Chloë De Witte Helena Berlamont and Freddy Haesebrouck Helicobacters are non‐sporulating, fastidious, micro‐aerophilic Gram‐negative bacteria. To date, the genus Helicobacter consists of over 50 identified species (Table 19.1). Helicobacters can be roughly divided into two major groups: the gastric species colonizing the stomach and the enterohepatic species colonizing the liver and/or intestines of their host. Helicobacter pylori is the best‐studied and most prevalent Helicobacter species colonizing the human stomach. H. pylori infections have only occasionally been described in animals and this species is, therefore, not considered in detail in this chapter. The other, non‐H. pylori helicobacters (NHPH) colonize the gastrointestinal tract of several animal species, ranging from terrestrial and aquatic mammals to reptiles, amphibians, birds, rodents, and fish. Helicobacter colonization may lead to development of gastric and/or enterohepatic pathologies and some NHPH have zoonotic potential (Table 19.1). This chapter focuses on the pathogenesis of gastric and enterohepatic NHPH infections in domestic animals. A general overview of the pathogenesis of gastric Helicobacter infections is shown in Figure 19.1. Gastric helicobacters are able to survive the acidic environment of the stomach by expressing urease at a high level. The presence of flagella and genes encoding proteins involved in chemotactic behavior allow helicobacters to move actively away from low pH regions toward more neutral regions of the gastric mucosa (Haesebrouck et al. 2009). Once the helicobacters reach the inner mucus layer, their motility decreases, possibly optimizing their attachment to gastric epithelial cells. In contrast to H. pylori, most gastric NHPH are found deep in the gastric pits (Figure 19.2; Haesebrouck et al. 2009). Binding to mucins and epithelial cells is an important step in the pathogenesis of Helicobacter infections. The transmembrane Muc1 is constitutively expressed by gastric epithelial cells and most likely serves as a first point of adherence for Helicobacter. Experimental infection of BALB/c mice with H. heilmannii resulted in an increased expression of the secreted mucin Muc6 and the transmembrane mucin Muc13 in the first nine weeks post‐infection (Liu et al. 2014), indicating that both mucins might play a role in NHPH adhesion and colonization. Furthermore, H. suis has been shown to bind to acidic glycan structures and glycans terminating with galactose, while type 2 Lewis antigens and terminal α‐GalNAc most likely play a role in adhesion of gastric NHPH to the canine gastric mucosa (Amorim et al. 2014). Table 19.1 Helicobacter species and their pathogenic significance for humans and animals. Source: adapted from Haesebrouck et al. (2009). Figure 19.1 General overview of the pathogenesis of gastric Helicobacter infections in domestic animals. Dotted line: effect only shown in vitro and/or in vivo in some animal species. GGT, gamma‐glutamyl transpeptidase; MALT, mucosa‐associated lymphoid‐tissue; MUC, mucin; OMPs, outer membrane proteins; ROS, reactive oxygen species; Th, T‐helper cell; Treg, regulatory T‐cell; TLR, Toll‐like receptor. Figure 19.2 Immunohistochemical Helicobacter staining of a pig stomach, showing H. suis bacteria (brown) present in the glands of the antrum of a stomach of a H. suis‐positive pig. Gastric helicobacters harbor a large set of genes encoding outer‐membrane proteins (OMPs). Comparative genomics showed that gastric NHPH lack all known H. pylori adhesins described so far (Bauwens et al. 2018), suggesting that other OMPs function as adhesins in these organisms. Two Helicobacter‐specific OMP families with possible functions in adhesion (family 13, i.e. Hop, Hor and Hom; and family 33, Hof), as well as an outer membrane phospholipase (OMPLA; family 38) and an Helicobacter‐specific VacA‐like cytotoxin family correlated with colonization capacity (family X3) were identified in all gastric helicobacters (Bauwens et al. 2018). Two Hof proteins, HofE and HofF, have been shown to play a role in the adhesion of H. heilmannii to the gastric mucosa and gastric epithelial cells (Liu et al. 2016). The exact role of the other OMPs in NHPH colonization remains to be further elucidated (Bauwens et al. 2018). Cytotoxin‐associated gene pathogenicity island (cagPAI) and vacuolating cytotoxin A (VacA) are major H. pylori virulence factors involved in the induction of gastric pathologies. Both factors, however, are absent in all gastric NHPH, except for H. cetorum which carries a functional VacA toxin. Gamma‐glutamyl transpeptidase (GGT) is a highly conserved and important virulence factor of gastric Helicobacter species (Figure 19.1) This enzyme hydrolyses glutathione and glutamine into glutamate, after which glutamate supplies energy for the bacterium. Degradation of glutamine and glutathione may lead to a deficiency for the host, possibly initiating and/or promoting several pathologies. In vitro research using human gastric epithelial cells has shown that H. suis GGT causes apoptosis or necrosis, depending in part on the amount of reactive oxygen species generated through degradation of the antioxidant glutathione (Flahou et al. 2011). Oral supplementation of glutamine has been shown to temper H. suis induced gastritis and epithelial proliferation in experimentally infected Mongolian gerbils and naturally infected pigs (Zhang et al. 2015; De Bruyne et al. 2016). Gastric helicobacters also possess other virulence factors, such as flavodoxin protein (FldA), plasminogen‐binding proteins (PgbA, PgbB), L‐asparaginase II (AnsB), high temperature requirement A (HtrA), collagenase (PrtC) and IceA (Vermoote et al. 2011). For H. pylori, these factors have already been associated with the development of gastric lesions. While AnsB plays a role in the cell‐cycle inhibition of fibroblasts and gastric cell lines (Scotti et al. 2010), FldA has been associated with the pathogenesis of mucosa‐associated lymphoid tissue (MALT) lymphomas (Chang et al. 1999). PgbA and PgbB allow H. pylori to bind to host plasminogen, which may contribute to a delay of the natural healing process of gastric ulcers (Vermoote et al. 2011). HtrA is involved in cleavage of E‐cadherin and thereby contributes to the disruption of the gastric epithelial barrier (Hoy et al. 2010). Collagen becomes degraded through the production of PrtC, (Kavermann et al. 2003). Finally, IceA has been associated with peptic ulcer disease and seems to be induced by contact between epithelial cells and H. pylori (Huang et al. 2016). However, the possible role of these virulence factors in the development of gastric pathologies associated with NHPH infections remains unclear. Canine and feline gastric helicobacters possess a unique gene encoding chondroitinase AC‐type lyase belonging to CAZy family 8 (Namburi et al. 2016). Most likely, this enzyme breaks down chondroitin‐4‐O‐sulfate secreted by chief peptic cells, and since chondroitin‐4‐O‐sulfate regulates the activity of pepsin in the chief cells, its depletion might cause decreased peptic control resulting in ulcer development. It remains, however, unclear how this enzyme can participate in the promotion of gastric disorders in dogs and cats (Namburi et al. 2016). Despite the presence of a host immune response, helicobacters are generally not cleared from the stomach, resulting in lifelong infections. H. pylori has been shown to avoid Toll‐like receptor (TLR) recognition by modulating its surface molecules, such as lipopolysaccharide (LPS) and flagellin. Variation in LPS sialyation patterns may explain why TLR2‐mediated nuclear factor κB (NF‐κB)‐inducing abilities differ between H. bizzozeronii strains (Kondadi et al. 2015). The SfpA/LpxR OMP family (i.e. family 36) has been found in all gastric helicobacters and is possibly involved in LPS modulation by removing the 3′‐acyloxyacyl group of lipid A (Bauwens et al. 2018). Furthermore, all gastric helicobacters show the presence of the jhp0562 glycosyltransferase enzyme, except for H. ailurogastricus. The glycosyltransferase enzyme jhp0562 of H. pylori functions in the synthesis of both type I and type II Lewis antigens, which are present on the LPS of the bacterial outer membrane. Diverse Lewis antigens are generated via intragenomic recombination of jhp0562, and this glycosyltransferase contributes to the process of LPS variation in H. pylori. Finally, the presence of genes involved in sialic acid biosynthesis (neuA, neuB and wecB) may also contribute to host immune evasion by decorating the bacterial surface with sialic acid. As also described for H. pylori, H. suis GGT has been identified to inhibit proliferation and cytokine production of lymphocytes in vitro through deprivation of extracellular glutamine, a conditional essential amino acid important for normal cell function and maintenance, and through hydrolysis of the antioxidant glutathione (Zhang et al. 2013, 2015). In this same study, H. suis outer‐membrane vesicles were identified as a possible delivery route of H. suis GGT to lymphocytes residing in the deeper mucosal layers (Zhang et al. 2013, 2015). Other proteins associated with immune‐evasion mechanisms have been found in gastric helicobacters, such as neutrophil‐activating protein A (NapA), catalase (KatA), superoxide dismutase and alkyl hydroperoxide reductase (Vermoote et al. 2011; Joosten et al. 2015). These enzymes have been described to protect H. pylori during acute inflammation since they lower the amount and impact of reactive oxygen species produced by phagocytes. Most likely, they are also involved in the persistence of gastric NHPH, although this needs to be investigated. H. mustelae produces a unique surface array showed that colonization levels of Hsr‐negative mutants were similar to those of the parent strain up to 9 weeks after infection but were significantly reduced 12–18 weeks after infection (Patterson et al. 2003). These findings indicate that Hsr impacts long‐term survival of H. mustelae, most likely due to the variability of exposed of Hsr epitopes which may contribute to immune evasion (Forester et al. 2001; Patterson et al. 2003). Dendritic cells might also play a crucial role in promoting tolerance to gastric Helicobacter infections. H. pylori has developed several strategies to influence dendritic cell maturation and cytokine production, skewing them toward a tolerogenic phenotype and thereby directing a regulatory T cell (Treg) response. By eliciting a Treg response, H. pylori succeeds in suppressing gastric immune and inflammatory responses, leading to chronic colonization (Mejías‐Luque and Gerhard 2017). Bosschem et al. (2017) demonstrated that H. suis induces a semi‐maturation of porcine monocyte‐derived dendritic cells, which in turn may elicit Treg expansion. Since H. pylori urease impairs folding and correct transport of MHC II molecules by binding to CD74 and since this enzyme is highly conserved among gastric helicobacters (Beswick et al. 2006), H. suis urease may play a similar role in inducing a Treg immune response. Finally, it has been shown that H. suis infection can change the mucin composition and glycosylation of the porcine gastric mucus, which in turn decreases the amount of H. suis binding glycan structures (Padra et al. 2019). Furthermore, the H. suis growth‐inhibiting effect of mucins from non‐infected pigs was replaced by a growth‐enhancing effect by mucins from infected pigs. It was hypothesized that H. suis infections impair the mucus barrier by decreasing both the bacteria‐binding ability of the mucins and the innate anti‐proliferation activity of mucus on H. suis. Inhibition of these mucus‐based defenses may create a more stable and inhabitable niche for H. suis, which may contribute to lifelong infections (Padra et al. 2019). The inflammatory response toward gastric Helicobacter infections plays an important part in the pathogenesis. Chronic inflammation has been associated with epithelial proliferation, metaplasia and/or atrophy, which contribute to the development of gastric cancer. Once gastric helicobacters penetrate the gastric epithelial barrier, innate host defense mechanisms are triggered and the expression of proinflammatory and anti‐bacterial factors by gastric epithelial cells is stimulated. This leads to the recruitment of neutrophils, monocytes/macrophages and dendritic cells, which infiltrate the gastric mucosa. The secretion of mediators such as histamine, proteases, adenosine and H2O2 from neutrophils and mast cells can modify the barrier and/or ion transport function of epithelial cells. Oxidative metabolites from neutrophils can also induce cellular damage, including apoptosis and DNA injury. The longer the period of exposure of a tissue to activated immune/inflammatory cells and mediators, the more cellular damage may accumulate (Ernst and Gold 2000). In response to Helicobacter antigens, dendritic cells and macrophages further amplify the inflammatory response by the production of cytokines, resulting in an adaptive immune response (Wilson and Crabtree 2007). While H. pylori and H. felis mainly induce a T helper (Th) 1/Th17 response, other gastric NHPH seem to induce a Th2/Th17 response, although this depends on the host, which mainly determines the outcome of the immune response (Bosschem et al. 2016). While coinciding Treg responses contribute to the persistent infection of gastric helicobacters infections, Th1‐driven host responses contribute to gastroduodenal disease (Ernst and Gold 2000). Gastric Helicobacter
19
Helicobacter
Introduction
Gastric Helicobacters
Virulence Mechanisms of Gastric Helicobacters Involved in Adhesion and Colonization
Taxon
Natural hosts
Zoonotic potential
Disease associations
References
Gastric Helicobacter spp.
“Candidatus H. bovis”
Cattle
Yes
Asymptomatic, gastritis
De Groote et al. (1999), Haesebrouck et al. (2009)
“Candidatus H. homininae”
Chimpanzee, gorilla
Unknown
Unknown
Flahou et al. (2014)
H. acinonychis
Cheetah, tiger, lion
Unknown
Asymptomatic, chronic gastritis, gastric ulcers
Haesebrouck et al. (2009), Tegtmeyer et al. (2013)
H. ailurogastricus
Cat
Unknown
Unknown
Haesebrouck et al. (2009), Joosten et al. (2015)
H. baculiformis
Cat
Unknown
Unknown
Haesebrouck et al. (2009)
H. bizzozeronii
Cat, dog, rabbit
Yes
Asymptomatic, gastritis, peptic ulcers, MALT‐lymphoma
Haesebrouck et al. (2009)
H. cetorum
Cetaceans, pinnipeds
Yes
Asymptomatic, gastritis, peptic ulcers
Harper et al. (2002), Goldman et al. (2011)
H. cynogastricus
Dog
Unknown
Unknown
Haesebrouck et al. (2009)
H. felis
Dog, cat, cheetah, New Guinea wild dog, rabbit
Yes
Asymptomatic, gastritis, peptic ulcers, MALT‐lymphoma
Haesebrouck et al. (2009)
H. heilmannii
Dog, cat, cheetah, bobcat, tiger, lynx, leopard, puma
Yes
Asymptomatic, gastritis, peptic ulcers, MALT‐lymphoma
Haesebrouck et al. (2009)
H. mustelae
Ferret
Unknown
Asymptomatic, gastritis, peptic ulcers, gastric cancer, MALT‐lymphoma
Haesebrouck et al. (2009)
H. pylori
Human
Asymptomatic, gastritis, peptic ulcers, gastric cancer, MALT‐lymphoma
Haesebrouck et al. (2009)
H. salomonis
Cat, dog, rabbit
Yes
Asymptomatic, gastritis, peptic ulcers, MALT‐lymphoma
Haesebrouck et al. (2009)
H. suis
Pig, mandrill monkey, rhesus macaque, crab‐eating macaque
Yes
Asymptomatic, gastritis, peptic ulcers, MALT‐lymphoma
Haesebrouck et al. (2009), De Witte et al. (2019)
Enterohepatic Helicobacter spp.
“Candidatus H. colifelis”
Cat
Unknown
Unknown
Foley et al. (1998)
H. anseris
Goose
Unknown
Unknown
Schauer (2001)
H. apri
Wild boar
Unknown
Unknown
Zanoni et al. (2016)
H. aurati
Hamster
Unknown
Asymptomatic, chronic gastritis, intestinal inflammation and metaplasia
Zenner (1999), Patterson et al. (2000)
H. bilis
Mouse, rat, gerbil, hamster, dog, cat, sheep, pig
Yes
Asymptomatic, hepatic and intestinal disease
Zenner (1999), Haesebrouck et al. (2009)
H. brantae
Goose
Unknown
Unknown
Schauer (2001)
H. callitrichis
Marmoset
Unknown
Asymptomatic, colitis, hepatitis, intestinal adenocarcinoma
Marini et al. (2010)
H. canadensis
Bird, pig, primates
Yes
Unknown
Schauer (2001), Flahou et al. (2014)
H. canicola
Dog
Unknown
Unknown
Rossi et al. (2008)
H. canis
Dog, cat, sheep
Yes
Asymptomatic, diarrhea, hepatitis, gastroenteritis
Schauer (2001), Swennes et al. (2014)
“Candidatus H. burdigaliensis”
Human
/
Unknown
Berthenet et al. (2019)
H. cholecystus
Hamster
Unknown
Asymptomatic, cholangiofibrosis, pancreatitis
Whary and Fox (2004)
H. cinaedi
Human, hamster, rat, cat, dog, rhesus monkey, primates
Yes
Asymptomatic, hepatic and intestinal disease
Whary and Fox (2004), Rossi et al. (2008), Marini et al. (2010), Flahou et al. (2014)
H. equorum
Horse
Unknown
Unknown
Moyaert et al. (2009)
H. fennelliae
Human, dog, primates
Unknown
Asymptomatic, enteritis, proctitis, proctocolitis
Rossi et al. (2008), Flahou et al. (2014)
H. ganmani
Mouse, primates
Yes
Unknown
Whary and Fox (2004), Flahou et al. (2014)
H. hepaticus
Mouse, gerbil
Yes
Asymptomatic; hepatic, mammary and gastro‐intestinal inflammation and dysplasia
Whary and Fox (2004)
H. himalayensis
Marmot
Unknown
Unknown
Hu et al. (2015)
H. jaachi
Marmoset
Unknown
Asymptomatic, colitis, hepatitis, intestinal adenocarcinoma
Marini et al. (2010)
H. japonicum
Mouse
Unknown
Asymptomatic, typhlocolitis, lower bowel carcinoma
Shen et al. (2016)
“Candidatus H. labacensis”
Red fox
Unknown
Unknown
Gruntar et al. (2020)
“Candidatus H. labetoulli”
Human
/
Unknown
Berthenet et al. (2019)
H. macacae
Rhesus monkey, baboon
Unknown
Asymptomatic, colitis, hepatitis, intestinal adenocarcinoma
Marini et al. (2010), Flahou et al. (2014)
H. marmotae
Woodchuck, cat
Unknown
Asymptomatic, hepatitis, typhlocolitis
Whary and Fox (2004)
H. magdeburgensis
Mouse
Unknown
Unknown
Whary and Fox (2004)
H. mastomyrinus
Rodents, primates
Unknown
Asymptomatic, ulcerative typhlocolitis
Shen et al. (2005), Flahou et al. (2014)
“Candidatus H. meihli”
Red fox
Unknown
Unknown
Gruntar et al. (2020)
H. mesocricetorum
Hamster
Unknown
Unknown
Whary and Fox (2004)
H. muricola
Wild mouse
Unknown
Asymptomatic, hepatic and intestinal disease
Whary and Fox (2004)
H. muridarum
Mouse, rat
Unknown
Asymptomatic, gastritis, hepatic and intestinal disease
Queiroz et al. (1992), Whary and Fox (2004)
H. pamatensis
Bird, pig, cat
Yes
Unknown
Schauer (2001), Rossi et al. (2008)
H. pullorum
Poultry, parrot
Yes
Asymptomatic, hepatitis, diarrhea, enteritis
Harbour and Sutton (2008)
H. rodentium
Mouse, rat, primates
Unknown
Asymptomatic, hepatic and intestinal disease
Whary and Fox (2004), Flahou et al. (2014)
H. saguini
Cotton‐top tamarin
Unknown
Asymptomatic, ulcerative colitis, typhlocolitis and dysplasia
Marini et al. (2010)
H. suncus
House musk shrew
Unknown
Asymptomatic, gastritis
Whary and Fox (2004)
H. trogontum
Rat, pig, sheep
Unknown
Asymptomatic, hepatic and intestinal disease
Whary and Fox (2004), Inglis et al. (2006), Swennes et al. (2014)
H. typhlonius
Mouse, rat, primates
Unknown
Asymptomatic, hepatic and intestinal disease
Whary and Fox (2004), Flahou et al. (2014)
H. valdiviensis
Wild birds
Yes
Asymptomatic, diarrhea
Schauer (2001)
“Candidatus H. vulpis”
Red fox
Unknown
Unknown
Gruntar et al. (2020)
Virulence Mechanisms of Gastric Helicobacters Involved in the Induction of Gastric Pathologies
Virulence Mechanisms of Gastric Helicobacters Involved in Host Immune Evasion
Host Immune Response to Gastric Helicobacters and their Involvement in Pathogenesis
Stay updated, free articles. Join our Telegram channel

Full access? Get Clinical Tree
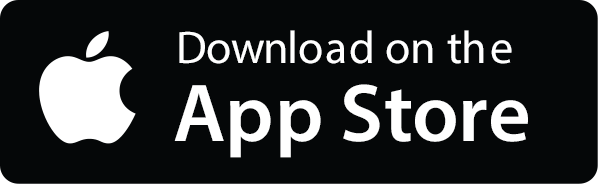
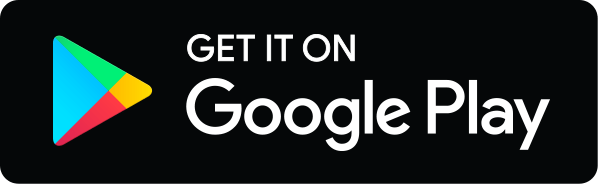