Chapter 6 Internal Parasites
Nematode Infection
Etiology and Pathogenesis
Gastrointestinal nematode parasites of sheep and goats are very similar to those of cattle, but the species encountered will differ as a result of host specificity (e.g., cattle species do not readily infect sheep and goats, and vice versa). The major gastrointestinal nematodes that parasitize pastured sheep and goats alike are Haemonchus contortus, Teladorsagia (formerly Ostertagia) circumcincta, Trichostrongylus spp. (predominantly Trichostrongylus colubriformis), Cooperia curticei, and Oesophagostomum spp. Of minor clinical importance are Nematodirus spp., Trichuris ovis, Bunostomum trigonocephalum, and Strongyloides papillosus. The particular species of nematodes that are primarily responsible for producing disease vary from region to region, where climate usually determines which pathogens are of greatest importance and weather determines the epidemiology of transmission. Most of these nematodes affect the abomasum or small intestine and cause greatest levels of clinical disease in young, growing animals. In much of the United States, H. contortus is the most important nematode with respect to clinical disease and economic impact. Although adult animals also are infected, clinical disease is not common in sheep (and, to a lesser extent, goats) that are older than 18 months, owing to development of immunity. High stocking rates (overcrowding) lead to overgrazing, which increases both the contamination of the pasture and the rates of exposure to infective larvae. Such pasture mismanagement often exists concurrently with malnutrition (inadequate nutrient or protein intake); this combination usually results in greater susceptibility to infection no matter what the age of the animals.1
The life cycle for all of the aforementioned parasitic nematodes is essentially the same: Eggs deposited by female worms are passed in the feces and hatch under favorable environmental (temperature and moisture) conditions, releasing the first-stage larvae. The first-stage larvae feed on organic matter in the feces and molt to the second-stage larvae, which continue to feed before molting to the third-stage larvae, the infective form. The third-stage larvae do not feed, because the cuticle of the second-stage larvae is retained, which serves as a protective outer sheath. The first- and second-stage larvae are susceptible to adverse environmental conditions such as high temperatures and desiccation, but the third-stage larvae are protected by the outer cuticle and can survive for an extended period of time (sometimes many months) either within feces or on pasture. During dry environmental conditions, fecal pellets tend to trap the third-stage larvae, because no moisture is available to facilitate migration out of the feces; therefore drought conditions followed by rain can result in devastatingly high rates of pasture contamination as larvae that have remained in the feces are released.1 Whether as an immediate or a delayed event, the third-stage larvae migrate out of the feces when adequate moisture (rain, flooding, heavy dew) is present and are then ingested by the host during grazing. The larvae exsheath in the rumen and find their way to the appropriate organ and penetrate into the mucosa, where they molt to the fourth-stage larvae. Under normal development conditions, the fourth-stage larvae migrates back out into the lumen and molt to the fifth stage of the life cycle (the immature adult), which then develop into the reproductive mature adult to complete the life cycle. Under environmental conditions that are harmful to survival and development of the free-living larval stages outside the host (winter cold or summer heat), the fourth-stage larvae will go into a state of arrested development (or hypobiosis) in the gastrointestinal mucosa and remain in that state for 3 to 4 months, at which time they resume development. The end of hypobiosis coincides with changes in the weather, which causes the environment to once again become conducive to development and survival of free-living parasitic stages.
Clinical Signs
Each nematode parasite induces its own specific pathologic changes in the host, but infections rarely are due to a single species but rather are caused by a mixture of many. Most infected animals will not display any outward manifestations of disease; however, when infections are severe enough to cause clinical disease, signs may include anemia, diarrhea, poor growth, weight loss, submandibular edema (bottlejaw), midline edema, decreased feed conversion, decreased milk production, and death. Which of these manifestations predominates depends on which species of pathogen are most numerous. Haemonchus contortus infection is the most devastating, particularly in hot and humid tropical and subtropical regions but also is a problem in many cooler, temperate regions1 (see Chapter 20).
Diagnosis
Antemortem diagnosis of gastrointestinal nematode infections usually is made by examining the feces for nematode eggs. Although a direct fecal smear can be examined, the mere presence of nematode eggs is not helpful in determining the parasite load of an individual animal or group of animals. Quantifying the fecal egg count (FEC) is the best way of estimating parasite loads. A quantitative McMaster technique for determining the FEC (reported as number of eggs per gram [EPG]) is illustrated in Box 6-1 and Figure 6-1.
BOX 6-1 Modified McMaster Egg Counting for Quantitation of Nematode Eggs
Saturated sodium chloride (table salt) solution (prepared as described below)
50-mL centrifuge tube with screw cap (Note: tube should be marked in 1-mL increments)
Pipette (Note: a 1-mL syringe or eye dropper works well)
Fresh fecal sample (kept refrigerated until tested, as described below)
Preparation of Saturated Salt Solution
1. Collect fresh feces that are uncontaminated by soil or bedding. (Note: Using a rubber glove, extract feces directly from the rectum. Alternatively, feces can be picked up off the ground if done soon after deposition.)
2. Label container with the name or number of the animal and the date of collection. Fresh samples work best, but accurate results can be obtained if the samples are kept refrigerated during the interim. If samples are not refrigerated, the eggs will hatch within 12 to 24 hours. Once hatched, they cannot be counted.
McMaster Egg-Counting Procedure
1. Weigh out 2 g of feces into a 50-mL centrifuge tube and fill to 30 mL with salt solution. It is recommended to purchase a small scale for accurate weighing of feces, but if a scale is not available, a close estimation can be achieved by placing 28 mL of salt solution into a 50- mL centrifuge tube and then adding feces until a volume of 30 mL is achieved.
2. Pour off approximately 25 mL of the salt solution into another small container, keeping feces in the tube (a tongue depressor can be used for this purpose).
3. Let soak for a few minutes and mix (soft feces) or break up (fecal pellets) with a tongue blade.
4. Add back approximately half of the salt solution and mix well, breaking up any remaining feces as well as possible.
5. Add back the remaining salt solution and screw the cap back onto the tube.
6. Shake tube vigorously for approximately 1 minute to homogenize any remaining feces as much as possible.
7. Set tube aside for a few minutes to let bubbles dissipate.
8. Wet McMaster chamber with water, and dry top and bottom on paper towels.
9. Rock (do not shake) tube side to side several times to thoroughly mix solution without causing large air bubbles to form.
10. Using 1-mL syringe or eye dropper, immediately take up a sample of the suspension and fill both sides of counting chamber. Work quickly. If it takes more than a few seconds to load the first chamber, then mix fecal solution again and refill pipette before loading the second chamber.
11. Let stand for 1 to 2 minutes to allow eggs to float to top.
12. Count all eggs inside of the two grid areas viewed under low power (using a 10x objective). Focus on the top layer, which contains the very small air bubbles (seen as small black circles; if numerous large air bubbles are visible, remove the fluid and refill).
13. Count only trichostrongyle or strongyle eggs (oval, approximately 80 to 90 μm long). Do not count Strongyloides (oval, approximately 50 μm long), tapeworm eggs (triangular or D-shaped), or coccidia (of various sizes). Notation is made regarding the presence of other species, but only the trichostrongyle or strongyle eggs are counted.
14. Once filled, the chambers can sit for no longer than 60 minutes before counting without causing problems. If the samples are permitted to sit for any longer, drying or crystal formation may begin.
15. Multiply the total egg count (from both chambers) by 50 to determine EPG (eggs per gram).
Common trichostrongyle eggs are shown in Figure 6-2, A. In addition, all eggs of trichostrongyle nematodes look similar and cannot readily be identified as to species. Knowing which species are predominant, however, has important implications for selection of control strategies. Thus culture of feces followed by larval isolation and microscopic identification has been the traditional method of identifying nematode species that are infecting an animal. However, this method requires a 10- to 14-day incubation period and considerable parasitologic expertise. A quicker and easier method is therefore highly desirable. Such a test for identifying Haemonchus eggs now exists.2,3 It has been demonstrated that peanut lectin will bind specifically to eggs of Haemonchus, but not to those of other trichostrongyle species. Therefore, by adding peanut lectin that is conjugated with fluorescein isothiocyanate to trichostrongyle eggs isolated from feces, it becomes possible to identify Haemonchus eggs using fluorescence microscopy. This test is easy to perform, and results are available the same day the sample is received. The only limiting factor is the requirement for a fluorescence microscope. Thus this test can be performed only in a reference laboratory.
In settings in which anemia causing nematodes (primarily H. contortus) are predominant, blood packed cell volume (PCV) and FAMACHA score both are good indicators of the level of blood loss and associated problems (Box 6-2). The FAMACHA card depicts five colors from red (healthy) to white (very anemic), which is then matched to the color of the inside of the lower eyelid of the affected animal (Figure 6- 3). The score correlates well with PCV and can be used easily in the field.4 (Note: the term FAMACHA is derived from it’s originators name, Professor Francois ‘Fafa’ Malan’s Chart.)
BOX 6-2 FAMACHA Guidelines
1. Ensure that all personnel using FAMACHA have been properly trained.
2. Because Haemonchus is the only parasite monitored with FAMACHA, attention should be paid to identifying and controlling other parasites when needed (e.g., by fecal egg count [FEC] or fecal culture)
3. Herd or flock examinations should be properly carried out every 2 to 3 weeks, and more often during peak parasite transmission times (i.e., hot and humid weather)
4. Monitor lambs and kids and animals that lag behind very carefully
5. Identify individual animals and keep accurate records
6. All animals needing deworming treatment three times more often than the flock or herd average should be culled
7. Institute sound nutritional, management, and selection practices that maximize health and minimize parasitic disease.
Treatment and Control Programs
Developing an effective modern worm control program is a dynamic process that requires regular periodic review and updating of management practices.1 The clinician should first take a thorough history and determine strengths and weaknesses of current practices to direct the design and implementation of an updated control program. Before deciding on which control measures to implement, however, the clinician needs to know which nematode parasites are predominant and which anthelmintics are effective against the species of concern.
Traditionally, control programs have relied on the use of broad-spectrum anthelmintics since their introduction in the mid-1900s. The overuse of these anthelmintics, however, has led to development of widespread resistance of nematode populations to many and sometimes all available anthelmintics, thereby making control extremely difficult.5 Over the past few decades, traditional approaches to worm control have been based on deworming all of the animals in the herd or flock. After deworming, the small number of surviving parasites will have little or no noticeable effect on animal health or production until approximately 25% of the worm population is resistant. The continous use of the same dewormer (or members of its class) in a flock or herd will over time result in a population of nematodes resistant to that particular class of deworming chemicals. This type of deworming program thus merely selects for resistant nematodes.
Nematode infections are not distributed evenly in an animal population; only approximately 30% to 35% of the animals harbor a majority of the nematodes. Thus nematode eggs shed in the feces of those animals constitute the vast majority of pasture contamination. Because anthelmintic resistance is genetically based, once resistance is present, no reversion to susceptibility occurs. Consequently, it is necessary to retain susceptible genes in a nematode population to extend the life of those anthelmintics that are effective. To achieve this end, it is necessary to leave some animals untreated. This requirement demands a change in mindset to a targeted selective treatment (TST) approach. It is now broadly accepted that this approach will help ensure that a refugium (portion of the worm population that is not selected by anthelmintic treatment) of susceptible nematode larvae (from nontreated animals) is maintained on the pasture, to help dilute out resistant genes from resistant nematodes that survived treatment. TST has been used successfully in settings in which H. contortus was the primary nematode.6,7 This success was made possible by using the FAMACHA system to identify the anemic animals needing dewormng, thereby leaving the nonanemic animals to provide the refugium. In areas in which other nematode species are predominant, FAMACHA is of little use, and FECs and body condition scoring should be used to identify the animals with heaviest infections. Another field method that has been used in young growing animals to identify the more highly parasitized of the group is evaluation for reduced weight gain (an indicator of the effect of parasites on production).6 This strategy requires regular weighing of all animals in the group; those that do not meet the desired weight gain threshold are separated and dewormed.
The Southern Consortium of Small Ruminant Parasite Control (SCSRPC) is a good resource for information concerning FAMACHA and parasite control in general. Specific links are available on the SCSRPC website (www.scsrpc.org).
Strategic deworming targets specific aspects of the epidemiology of the parasite. Transmission of nematode parasites depends on consumption of infective larvae during grazing, so the forage growing season is the primary target for controlling infection. Increasing temperature and available moisture (natural or artificial) provide the conditions for nutritious forage growth that gazing animals depend on for their growth and development. It is best to establish routine parasite evaluations (FEC, PCV, FAMACHA, or weight gain) at specified intervals (usually 2 to 4 weeks, depending on expected severity of problems) from parturition throughout the grazing season. TST can then be applied to establish or maintain refugia, with the aim of extending the useful life of the anthelmintics used. An exception to the recommendation for using TST is with lambs or kids to be sold for fattening or slaughter. Because these animals will be moved to confinement pens and then slaughtered, deworming them all before shipping will help put them in better condition without risk of contaminating pastures with resistant worms. For lambs and kids (or older breeding stock) to be sold as replacements, however, TST is appropriate, to minimize transporting surviving resistant worms onto the buyer’s farm.
Another strategic concept is deworming and then moving to “safe” pastures—areas in which the level of parasite contamination is low and reinfection of grazing animals will take longer. Examples of safe pastures are those in which sheep or goats have not grazed for 3 to 6 months (depending on the climate), pastures used for hay production, “new” pastures (i.e., those that have been used previously for crops or have been out of production), and pastures grazed by horses or cattle. The use of safe pastures can be beneficial, but it is paramount that TST be implemented to ensure that a refugia of susceptible worms will be maintained on the new pasture. If all animals are dewormed, then only resistant worms will contaminate the new pasture, which will hasten development of resistance to the anthelmintics being used. During the grazing season, pasture rotation at approximately 30-day intervals is commonly done to take advantage of the nutritive value of growing forage. Unfortunately, 25 to 30 days also is the optimal period for developing larvae to be readily available for reinfection when temperature and moisture conditions are right. Accordingly, rotating pastures at less than 3-month intervals during the grazing season may be ineffective in significantly reducing numbers of infective larvae unless high temperatures and low rainfall result in rapid desiccation of the fecal pellets, which will kill a large proportion of developing larvae. In warm climates (with mild winters), during cooler months, pasture rotation generally is ineffective, because low temperatures and retained moisture provide an environment for extended larval survival.8 However, if eggs or larvae are exposed to frequent hard freezes separated by thawing temperatures, then most will be killed.
In northern temperate climates, when ewes or does are moved to a dry lot or barn for the winter, a strategic TST deworming as they are moved off pasture will help keep the parasite burden low throughout the winter. Hypobiosis also is common in northern temperate climates, where a majority of the parasites (the ones of concern are the most pathogenic, primarily T. circumcincta and H. contortus) are inside the animals and not on the pasture. Decreasing the numbers of hypobiotic larvae before emergence in the spring helps to reduce the periparturient rise in FEC and resultant pasture contamination. To this end, the anthelmintic has to be effective against hypobiotic larvae. The macrocylic lactones have the best efficacy, but some of the benzimidazoles may be partially effective. Because it is not possible to determine which animals harbor the highest number of hypobiotic larvae, TST cannot be used effectively. So in this particular circumstance (usually in midwinter), deworming all animals may be justified. However, some adult animals may not require treatment during the grazing season and probably also have relatively low levels of infection with hypobiotic larvae. So if multiple-drug resistance is a problem on a given farm, it still would be wise to leave some of the animals untreated (based on previous low infection history) to supply refugia in the spring. If ewes or does are dewormed as described, spring periparturient deworming may not be necessary, but it is prudent to do an evaluation and then deworm (i.e., using TST) those animals with the heaviest infections. This strategy will help keep FEC and pasture contamination low during the spring periparturient period. Unfortunately, in warmer temperate to subtropical environments, this method is less effective, because larvae can survive on pasture during the winter months and hypobiosis is not a major factor in the winter or summer as it is in cattle (e.g., Ostertagia ostertagi in the summer). In these regions, routine but reduced interval infection monitoring can identify ewes and does with the heaviest infections warranting TST, which will help during the spring periparturient period to reduce FEC and pasture contamination. Well-nourished animals also are recognized to tolerate parasitism better, and the addition of a protein supplement overlapping the expected periparturient period has been shown to decrease the number of worm eggs shed around the time of parturition; however, the cost of the protein supplement may outweigh its benefits.9
Opportunistic deworming and salvage deworming usually are less effective in long-term management. Many times salvage deworming programs are used to save the lives of heavily parasitized animals. If animals are dewormed only after showing severe signs of parasitism (e.g., bottlejaw, severe anemia), animal and flock or herd productivity will already have been depressed. Deworming during handling for other procedures (e.g., castration, vaccination, shearing) is an example of an opportunistic program. It is convenient but is not conducive to long-term productivity. In view of the serious potential impact of parasitism on the health and productivity of small ruminants, animal work should be scheduled around parasite management programs, not vice versa.10 Integrating these other management procedures into the regularly scheduled parasite monitoring program works quite well.
Suppressive deworming programs entail the use of anthelmintics at regular intervals, usually every 2 to 4 weeks. Such suppressive programs have been in common use over the past couple of decades and initially were quite effective. Of note, however, they are labor-intensive, tend to be very expensive, fail to identify animals with superior immunity to parasites, and ultimately (and most important) have been the main cause in the development of anthelmintic resistance. As a general rule, the more frequently deworming occurs, the quicker resistance develops to anthelmintics. After deworming, as mentioned earlier, only resistant parasites remain to reproduce freely, resulting in proliferation of resistant worms. Using anthelmintics that remain in tissues at subtherapeutic concentrations for extended periods and treating and retaining immunocompromised animals will further encourage the development of anthelmintic resistance. Practices that ensure adequate dosages, proper dosing techniques, and appropriate types of anthelmintics should be emphasized.11 Animals can still be wormed at 2- to 4-week intervals, but only for the purpose of regular infection monitoring, and instead of mass deworming, TST should be implemented.
In view of the current situation, in which multiple-drug resistance is highly prevalent and few new anthelmintics are in development, clinicians should do everything they can to minimize the further development of anthelmintic resistance, both through their own actions and by counseling owners on the importance of proper management to enhance control programs and use of anthelmintics. The anthelmintics that have been used previously, the route of administration (e.g., PO, SC, IM, pour-on), frequency of use, and duration of use should be determined. Records of the results of infection evaluation (e.g., FEC, FAMACHA, PCV) should be maintained.
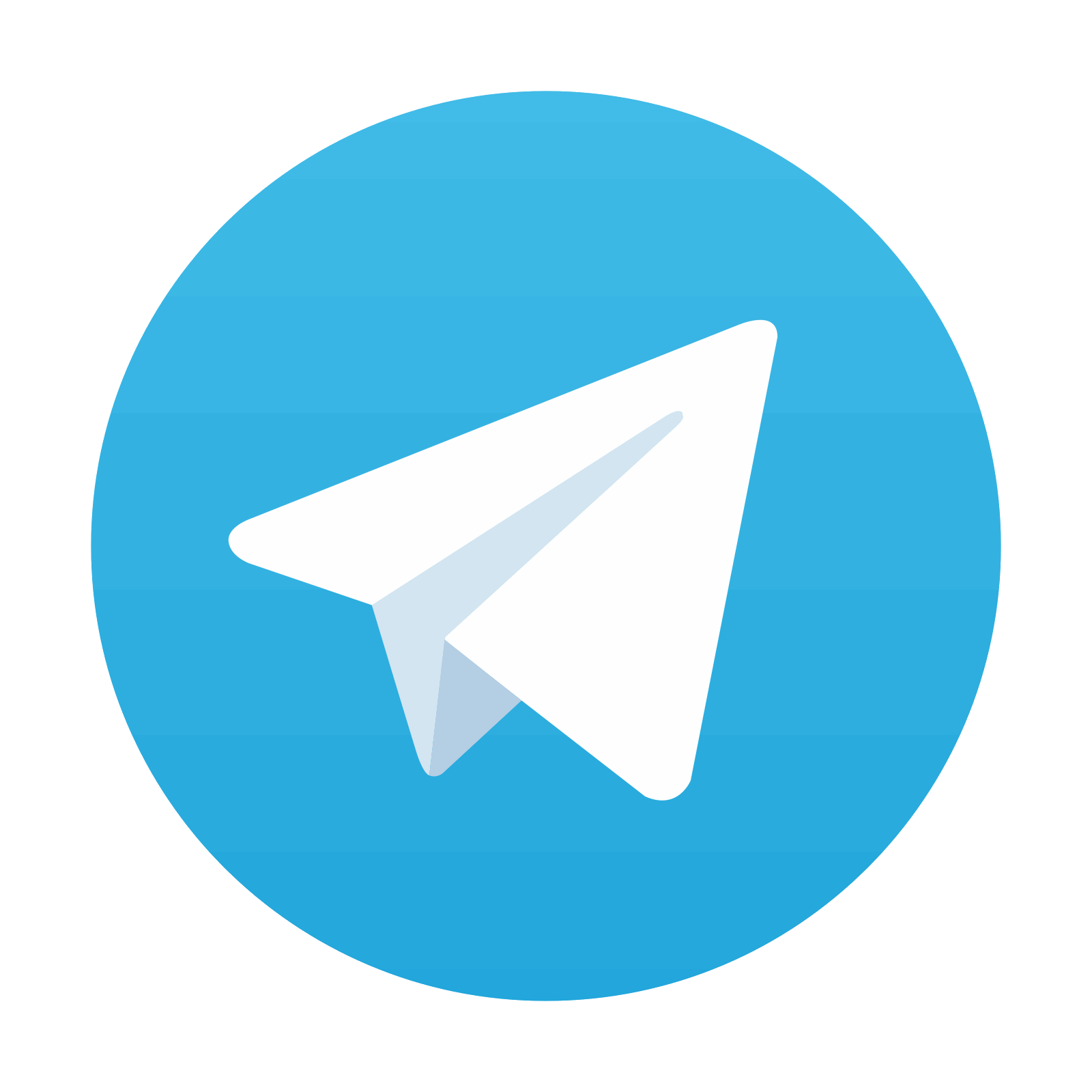
Stay updated, free articles. Join our Telegram channel

Full access? Get Clinical Tree
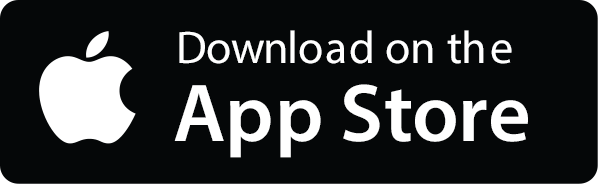
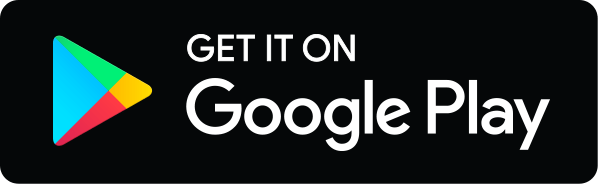