45 Mark G. Papich Conditions that require immunosuppressive drugs include skin diseases such as pemphigus, pemphigoid, and lupus-like syndromes. Systemic immune-mediated diseases requiring immunosuppressive drug therapy include immune-mediated hemolytic anemia (IMHA), systemic lupus (SLE), and immune-mediated thrombocytopenia (ITP). For management of some of these disorders, corticosteroids, alkylating agents, cytotoxic drugs, cyclosporine, and gold compounds have been used. Nonsteroidal antiinflammatory drugs (NSAIDs) alone are not usually effective. One of the problems with assessing these medications in veterinary medicine is that there is not enough good quality data to compare drugs, or to understand which one is best for specific conditions. For example, in a systematic review of treatment for IMHA – one of the most important immune-mediated diseases seen by veterinarians – the authors concluded that “This review shows that there are few pieces of high-quality evidence available to inform clinicians managing cases of IMHA in dogs.” (Swann and Skelly, 2013). Glucocorticoids are usually the initial drugs used for treating immune-mediated diseases. They are consistently effective, but have multiple side effects and adverse effects, especially with long-term use. The pharmacology of glucocorticoids is discussed in detail in Chapter 29 and readers are referred to that chapter. Briefly, glucocorticoids exert their action via binding to intracellular receptors, translocating to the nucleus, and binding to receptor sites on responsive genes (Rhen and Cidlowski, 2005; Boumpas et al., 1993), where they modulate the transcription of glucocorticoid-responsive genes (Barnes and Karin, 1997; Barnes, 2001; Hayashi et al., 2004; Rhen and Cidlowski, 2005). By regulating glucocorticoid-responsive genes, protein synthesis is altered, which affects cell function. The mechanism of action for the immunosuppression caused by corticosteroids is complex. In the study by Ammersbach et al. (2006), the authors demonstrated decreased neutrophil migration and survival, decreased cytokines, decreased adhesion expression, and a decrease in the cytotoxic lymphocyte function as well as lymphocyte apoptosis. The dose used in this study in dogs was approximately 2 mg/kg per day for 3 days. Corticosteroids increase the circulating numbers of mature neutrophils. There is a release of cells from the marginal pool of neutrophils and decreased migra-tion and egress into inflammatory sites. This effect is attributed to decreased expression of adhesion molecules, reduced adherence to the vessel endothelium, and reduced diapedesis from the vessels. Subsequently, there is decreased movement of inflammatory cells into tissues in response to chemotactic stimuli. Glucocorticoids also affect many functions of macro-phages. They suppress the function of macrophages and decrease the inflammatory response, generation of cytokines, and the ability of macrophages to process antigens. They also inhibit phagocytosis by macrophages. Glucocorticoids affect leukocyte traffic more than cellular function. Corticosteroids decrease the numbers of lymphocytes in the peripheral circulation – caused by a redistribution of circulating lymphocytes – depress lymphocyte activation, and decrease participation of lymphocytes in inflammation. T cells are affected more than B cells. Because B cells are more resistant to the immunosuppressive effects of glucocorticoids, there is minimal effect on immunoglobulin synthesis. However, high corticosteroid doses will decrease immunoglobulin levels, probably because of increased catabolism, as well as through the secondary effects to suppress accessory cells and cytokine synthesis. At antiinflammatory doses, glucocorticoids do not decrease an animal’s ability to mount a normal immune response (e.g., from vaccinations) (Nara et al., 1979). Other effects of glucocorticoids include inhibited release of inflammatory cytokines from leukocytes (e.g., IL-1, TNF-α, prostaglandins) and decreased expression of cytokines from lymphocytes (e.g., IL-2). It is difficult to separate the antiinflammatory from the immunosuppressive effects of glucocorticoids. For a complete review of these effects the articles by Cohn (1991) and Barnes (2006) should be consulted. For immune-mediated disorders (e.g., autoimmune skin disease, IMHA, and thrombocytopenia, SLE, immune-mediated polyarthritis) glucocorticoids suppress the immune system response to alleviate clinical signs. In a study in which various treatments for IMHA were examined, corticosteroids alone were as effective as combination treatments (for example, with azathioprine, cyclophosphamide, etc.) (Grundy and Barton, 2001). For long-term therapy, intermediate-acting steroids (prednisone, prednisolone) are used most often because they can be administered on an every-other-day (EOD) basis. However, injections of dexamethasone are often used for acute, short-term treatment. There are no demonstrated differences in response between dexamethasone and prednisone/prednisolone for acute treatment, but long-term adverse effects may be less for the shorter-acting agents such as prednisone/prednisolone. The immune-suppressing effects require higher dosages of corticosteroids than are necessary for antiinflammatory therapy (often by a factor of at least 2-fold), although dose-response relationships have not been fully studied in veterinary medicine. In one study in which the authors documented response to treatment of IMHA, the starting dose of prednisolone was 2 mg/kg per day, followed by a tapering schedule (Piek et al., 2008). In a systematic review of treatment for IMHA, the authors found that glucocorticoids alone will result in a successful outcome in a high proportion of cases, although there is no consensus on the drug dose or duration of treatment (Swann and Skelly, 2013). Initial (induction) dosage regimens employ daily doses of 2.2 to 6.6 mg/kg (prednisolone or prednisone). Doses greater than 6 mg/kg are rarely used in dogs. Initial doses generally are in the range of 2–4 mg/kg/day. One study reported that the optimal induction doses for skin diseases were 4.4 mg/kg/day for dogs and 6.6 mg/kg/day for cats. A systematic review of treatment outcomes from IMHA in dogs concluded that prednisolone greater than 2 mg/kg every 12 hours are likely to result in unacceptable adverse effects without apparent improvement in short- or long-term outcomes. After an induction period, if the patient responds favorably, the dose may be decreased by approximately 50%. Eventually, one should attempt to maintain the patient on this low dose on an every-other-day EOD basis. Some diseases are slow to respond and a reduction of doses should be performed on a gradual and prolonged schedule. For example, lowering the dose based on an evaluation every 2–4 weeks may be necessary. Eventually, for immune-mediated disease, maintenance doses of 1 mg/kg, EOD are possible but higher doses or adjunctive therapy may be necessary in individual patients. Side effects associated with treatment are discussed in Chapter 29. These effects are not necessarily reasons for discontinuing therapy, and include polyuria/polydipsia, increased appetite (polyphagia), and behavior changes (increased restlessness for example). Adverse effects from immunosuppressive doses of corticosteroids include risk of infectious diseases, adrenal suppression, pancreatitis, delay of healing, catabolic effects on muscle and connective tissues, and hepatopathy. If a glucocorticoid is used that has a duration of action of 12–36 hours (intermediate-acting), the hypothalamic–pituitary–adrenal (HPA) axis has an opportunity to recover before the next dose. It is important that one chooses a glucocorticoid that does not have a long duration of action for EOD therapy – for example, dexamethasone is unacceptable because its duration is at least 36–48 hours. After 1 mg/kg every 48 hours of prednisolone, adrenocorticotropic hormone (ACTH) was suppressed in dogs for 18 to 24 hours, and returned to normal until the next scheduled dose (Brockus et al., 1999). EOD therapy will minimize, but will not prevent adrenal atrophy, and other adverse effects such as those on the immune system and the effects on metabolism. Cyclosporine is a fat-soluble, cyclic polypeptide with immunosuppressive activity (Figure 45.1). It was derived from the soil fungus Tolypocladium inflatum. The proper spelling of the name has been a source of confusion. The US adopted name (USAN) is cyclosporine. The British Approved Name (BAN) is ciclosporin, which is the same as the International Nonproprietary Name (INN). Previously used names are cyclosporin and cyclosporin A. The designation of “A” after the name is no longer necessary. It has been an important drug used in humans, primarily to produce immunosuppression in organ transplant patients. It has gained recognition for veterinary use because of availability of a veterinary formulation of cyclosporine (Atopica). The veterinary product is FDA-approved for both dogs and cats. A review published in 2014 (Archer et al., 2014) provides details of previous studies, pharmacokinetics, and studies in dogs. Figure 45.1 Structure of cyclosporine (left) and tacrolimus (right). Cyclosporine binds to a specific cellular receptor on calcineurin and inhibits the T-cell receptor-activated signal transduction pathway by suppressing the nuclear factor of activated T cells (NFAT) (Figure 45.2). Particularly important are its effects to suppress interleukin-2 (IL-2) tumor necrosis factor-α (TNF-α) and other cytokines, and block proliferation of activated T lymphocytes. The action of cyclosporine is more specific for T cells as compared to B cells. It has little effect on humoral immunity. One important advantage in comparison to other immunosuppressive drugs – especially cortico- steroids – is that it does not cause significant myelosuppression or suppress nonspecific immunity. Figure 45.2 Mechanism of action of cyclosporine. Cyclosporine enters the cytosol, where it binds and inhibits calcineurin at a specific receptor site. By inhibiting calcineurin, the movement of the nuclear factor of activated T cells (NFAT) is prevented from entering the nucleus. Without the action of NFAT, transcription factors that produce cytokines is inhibited. (See text for additional details.) Tacrolimus is also capable of inhibiting calcineurin, but at a different binding site. Cyclosporine has been used for a number of diseases in veterinary medicine and since the introduction into veterinary medicine over 10 years ago, the number of potential uses, particularly in dogs and cats, has increased substantially. Many of these diseases have been dermatological, as reviewed by Robson and Burton (2003). In dogs, when used in the treatment of perianal fistulas, (Mathews and Sukhiani, 1997; Griffiths et al., 1999) an 85% healing rate was found in one study (Mathews et al., 1997) (2.5–6 mg/kg/day); in sebaceous adenitis, good response was reported in one case (Carothers et al., 1991); and in idiopathic sterile nodular panniculitis, excellent results were seen in two reported cases, which were followed for 6 months following discontinuance of the cyclosporine (Guaguère, 2000). Cyclosporine has been used for treatment of a variety of immune-mediated diseases that include IMHA, inflammatory bowel disease (IBD) (Allenspach et al., 2006), immune-mediated polyarthritis (Rhoades et al., 2016), and aplastic anemia (AA). For some diseases there is only anecdotal accounts of successful outcomes with cyclosporine, and for others (e.g., IMHA) the evidence is of such poor quality that conclusions cannot be made. For treating immune-mediated skin diseases, the results from pilot studies for treating pemphigus foliaceous in dogs have been disappointing (Rosenkrantz et al., 1989). It did not help any patients with mycosis fungoides. In a study in which five dogs with pemphigus foliaceous were treated, there was little benefit (Olivry et al., 2003a). The dogs with pemphigus foliaceous were initially treated with the “atopy dose” of 5 mg/kg/day. If there was no response the dose was increased to 10 mg/kg/day. At the end of the trial, it was concluded that at 5–10 mg/kg/day cyclosporine was unable to produce complete remission in any of the five dogs. In a case study of immune-mediated polyarthritis in dogs (Clements et al., 2004), dogs were treated with prednisolone and various other immune-modifying drugs. Three dogs treated with cyclosporine at 5 mg/kg per day did not respond. In people it has been used successfully for treatment of atopic dermatitis (Camp et al., 1993). Because of this efficacy, the use of cyclosporine for treating canine atopic dermatitis was initially investigated in a pilot study of 14 dogs (Fontaine and Olivry, 2001), and subsequently in larger controlled studies (Olivry et al., 2002a, 2002b). The evidence for recommending cyclosporine was summarized by a task force (Marsella and Olivry, 2001) and an evidence-based review (Olivry et al., 2003b). In another trial of 30 dogs treated with cyclosporine (5 mg/kg/day) or prednisolone (0.5 mg/kg/day), the efficacy of cyclosporine was not statistically different than prednisolone (Olivry et al., 2002a). Reductions in mean lesion scores were 60% and 59% in prednisolone and cyclosporine groups, respectively. Reduction in mean pruritus scores were 81% and 78% in prednisolone and cyclosporine groups, respectively. In another published study from the same author (Olivry et al., 2002b), 91 dogs with atopic dermatitis were treated with cyclosporine and compared to placebo. Two doses were examined, 2.5 mg/kg/day and 5 mg/kg/day. There was a dose-dependent effect. Dogs treated with 5 mg/kg had statistically significant reduction in pruritus scores (45%) and skin lesions (67%). The dose of 2.5 mg/kg was not statistically different from placebo. Some dogs may benefit from less than once-daily administration, as shown in another study (Steffan et al., 2003). In this study, administration of cyclosporine (5 mg/kg) was compared to methylprednisolone (0.75 mg/kg) for treatment of canine atopic dermatitis. The response was not different between the drugs, but there was an overall better assessment of efficacy and more gastrointestinal problems in dogs treated with cyclosporine. In the cyclosporine-treated dogs, the dose was started at 5 mg/kg/day for 4 weeks, but eventually half of the dogs were adjusted to an EOD dose, and one-quarter of the dogs were given cyclosporine at 5 mg/kg only twice a week. In August 2011, a cyclosporine oral solution (Atopia) was the first drug approved by the FDA for treating allergic dermatitis in cats. Capsules also can be used and one 25-mg capsule has been used for a dose of 25 mg/cat, once daily. The FDA-approved dose is 7 mg/kg per day, which is higher than the canine dose. Higher doses are needed because of the more variable absorption in cats compared to dogs. Other uses for cats include immunosuppression for kidney transplantation (Mathews and Gregory, 1997) at a dose of 3–4 mg/kg q 12 h. It also has been used to treat inflammatory diseases in cats, including stomatitis and eosinophilia granuloma complex (Guaguère and Prèlaud, 2000; Vercelli et al., 2006). For treatment of pemphigus foliaceous in cats it was more effective than in dogs. At a dose of 5 mg/kg per day, it was effective in some cats and steroid sparing (Irwin et al., 2012). The pharmacokinetics of cyclosporine are complicated because of the differences between dosage forms, presence of many metabolites, influence of drug interactions, and variability in oral absorption. The formulation called Sandimmune was used for many years but was absorbed inconsistently and is not used today. The common human formulation is a microemulsion called Neoral, which has a much more consistent rate and extent of absorption that is less affected by factors such as feeding than Sandimmune (Lown et al., 1997). There are now over 20 human generic formulations of this formulation. There is no evidence available to show that the human generic formulations are bioequivalent to the brand-name product in dogs and cats. The introduction in late 2003 of Atopica represents the first oral veterinary formulation of cyclosporine. The formulation is exactly the same as Neoral microemulsion (absorption, kinetics, and dissolution), except that there is a greater variety of capsule sizes available (10, 25, 50, and 100 mg) for veterinary use. Compounded formulations can have variable strength and are not recommended for clinical use in dogs and cats. The pharmacokinetics of cyclosporine in treated animals has been examined (Steffan et al., 2004). Cyclosporine is metabolized in the intestine and liver. Twenty-five to thirty metabolites have been identified. The prehepatic intestinal enzymes account for significant metabolism of cyclosporine (Wu et al., 1995), and systemic absorption in dogs is only 20–30% (Myre et al., 1991). Systemic absorption in cats is similar at 25–29% (Mehl et al., 2003). The intestinal metabolism by cytochrome P450 enzymes (CYP) and the efflux caused by intestinal p-glycoprotein (P-gp) account for most of the loss in systemic availability after oral administration. Drug enzyme inhibitors can inhibit the presystemic metabolism and produce an increase in systemic availability of cyclosporine (see Section Drug Interaction). For example, 5–10 mg/kg of ketoconazole once daily can decrease the dose of cyclosporine because clearance is reduced by 85% (Myre et al., 1991). As in the clinical trials cited in this chapter, the effective dose for treating dermatitis in dogs has been 5 mg/kg/day. There may be a delay in response when cyclosporine is initiated for as long as 4–6 weeks and some dermatologists administer corticosteroids for the first 2 weeks when initiating treatment. Some clinicians have been able to lower the dose, or administer the dose every other day, and still maintain clinical remission. Approximately half of treated dogs can be maintained by increasing the dose interval. Although clinical studies evaluating efficacy for treating immune-mediated diseases in dogs or cats are very limited, cyclosporine has been used for diseases listed in Sections Clinical Use in Dogs and Clinical Use in Cats. For these conditions it is generally assumed that higher doses are needed – generally in the range of 10 mg/kg every 12 hours oral. At these doses, cyclosporine consistently suppressed cytokine expression from activated T cells in dogs for a duration of 12 hours (Fellman et al., 2015). The same authors found that at a lower dose of 5 mg/kg once daily in dogs produced a more variable response and inconsistent suppression of activated T cells (Archer et al., 2011). For organ transplantation in people, doses of 5–10 mg/kg/day are used to achieve targeted whole blood concentrations of 150–400 ng/ml. In people, dosages are adjusted to meet the needs of the individual patient on the basis of clinical response and monitoring trough plasma concentrations. For organ transplantation in cats (Mathews and Gregory, 1997) a dose of 3 mg/kg q 12 h was used to achieve trough blood concentrations of 300–500 ng/ml. For organ transplant in dogs, 10 mg/kg q 12 h to achieve concentrations of 500–600 ng/ml has been used (Mathews et al., 2000). A report on treating perianal fistulas in dogs (Mathews and Sukhiani, 1997) found that an average dose of 6 mg/kg q 12 h was needed to achieve a targeted blood concentration of 400–600 ng/ml. However, this recommendation was later modified to 2.5–6 mg/kg/day. The most common dose for treating perianal fistulas is 3 mg/kg q 12 h. Steffan and colleagues (2004) found that when administering approved doses to dogs with atopic dermatitis, routine monitoring of blood cyclosporine concentrations was not necessary. When animals were administered a dose of 5 mg/kg/day, there did not appear to be a strong correlation between blood concentration achieved and clinical response. It is also difficult to interpret blood concentration monitoring results because no therapeutic range has been established for dogs and cats. Nevertheless, because of wide individual variation in cyclosporine pharmacokinetics, monitoring blood concentrations is sometimes used to determine if the lack of therapeutic response is caused by inadequate oral absorption, rapid metabolism, or poor compliance by the pet owner. Monitoring can sometimes be helpful to identify why patients may be nonresponders. Effective treatment has been reported, even when trough blood concentrations are low. However, according to one paper, clinical benefits have not been observed when trough concentrations have fallen below 50 ng/ml; therefore, blood testing of patients failing therapy was suggested to reveal inadequate dosing (Robson and Burton, 2003). One must be cognizant of the assay used when monitoring cyclosporine. Plasma values will be lower than whole-blood assays because as much as 50–60% and 10–20% of the dose is concentrated in erythrocytes and leukocytes, respectively. Nonspecific immunoassays will report higher values than a more specific (monoclonal or HPLC) assay. Despite the hypothesized higher specificity when using the monoclonal assay, important discrepancies between these assays and the more specific HPLC assay have been reported (Steimer, 1999). If trough samples are monitored (to detect the lowest point during the dose interval), samples are collected immediately before the next scheduled dose (Figure 45.3). However, trough concentrations can be low because of the short half-life and may not correlate with clinical response. Effective treatment has been reported, even when trough blood concentrations are low. If peak samples are monitored (to detect the maximum concentration) blood sample monitoring is done by collecting a 2-hour sample (C2), which correlates better with the area-under-the-curve (AUC) than the trough concentration (Figure 45.3). A 2-hour sample collected from dogs was able to detect the peak response, which correlated with immunosuppressive effects better than trough concentrations (Fellman et al., 2015). This confirmation in dogs agrees with evidence in people that a C2 blood sample value correlates better with clinical results than trough samples (Levy et al., 2002; Nashan et al., 2002). If monitoring is performed using a 2-hour sample, anticipate that the drug concentration at 2 hours will be approximately twice as high compared to the levels at 12 hours. The peak concentration to dose ratio in dogs is 179 ± 56. Therefore, at a dose of 5 mg/kg, the average peak concentration is 895 ng/mL with high variability of 31% coefficient of variation (Archer et al., 2014). Figure 45.3 Hypothetical blood concentration versus time curve for cyclosporine administration to animals. The peak concentration typically occurs at 2 hours in most animals and can be used to monitor peak effects. This concentration (C2) is correlated with the total area-under-the-curve (AUC) and is associated with pharmacodynamic effects (see text). If monitoring is performed by collecting a trough sample, there can be differences between the 12 hour sample (with twice-daily dosing) compared to the 24 hour sample (with once-daily dosing). Cyclosporine can cause vomiting, diarrhea, anorexia, secondary infections, and gingival hyperplasia (Table 45.1 ). Hyperplastic skin lesions have occasionally developed in dogs treated with cyclosporine (Favrot et al., 2005). Papillomavirus can be detected in some skin lesions. Tremors or shaking have been observed in dogs administered high doses. These events are rare and usually not a problem as long as doses are kept within accepted limits. Table 45.1 Adverse reactions to cyclosporine reported in animals The most common clinical problem with cyclosporine in dogs and cats that has been cited (Olivry et al., 2002a, 2002b, 2003b; Steffan et al., 2003; Heinrich et al., 2011), or detected in the trials conducted by the drug manufacturer (Novartis), is gastrointestinal problems. Vomiting, anorexia, and diarrhea are consistently the most common adverse effect reported, but in some patients may improve several days after initiating treatment. But, in some patients, it has led to discontinue the medication. When anorexia and vomiting are observed, veterinarians have tried various interventions such as lowering the dose, or administration of the dose with some food. Feeding will reduce the amount of cyclosporine absorbed in dogs by 15–20%, but it is unlikely that this decrease will be severe enough to affect efficacy. Keep in mind that some animals may refuse to eat their food if the drug is mixed in with their diet. Nephrotoxicity, once a problem with older forms, is rare with current formulations. Secondary malignancies because of decreased immune surveillance are a possible complication to long-term therapy but have not been reported in dogs or cats. Increased risk of toxoplasmosis has been a concern from cyclosporine administered to cats (Lappin et al., 2015). At a dose of 7.5 mg/kg/day (label dose), for an extended (long) interval, cyclosporine increased the severity of Toxoplasma gondii infection in naïve (seronegative) cats, but not in cats previously exposed (seropositive) to Toxoplasma gondii, and did not activate oocyst shedding in cats that had been previously exposed. Seronegative (naïve) cats may be at risk for developing clinical toxoplasmosis if they are infected while receiving cyclosporine treatment. Other safety concerns in cats were addressed by the manufacturer or in other studies (Roberts et al., 2014). Safety of cyclosporine in cats at high doses for 6 months was assessed, with the conclusion that it is safe and well tolerated. Glucose metabolism has been studied in dogs (Kovalik et al., 2011). Cyclosporine will negatively affect glucose homeostasis in some animals. The mechanism is via decreased insulin secretion and possibly insulin resistance, but the clinical significance is unclear. Cyclosporine may increase platelet hypercoagulability, which may be a concern when it is used to treat IMHA in dogs (this disease has been associated with thromboembolism). Cyclosporine administration increases thromboxane (TXA2), which can trigger platelet aggregation and vasoconstriction (Thomason et al., 2012, 2016). Administration of aspirin to dogs will prevent the cyclosporine-induced increase in TXA2 (Thomason et al., 2016). Several drugs may interact with cyclosporine. These drugs include ketoconazole, itraconazole, fluconazole, erythromycin, and diltiazem. All these drugs can potentially increase concentrations of cyclosporine in animals. For example, coadministration of ketoconazole to treat secondary fungal infections will substantially decrease metabolism of cyclosporine (Myre et al., 1991). Ketoconazole has been used deliberately to reduce the need for cyclosporine in some investigations (Patricelli et al., 2002) and doses have been reduced to one-third of the original dose when administered with ketoconazole. In one study with cyclosporine in the treatment of perianal fistulae, the dose of 1 mg/kg cyclosporine combined with 10 mg/kg ketoconazole was used. The author felt that a dose of 0.5 mg/kg combined with 10 mg/kg ketoconazole also could be used (Mouatt, 2002). In people, grapefruit juice will increase concentrations of cyclosporine because of inhibition of metabolizing enzymes. However, the dose of grapefruit required to produce a similar effect in dogs is very high (41 capsules) and not clinically practical (Hanley et al., 2010). Cyclosporine also is an inhibitor of one of the membrane transport proteins P-glycoprotein. It can inhibit transport of ivermectin and increase risk of ivermectin toxicity in dogs. Cyclosporine (Optimmune) is approved to increase tear production in dogs with keratoconjunctivitis sicca (KCS). Success rates are high. Topical cyclosporine has also been used to treat other immune-mediated diseases of the eye. It is available as a 0.2% ophthalmic ointment. Cyclosporine intraocular implants have been developed by an ophthalmologist at North Carolina State University (Gilger et al., 2006). These implants are administered to horses to treat anterior uveitis. This method of treatment slowly releases the medication and can produce a long-term sustained therapeutic effect.
Immunosuppressive Drugs
Glucocorticoids
Cellular Effects
Immunosuppressive Effects
Choice of Drugs
Doses
Adverse Effects
Rationale for Every-Other-Day Therapy
Cyclosporine
Clinical Use in Dogs
Immune-mediated Diseases
Atopic Dermatitis
Clinical Use in Cats
Formulations and Pharmacokinetics
Administration
Monitoring
Adverse Effects and Precautions
Cats: adverse events
Anorexia (most common)
Vomiting
Weight loss, depression
Dogs: adverse events
Vomiting (most common)
Diarrhea
Depression
Lethargy
Pruritus
Anorexia
Increased liver enzymes
Gingival hyperplasia
Drug Interactions
Ophthalmic Use.
Keratoconjunctivitis sicca:
Anterior uveitis:
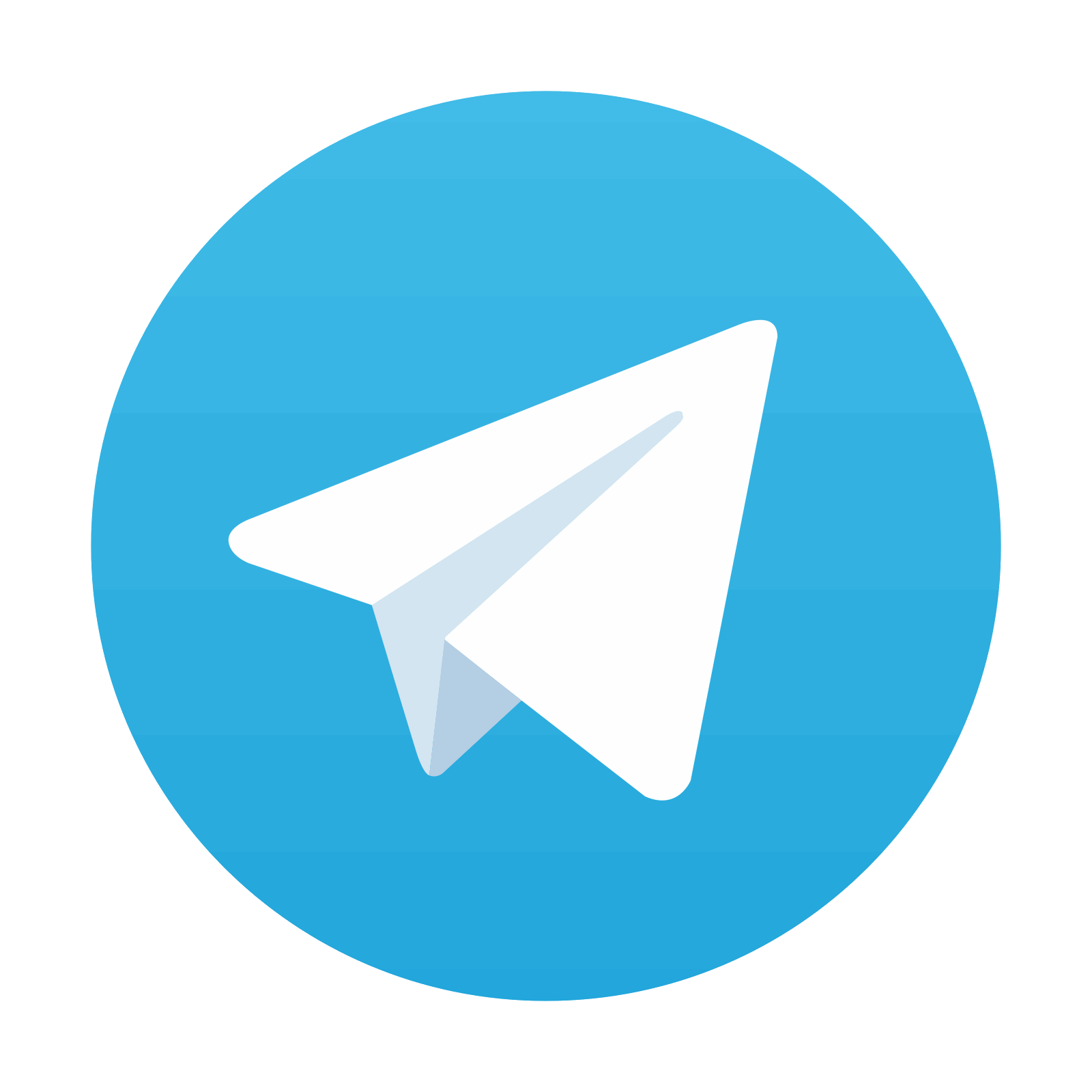
Stay updated, free articles. Join our Telegram channel

Full access? Get Clinical Tree
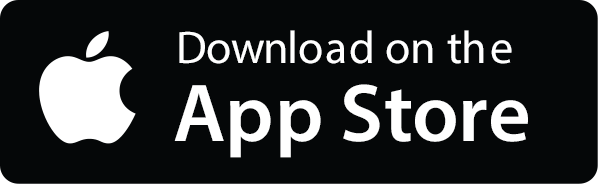
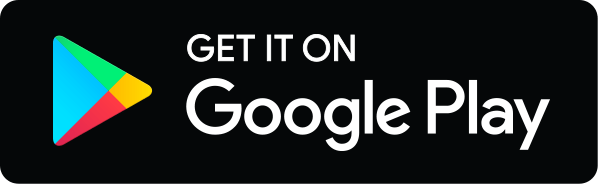