to cause pneumonia as part of the bovine respiratory disease (BRD) complex (Figure 11.1). These bacteria are not thought to cause disease on their own in immunocompetent hosts. Recent assessments of the microbiota of healthy and diseased calves have provided new insights into the commensal status of M. haemolytica. 16S rRNA gene microarray analysis of a relatively small number of feedlot cattle revealed differences in the nasopharyngeal microbiota between feedlot cattle that remained healthy and those that developed BRD (Holman et al. 2015). Some of the former and all of the latter were positive for Mannheimia. Analysis of deep nasal swabs and transtracheal aspirate samples from feedlot cattle in western Canada revealed lower bacterial diversity in the nasopharynx and trachea of steers with BRD than healthy animals (Timsit et al. 2018). The former also were more likely to have a nasopharyngeal microbiota that included Mannheimia. This study revealed that a microbiota containing Gram‐positive lactic acid bacteria was associated with healthy animals and was inhibitory to M. haemolytica in vitro (Amat et al. 2019). Nasal administration of these probiotic bacteria to Holstein bull calves protected them against nasal colonization with M. haemolytica. Results vary somewhat among microbiota studies. One analysis of nasopharyngeal and bronchoalveolar lavage samples from feedlot cattle reveal the more frequent presence of Bibersteinia than Mannheimia (Zeineldin et al. 2017). Under certain conditions (stress, viral infection) M. haemolytica descends into the lower respiratory tract, where it can cause a severe fibrinous pneumonia (Caswell 2014). Illness occurs following exposure to predisposing causes and recognizing and controlling these risk factors are a basis for disease prevention. In beef cattle, shipping fever pneumonia typically occurs after calves are weaned, transported, castrated, dehorned, comingled with cattle from other sources (such as in auction barns), transiently deprived of feed and water, castrated, handled, or experience other risk factors including change in weather, abrupt change to high‐energy diet, or infection with viruses such as bovine respiratory syncytial virus (BRSV/bovine orthopneumovirus), bovine alphaherpesvirus 1 (BHV‐1/infectious bovine rhinotracheitis virus), bovine parainfluenza virus 3 (BPIV/bovine respirovirus 3), bovine coronavirus, or bovine viral diarrhea virus (BVDV). In addition to weaned beef calves, M. haemolytica and B. trehalosi are important causes of illness and death in preweaned and weaned dairy and veal calves, lambs, and goat kids. Some predisposing causes are comparable to those above – comingling with animals from other sources, and infection with respiratory viruses or BVDV – but additional important risk factors include failure to receive adequate colostrum, reduced air quality in barns with suboptimal ventilation or high stocking density, shared housing with older animals, prior illness, inadequate plane of nutrition, and (for lambs) Mycoplasma ovipneumoniae infection. Finally, M. haemolytica causes pneumonia in dairy cows as outbreaks or sporadic cases (Biesheuvel et al. 2021), where the presumed risk factors include new animals entering the herd, recent calving, concurrent disease, and the factors mentioned above. Although M. haemolytica and B. trehalosi spread from animal to animal within a group, the disease is not considered contagious. Rather, outbreaks of pneumonia reflect a group of animals that experienced the same risk factors. Figure 11.1 Mannheimia haemolytica virulence factors and the pathogenesis of bovine respiratory disease (BRD). (a) Virulence factors include the polysaccharide capsule, leukotoxin (Lkt), lipopolysaccharide (LPS), adhesins, and O‐sialoglycoprotease. Furthermore, the ability of M. haemolytica to interact with the microbiome of the upper respiratory tract, form a biofilm, and invade bronchial epithelial cells may contribute to pathogenesis. (b) Ruminants are typically infected by direct contact with infected animals. (c) Serotype 2 (and genotype 1) bacteria colonize the upper respiratory tract but do not typically cause disease. (d) Serotype 1 (and genotype 2) bacteria also colonize the upper respiratory tract of healthy animals but have greater potential to cause disease. (e) BRD risk factors and predisposing causes lead to bacterial proliferation in the nasal cavity, impairment of respiratory defenses, and inflammation in the respiratory tract. (f) Thus, these predisposing factors as well as the bacterial virulence attributes allow M. haemolytica to colonize the lower respiratory tract. (g) There, the LPS, leukotoxin, and other bacterial components incite an inflammatory response, resulting in edema and fibrin exudation, leukocyte infiltration, and development of sepsis. Ig, immunoglobulin; NET, neutrophil extracellular trap; NO, nitric oxide; ROS, reactive oxygen species; RTX, repeats in the structural toxin. The severity of disease varies considerably and does not always correlate with bacterial burden (Bassel et al. 2020). Serotypes 1 and 6 are more frequently isolated from cattle with respiratory disease, whereas serotype 2 and others are more commonly isolated from healthy cattle. In contrast, serotype 2 is more commonly associated with respiratory disease in sheep. Bovine non‐clinical nasopharyngeal isolates are more commonly genotype 1, whereas lung isolates associated with respiratory disease are more commonly genotype 2 (Clawson et al. 2016). The latter are also more likely to contain antibiotic resistance elements. Mastitis in sheep due to M. haemolytica is thought to arise largely from bite wounds and transfer of the oral microbiota from suckling lambs to ewes (Fragkou et al. 2011). M. haemolytica can also play a part in the devastating polymicrobial pneumonia that occurs in bighorn sheep infected with M. ovipneumoniae (Besser et al. 2013). Bighorn sheep are not naturally colonized with M. haemolytica serotype 1, which is found in but is generally not pathogenic for domestic sheep (Subramaniam et al. 2014; Glendinning et al., 2017). Transmission from both domestic sheep and cattle to bighorn sheep has been reported (Foreyt and Jessup 1982; Wolfe et al. 2010). Bighorn sheep neutrophils are exceptionally responsive to the inflammatory effects of leukotoxins (LKT) (Silflow and Foreyt 1994), consistent with the severe pneumonia that occurs in bighorn sheep. Acute pneumonia is the best recognized manifestation of Mannheimia or Bibersteinia infection. Affected animals typically are depressed, which manifests as reduced feed intake, reluctance to move, and ear droop. This is often accompanied by increased respiratory rate and effort, cough, nasal discharge, increased body temperature, and increased lung sounds on auscultation. Demonstrating elevated serum haptoglobin or plasma fibrinogen levels supports the clinical diagnosis. Although the diagnosis is generally based on clinical signs, it is well‐recognized that clinical signs are neither sensitive nor specific for diagnosis of pneumonia: about two thirds of animals with lung lesions at slaughter were never diagnosed and treated during life. Innovations in clinical diagnosis of pneumonia include the use of thoracic ultrasound to detect lung consolidation or pleuritis, and cytologic analysis of bronchoalveolar lavage fluid to detect increased proportions of neutrophils. Once a clinical diagnosis of pneumonia is established, samples of nasal swabs, transtracheal wash, or bronchoalveolar lavage fluid from multiple animals prior to antibiotic therapy can be used for culture, to identify the specific pathogen and determine antimicrobial resistance patterns. The postmortem lesions of M. haemolytica and B. trehalosi are indistinguishable. The typical lesion is bronchopneumonia resulting in consolidation of the cranioventral areas of the lung (Figure 11.2a). Fulminant lesions affect an entire lobe (often the right middle lobe), and are very firm, purple‐black, covered by fibrin, and cut crisply (Figure 11.2b). Microscopically, alveoli and bronchioles are filled with fibrin, neutrophils, and macrophages, and often large numbers of bacteria. The neutrophils and macrophages often appear as “oat cells” (Figure 11.2c), a morphologically unique form of cell death with formation of streaming basophilic chromatin (Ackermann et al. 1994). Well‐demarcated irregularly shaped foci of coagulation necrosis are often present, surrounded by thick bands of dead leukocytes with streaming chromatin, and fibrin thrombi are occasionally present in these necrotic foci or in adjacent viable lung tissue. Lesions with more gradual onset are symmetrically distributed, red‐tan or red‐purple, firmer than normal, and have a clear demarcation between consolidated lobules and adjacent normal lobules. Histologically, neutrophils, macrophages and edema fill the alveoli and bronchioles. Although most animals respond well to early antimicrobial therapy, others develop chronic pneumonia that manifests as poor response to therapy or relapses after antibiotics are discontinued. These animals may or may not be febrile, and often have chronic cough, reluctance to move, and increased respiratory rate and effort. Although Mannheimia or Bibersteinia can sometimes be isolated from the lung in the chronic stages, these lesions usually involve multiple bacterial pathogens. The lesions in chronic cases include bronchopneumonia with abscesses, sequestrum formation, bronchiectasis, or development of Mycoplasma bovis pneumonia. Figure 11.2 Mannheimia haemolytica pneumonia. (a) Gross lesions in a colostrum‐deprived calf challenged by aerosol with M. haemolytica serotype 1, genotype 2. The cranioventral portion of the lung is red‐purple and firmer than normal, and fibrin covers the pleural surface of the lung and pericardial sac. (b) The focal lesion within the lung has coagulation necrosis in the center, surrounded by a basophilic rim of dead leukocytes. The adjacent lung is partially aerated, extending to the pleura at the right. Lung tissue at left is effaced by fibrous tissue reflecting chronicity. (c) An area of necrosis is at the left, and inflamed viable tissue is at right. The center contains many dead leukocytes with condensed streaming chromatin, typical of “oat cells”. Septicemia is the second major disease manifestation of M. haemolytica and B. trehalosi infections. B. trehalosi is a well‐recognized cause of septicemia in weaned lambs. This usually occurs as a sporadic disease in which one or a few lambs are found dead, and the postmortem lesions include widespread petechial hemorrhages and pulmonary edema. Ulcers of the upper alimentary tract are often present and thought to be a portal by which the bacteria enter the blood. Histologically, blood vessels in lung and other tissues are stuffed with innumerable bacteria. M. haemolytica can also cause septicemia in veal calves, with lesions of fibrinous pleuritis, pericarditis and peritonitis (Biesheuvel et al. 2021). Finally, in neonatal lambs or calves with acute bronchopneumonia caused by M. haemolytica or B. trehalosi, the bacteria can invade from the lung into the systemic circulation to cause fatal septicemia (Brown et al. 2021). M. haemolytica can cause otitis media in dairy and beef calves, although M. bovis is a more well‐recognized cause. M. haemolytica causes acute gangrenous mastitis in sheep. Mannheimia granulomatis causes granulomatous lesions in subcutaneous tissues of cattle and deer and is occasionally identified in lungs with pneumonia. M. haemolytica produces several products that allow it to act as an opportunistic pathogen. These include capsular polysaccharide (Adlam et al. 1984; Morck et al. 1989), fimbriae and outer membrane proteins that can aid attachment to host cells (Morck et al. 1987; De la Mora et al. 2006; Morton et al. 1996; Nardini et al. 1998; Mahasreshti et al. 1997; Clarke et al. 2001; Kisiela and Czuprynski 2009), lipopolysaccharide (Ali et al. 1992), various proteases and other enzymes (Lee et al. 1994; Rico et al., 2017; Ayalew et al. 2019), and a potent exotoxin, LKT (Shewen and Wilkie, 1982). LKT is regarded as the most important virulence molecule, as mutants that lack it are diminished in their virulence in experimental infections (Highlander et al. 2000), and natural infections are usually associated with LKT‐positive strains of M. haemolytica (Klima et al. 2014). Expression of lkt increased during the early stages of experimental infection in calves (Sathiamoorthy et al. 2011). LKT is a 104 kDa toxin produced during logarithmic growth (Shewen and Wilkie 1985) that is part of the repeats in the structural toxin (RTX) family of exotoxins produced by a wide array of Gram‐negative bacteria (Lo 1990). Its cytotoxic activity for ruminant leukocytes, but not those of other species, was first demonstrated in the 1980s (Shewen and Wilkie, 1982). Over time, it became clear that the biological effects of the LKT are time and concentration dependent (Figure 11.3). Like other RTX toxins, LKT can trigger release of inflammatory mediators and cause cell death by necrosis and caspase‐dependent mechanisms that resemble apoptosis (Ristow and Welch 2019). Neutralizing antibodies can block the effects of LKT in vitro, and provide partial protection in vivo (Shewen and Wilkie 1988; Confer et al. 1998). Seminal work by several laboratories demonstrated that LKT binds to CD18 on ruminant leukocytes (Li et al., 1999; Jeyaseelan et al. 2000; Deshpande et al. 2002; Figure 11.3). The species‐restricted binding reflects the presence of a signal peptide sequence on ruminant CD18 that is cleaved from CD18 on leukocytes from other species (Shanthalingam and Srikumaran, 2009). LKT can exert its effects both at the surface membrane of leukocytes and following internalization and transport to the outer membrane of mitochondria, where it disrupts mitochondrial function (Atapattu et al. 2008). It has been suggested that bovine CD18 alleles might differ in LKT binding and, by so doing, influence disease severity in cattle (Workman et al. 2018). The LKT operon is related in M. haemolytica, M. glucosida, and B. trehalosi, suggesting a common ancestry (Davies et al. 2002). The B. trehalosi LKT exhibits epitope differences that render it resistant to a potent neutralizing antibody against M. haemolytica LKT (Murugananthan et al. 2018). Figure 11.3 Role of leukotoxin (LKT) and lipopolysaccharide (LPS) in virulence. Mannheimia haemolytica adheres to respiratory epithelial cells. LPS released from the outer membrane binds to CD14/Toll‐like receptor 4 on macrophages and neutrophils. This activates these cells, resulting in procoagulant activity with cleavage of fibrinogen to fibrin, and greater expression of CD18, the cellular receptor for LKT. Thus, inflammation sensitizes leukocytes to the effects of leukotoxin. Lower concentrations of leukotoxin lead to leukocyte activation and degranulation, and greater concentrations lead to death by necrosis, NETosis or apoptosis. IL, interleukin; NET, neutrophil extracellular trap; TNF, tumor necrosis factor. Created with http://BioRender.com. There are structural differences in the lipopolysaccharide produced by the various M. haemolytica serotypes (Davies and Donachie 1996), although they have similar biological activities to LPS from other Gram‐negative bacteria. These include stimulating release of cytokines and other mediators from leukocytes and altering epithelial cells that come into contact with M. haemolytica cells or its LPS (Cai et al. 2020). In fulminant infections, LPS likely drives the production of various mediators that exacerbate inflammation and lead to the characteristic fibrinous pleuropneumonia (Whiteley et al. 1992). M. haemolytica produces several other molecules that play a role in pathogenesis or elicit an immune response during infection. These include an O‐sialoglyprotease, which cleaves O‐linked but not N‐linked or non‐glycosylated proteins (Abdullah et al. 1992; Watt et al. 1997). Although its specific role in pathogenesis is unclear, it can cleave bovine immunoglobulin G1 (Lee and Shewen 1996), alter surface proteins on platelets and lymphocytes (Sutherland et al. 1996; Nyarko et al. 1998), and elicit an antibody response after immunization or infection with M. haemolytica (Lee et al. 1994). Iron acquisition is a key step in bacterial virulence. M. haemolytica can obtain iron from bovine transferrin via transferrin‐binding proteins A and B (Ogunnariwo and Schryvers 1990). Immunization against transferrin‐binding proteins A and B conferred partial protection against experimental infection with M. haemolytica (Potter et al. 1999). We have a limited understanding of virulence regulation in M. haemolytica and related species. Iron in the growth medium affects production of leukotoxin and capsule (Gatewood et al. 1994; Avalos‐Gómez et al. 2020). Phage and prophage are common in the M. haemolytica genome and differ somewhat from phage in B. trehalosi or other Mannheimia species (Highlander et al. 2006; Klima et al. 2016). The role of phage in colonization or pathogenesis is not clear at this time (Hsu et al. 2013). M. haemolytica and B. trehalosi have a two‐component quorum sensing system (Malott and Lo 2002; van der Vinne et al., 2005); these are important for regulation of virulence in other bacterial species, but there is little information regarding M. haemolytica. Serotype 1 and 6 M. haemolytica have a type I‐C clustered regularly interspaced short palindromic repeats‐associated system that has been hypothesized to influence pathogenesis (Klima et al. 2016). Genomic studies identified antimicrobial gene resistance cassettes in M. haemolytica isolates (Klima et al. 2016). Nucleotide polymorphisms divide M. haemolytica into two major genotypes (1 and 2), with genotype 2 being associated with pulmonary disease and possessing an integrative and conjugative element for antimicrobial resistance (Clawson et al. 2017). The pathogenesis of M. haemolytica pneumonia involves a progression of infection from the upper respiratory tract to the lungs, and disease results from the interplay between bacterial factors and host responses. Transmission is generally by direct contact with respiratory secretions from an infected animal. Examples include nose‐to‐nose contact with an infected individual, or close contact of neonates with their dam. However, transmission may also occur via fomites such as contaminated equipment for feeding or watering, whereas there is no clear evidence of aerosol transmission. It is important to recognize that infection with M. haemolytica is contagious but the disease is not; that is, the bacteria are transmitted from one animal to another, but episodes of disease are thought to occur only when already‐infected animals are exposed to risk factors for the disease (Timsit et al. 2013). Nonetheless, calves with detectable M. haemolytica
11
Mannheimia and Bibersteinia
Introduction
Characteristics of the Organism
Pathogenic Species
Source of Infection: Ecology, Evolution, and Epidemiology
Types of Disease and Pathologic Changes
Virulence Factors and Pathogenomics
Regulation of Virulence
Pathogenesis
Host Association
Stay updated, free articles. Join our Telegram channel

Full access? Get Clinical Tree
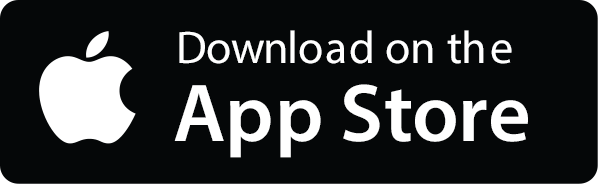
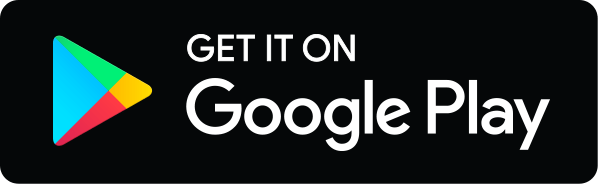