Chapter 13 Lens
ANATOMY AND PHYSIOLOGY
Development of the lens is described in Chapter 2. The lens is a transparent, avascular, biconvex body with an anterior surface that is flatter or less curved than the posterior surface (Figure 13-1). The centers of the surfaces are called the anterior and posterior poles. The rounded circumference is the equator, which has numerous irregularities where zonular fibers attach. Its anterior aspect is in contact with the posterior surface of the iris and fills the pupil. Its posterior aspect is in contact with the vitreous or, more specifically, a depression in the vitreous called the hyaloid (patellar) fossa.
The lens consists of the capsule, anterior epithelium, and lens fibers. It is divided into two general regions, the cortex (outer areas near the capsule) and the nucleus (central area) (Figure 13-2). As the lens grows throughout life, layers of fibers are produced in the equatorial area and are laid down on top of the former layers, forcing older fibers toward the lens center in a process resembling the formation of rings in tree trunks. These successive layers are visible clinically with biomicroscopy. They are called the adult, fetal, and embryonal nuclei, respectively (Figure 13-3).
The lens is supported at the equator by the lens zonules, or suspensory ligaments—collagenous fibers that attach to the processes of the ciliary body and suspend the lens in the middle of the pupil (Figure 13-4). Alterations of tension in these fibers alter the refractive (optical) power of the lens. To view nearby objects, the animal accommodates through contraction of the ciliary body muscles, mediated by parasympathetic stimulation. In primates and birds, this contraction leads to an increase in the curvature of the lens (i.e., it becomes more spheroid), thus increasing its refractive power (Figure 13-5). In carnivores, the contraction of the ciliary muscle results in forward movement of the lens in the eye, allowing the animal to accommodate for nearby objects. To view distant objects, sympathetic stimulation causes the animal to disaccommodate by relaxing its ciliary muscle. In primates and birds the relaxation results in a flatter lens with reduced refractive power. In carnivores it results in posterior movement of the lens in the eye. In general, the accommodative ability of birds and primates is superior to that of carnivores; most herbivores, reptiles, and rodents possess virtually no accommodative capabilities. However, it is worth remembering in this context that the cornea is the most important refracting surface in the eye, accounting for the majority of the optical power (because light undergoes significant refraction as it passes from air into the cornea). The lens accounts only for 30% to 35% of the eye’s refractive power and is used for fine adjustment for objects at different distances.
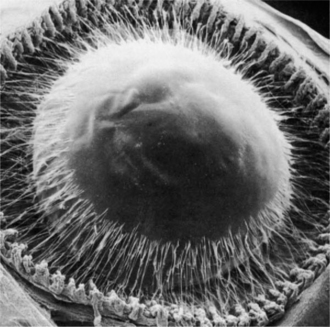
Figure 13-4 Scanning electron micrograph of anterior zonular insertion after removal of cornea and iris.
(From Streeton BW [1982], in Jakobiec FA [editor]: Ocular Anatomy, Embryology, and Teratology. Harper & Row, Philadelphia.)
Lens Components
Capsule
The capsule is a transparent, elastic envelope surrounding the lens (Figure 13-6). It provides insertion for zonular fibers that suspend the lens in the eye. In primates, the capsule regulates lens shape through its elasticity. The capsule is impermeable to large molecules (e.g., albumin, globulin) but allows water and electrolytes to pass. The anterior lens capsule, which is associated with the underlying epithelium, is much thicker than the posterior lens capsule, which lost its underlying epithelium during embryonic development (see also Figure 13-2).
Lens Epithelium
Cuboidal epithelial cells lie beneath the anterior capsule (see Figure 13-6). Toward the equator, the cells proliferate (through mitosis), become more columnar, and elongate into new lens fibers (see Figure 13-2). Because of mitotic activity in this area, these cells are susceptible to toxic and pathologic influences, which may become apparent as equatorial opacities. The lens epithelium is important in transport of cations through the lens capsule. The posterior lens epithelium, which transforms into lens fibers of the embryonic lens nucleus, is not seen in newborns and adults.
Lens Fibers
Lens fibers make up the substance of the lens and are arranged in interdigitating layers (Figure 13-7). These fibers stretch from the equatorial region toward the anterior and posterior poles of the lens. However, they do not quite reach the poles but instead meet fibers from the opposite equator and form a Y-shaped suture pattern with them (Figure 13-8). The suture pattern may become visible as a prominent upright (anterior) or inverted (posterior) Y if the lens becomes cataractous (see Figures 13-1 and 13-3). Because new lens fibers are formed throughout life, the older fibers in the (central) lens nucleus are denser and less transparent than the younger fibers laid down around them in the cortex. This difference between nucleus and cortex becomes more pronounced as the animal ages and may result in the formation of nuclear sclerosis (see later).
Metabolism and Composition
Metabolism of glucose provides most of the energy requirements of the lens. Glucose enters from the aqueous by both diffusion and assisted transport. Most of the glucose is broken down anaerobically to lactic acid via the hexokinase (pentose phosphate) pathway, although some aerobic glycolysis occurs via the citric acid cycle. Elevation in glucose levels (in diabetic patients) inhibits the hexokinase enzyme, and the glucose is diverted into the sorbitol shunt, where it is converted by aldose reductase into sorbitol (Figure 13-9).
The lens is high in protein (35%) and water (65%) and low in minerals. The proteins are divided into soluble proteins, or crystallins, and insoluble, or albuminoid, proteins. The former constitute approximately 85% of the lens protein content, but their proportion varies with species, location within the lens, age, and, most significantly, disease. The proportion of soluble proteins drops with age, and a similar process occurs when the lens becomes cataractous, as the proportion of insoluble proteins rises. During cataract formation, the lens proteins break into polypeptides and amino acids that diffuse through the lens capsule into the anterior and posterior chambers. Because these molecules are usually not recognized by the eye’s immune system, the breakdown and diffusion of cataractous lens protein usually trigger an inflammatory reaction known as lens-induced uveitis (LIU).
Nuclear Sclerosis
Throughout life, new lens cells are produced at the equator, forcing older cells toward the nucleus (see Figures 13-2 and 13-3). As the older cells become more tightly packed, the nucleus becomes denser and harder. In dogs, after about 6 years of age this greater nuclear density becomes visible as a grayish blue haze known as nuclear sclerosis (Figure 13-10). This haze is probably associated with increased insoluble proteins and decreased soluble crystallins (γ-crystallin) in the lens nucleus. Advanced nuclear sclerosis may appear similar to cataract, and in fact, the two are frequently confused by owners and practitioners. However, use of mydriatics and retroillumination (illumination of the lens by reflection of strong light from the tapetum) can help in differentiating between the two entities. The retroillumination will highlight the cataractous opacities, easily distinguishing them from the transparent nuclear sclerosis. In most animals, except for the most severe cases, the effect of nuclear sclerosis on vision is minimal, and the fundus can be readily visualized.
CONGENITAL ANOMALIES
CATARACT
A recent large-scale retrospective study covering 40 years and 230,000 dogs has shown that the prevalence of canine cataracts in North America has slowly been increasing and is reportedly 2.42% in the last decade. The increased prevalence is attributed to improved training and diagnostic techniques in veterinary ophthalmology and to the increased popularity of purebred dogs during the twentieth century. The overall prevalence of cataracts in mixed breed dogs, which presumably are not affected by hereditary cataracts, is 1.61%.
Classification
Cataract refers to a group of lens disorders of varying age of onset, speed and extent of progression, appearance, and etiology. Because of the variable nature and appearance of cataracts, numerous methods of classification are commonly used (Table 13-1).
Table 13-1 Summary of Cataract Classification
FEATURE | SUBCLASSIFICATION OF TERMS |
---|---|
Stage of development (maturity) | Incipient, immature, mature, hypermature, morgagnian |
Position within the lens | Anterior capsular, anterior subcapsular, cortical, equatorial, nuclear, posterior subcapsular, posterior capsular |
Age of development | Congenital, developmental, juvenile, senile, acquired |
Etiology or pathogenesis | Primary: inherited |
Secondary: traumatic, intraocular disease (uveitis, infection), nutritional, radiation, diabetic, toxic, congenital abnormalities, senile | |
Consistency | Fluid, soft, hard |
Cataracts may be classified according to cause. In many canine breeds, inheritance is the most common cause of cataracts. Additional causes are metabolic, traumatic, toxic, and developmental disorders of the eye. Cataracts also may be caused by nutritional deficiencies or may be secondary to other ocular diseases. Causes of cataracts are discussed in detail in a later section.
Stages of Cataract Development
Lens-Induced Uveitis
LIU is an inflammation of the eye caused by a reaction to the presence of lens antigens in the aqueous humor. The antigens usually leak from the lens into the anterior chamber following the degradation of lens protein in cataracts, thus causing phacolytic uveitis. The degradation and resulting LIU are limited in mature cataracts and more extensive in hypermature cataracts. Phacolytic uveitis is a humoral and cell-mediated immune reaction of the uvea to the released lens protein. The inflammation is a result of the fact that lens proteins are separated from the immune system before birth and are regarded as foreign. These antigens—especially the α-crystallins—are organ specific rather than species specific and cross species lines. Reaction to their presence is less severe in younger animals. A more severe form of granulomatous LIU may occur in older dogs with hypermature cataracts.
This inflammation must be treated medically (see Chapter 11) because it may gravely affect the prognosis of cataract surgery. Furthermore, the inflammation may cause secondary complications, including glaucoma and posterior synechia.
Causes of Cataracts
Hereditary Cataracts
In many pure breed dogs, inheritance is probably the most common cause of cataracts. The large-scale study cited earlier found 59 dog breeds that have a prevalence of cataracts higher than the “baseline” prevalence of 1.61% reported in mixed breed dogs. Seven breeds, including the toy and miniature poodle, had a cataract prevalence greater than 10%. Obviously, any breed in which the prevalence of a disease is higher than that of the general population should be suspected of being genetically susceptible to the disease. However, just as with any other disease, the inheritance of cataracts can be proven conclusively only through identification of a responsible gene, or by rigorous inheritance testing, including repeat breedings and cross-matings, over several generations. Such testing has demonstrated the inheritance of cataracts in several equine and bovine breeds and in approximately 20 canine breeds, including the Afghan hound, American cocker spaniel, bichon frise, Boston terrier, Chesapeake Bay retriever, German shepherd, golden retriever, Labrador retriever, miniature schnauzer, Old English sheepdog, toy and miniature poodle, Sealyham terrier, Staffordshire bull terrier, and wirehaired fox terrier (Table 13-2). Hereditary (and acquired) cataracts are very rare in cats.
In each of these 20 breeds the cataract is characterized by a typical age of appearance, initial opacity location within the lens, and rate of progression (or lack thereof) (see Table 13-2). Careful examination of young animals with inherited cataracts often demonstrates early, minute changes, but behavioral signs of visual impairment may not become evident until much later. Hereditary cataracts can be either recessive or dominant genetic traits. However, determining the genetics of a particular cataract in one breed does not exclude another genetic factor from causing a different type of cataract in the same breed. For example, there is evidence that both dominant and recessive cataracts are present in the golden retriever.
It is likely that the list in Table 13-2 is by no means final. On the basis of a very high cataract incidence and the same criteria (typical age, location, and progression), it is likely that cataracts are inherited in many additional canine breeds that have a cataract prevalence greater than 1.61%, including numerous terriers, spaniels, sheepdogs, and retrievers (see the Appendix).
Congenital Cataracts
When questioning the breeder, the clinician should ask the following questions:
Persistent pupillary membrane (PPM) (see Chapter 11) is inherited in the basenji, but familial and noninherited PPMs may be found in any dog breed and in numerous species. PPM may cause cataracts or corneal endothelial dystrophy, or both. Stationary anterior capsular cataracts develop if a strand of membrane adheres to the lens. The strand may or may not be absorbed before maturity; in either case, it will leave a permanent capsular opacity that may interfere with vision. Adhesion of a strand of pupillary membrane to the corneal endothelium results in permanent corneal endothelial dystrophy, which will similarly affect vision. Strands are not clinically significant if both ends attach to the iris or if one end is free in the anterior chamber. Some clinicians advocate surgical treatment of severe cases through corneal transplantation or cataract extraction. Others argue against surgery due to the stationary character of the cataract and corneal lesions, and because the PPM may be patent and ocular hemorrhage could occur during surgery. Medical treatment, using topical 1% atropine applied every 2 or 3 days to dilate the pupil and help vision, has also been suggested. However, many cases receive no medical or surgical treatment.
Persistent hyaloid artery and persistent hyperplastic primary vitreous are discussed in detail in Chapters 2 and 14. When the remnants of the embryonic blood supply contact the lens, cataracts of the posterior capsule and/or cortex result. The extent of visual interference depends on the size of the opacity. The remnants may persist as any of the following:
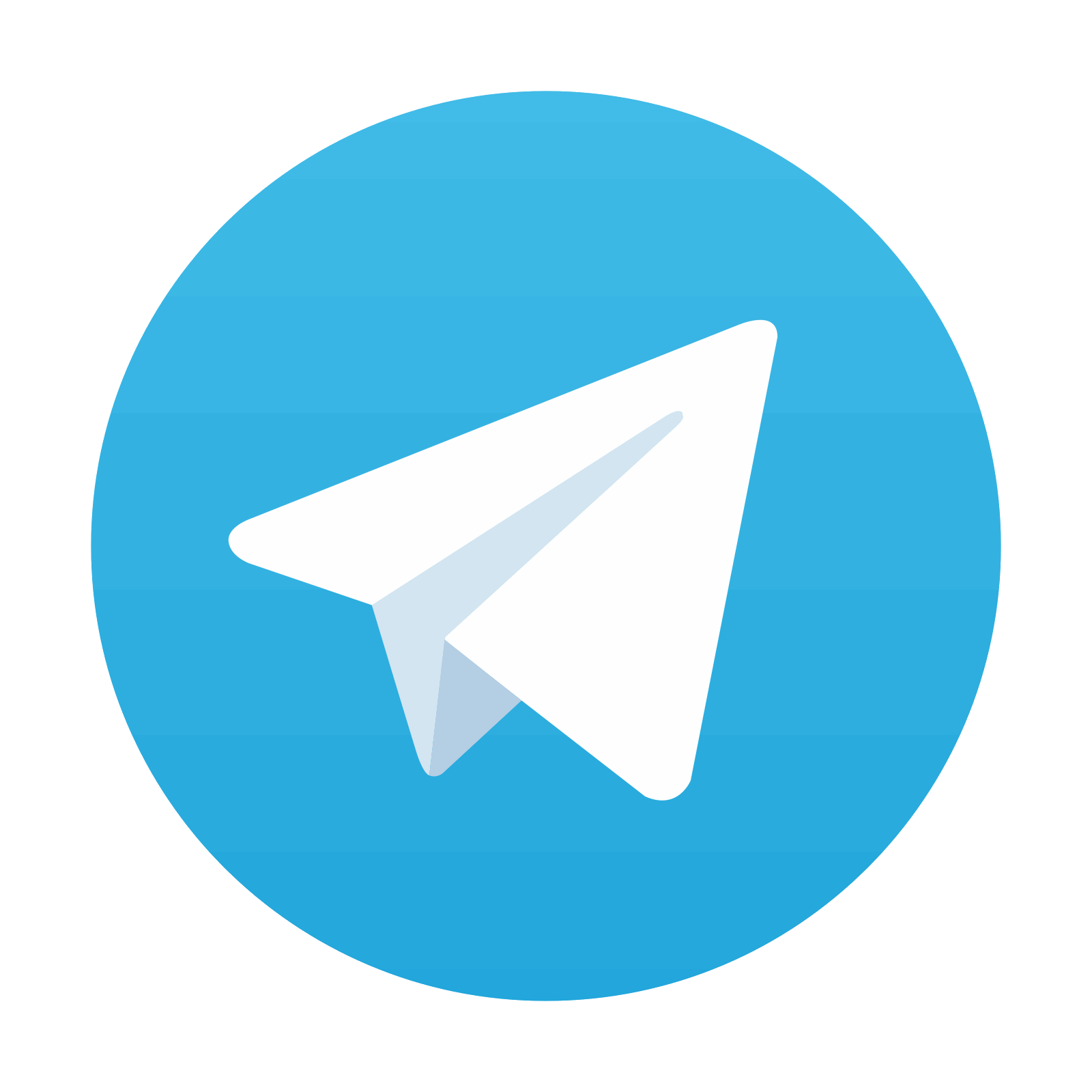
Stay updated, free articles. Join our Telegram channel

Full access? Get Clinical Tree
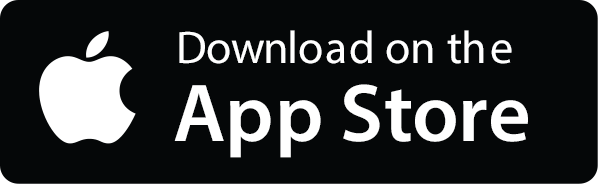
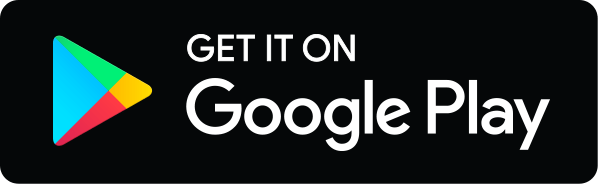