Chapter 9 Glaucoma
Clinical Anatomy and Physiology
Aqueous Humor Production and Outflow
Aqueous humor provides nutrition to the avascular cornea, trabecular meshworks, and lens. Knowledge of aqueous humor formation and the structure of the aqueous humor drainage apparatus are critical to understanding glaucoma in horses. Aqueous humor is formed primarily by active secretion through the ciliary body epithelia into the posterior chamber, utilizing energy and the enzyme, carbonic anhydrase (see Chapter 6, Diseases of the Uvea, for more information). A small portion of aqueous humor production arises from ultrafiltration of blood in the ciliary body circulation. Aqueous humor then flows through the pupil into the anterior chamber and finally exits through the highly specialized tissues of the iridocorneal angle (ICA)—the conventional outflow pathway—or through the more primitive outflow pathways of the iris, ciliary body, and sclera—the unconventional outflow pathway. The ICA or ciliary cleft is bordered anteriorly by the peripheral cornea and perilimbal sclera and posteriorly by the peripheral iris and ciliary body. Stout pectinate ligaments separate the anterior chamber from the ICA in the equine eye, and these are visible at the limbus in many horses.1–3
Aqueous humor flows between the pectinate ligaments into and through the cell-lined trabecular beams of the uveal trabecular meshwork (UTM), the corneoscleral trabecular meshwork (CSTM), the angular aqueous plexus (AAP), and then the intrascleral plexus (ISP) in the conventional outflow pathway (Figs. 9-1 and 9-2). The aqueous humor then drains from the ISP into the vortex veins that empty into the choroid. The UTM, CSTM, and AAP compose 74.3%, 21.5%, and 4.2% of the equine ICA area, respectively.1–3
Resistance to outflow is focused at the AAP and ISP in the conventional outflow system of the horse. The intertrabecular spaces of the UTM are very wide, and the spaces of the CSTM are very narrow. The CSTM acts as a sieve or filter for large particles, but it may or may not restrict aqueous flow directly. The AAP is a plexus of radially oriented, narrow-diameter vessels. The ISP consists of an extensive network of anastomosing circumferential channels that drain aqueous humor to the vortex venous system (Fig. 9-3). The ISP and vortex systems have a rich collateralization. Aqueous humor thus follows a path of low resistance in the UTM and CSTM, high resistance to outflow in the narrow tributaries of the AAP and ISP, and low resistance to outflow again in the vortex systems.1–3
Microsphere perfusion studies have shown the suprachoroidal and supraciliary spaces provide an extensive unconventional outflow pathway to the sclera and choroid in the horse (Fig. 9-4). The intertrabecular spaces of the equine ICA provide a large area of aqueous humor contact with the iris and ciliary body. Aqueous humor can enter the anterior iris face to drain into the vortex veins, and it also leaves the UTM through the interstitial spaces of the ciliary body musculature to drain into the supraciliary and suprachoroidal spaces and choroid.1–3
Retinal Ganglion Cells
The horse has a very large retinal sensory surface area, vast numbers of photoreceptors, a massive population of retinal ganglion cells (RGCs) with large cell bodies, a large optic disc area and optic nerve cross-sectional area (15.7 mm2 versus 5.17 mm2 in humans), high total optic nerve axon counts (1.076 × 106 versus 1.159 × 106 in humans), large mean individual optic nerve axon cross-sectional areas (3.11 mm2 versus 1.75 mm2 in humans), and 4.4 times the percentage of optic nerve axons larger than 2 mm in diameter compared with humans (35.5% in horses versus 8% in humans).4 The comparatively low density of axons in the horse optic nerve (62,800 axons/mm2 in horses versus 175,000 axons/mm2 in humans) undoubtedly reflects a low tissue density of RGCs, because the ratio of ganglion cells to optic nerve axons is 1:1 (Figs. 9-5 and 9-6). The marked proportion of large-diameter ganglion cells in the horse retina may be an adaptation to cover a large retinal sensory surface area with a relatively low population density of ganglion cells.4
Optic Nerve and Optic Nerve Head Cupping
RGC axons of the retinal nerve fiber layer become arranged into optic nerve fiber bundles by astrocytes in the choroidal lamina cribrosa. The scleral lamina cribrosa is a specialized extracellular matrix of the central nervous system that spans the scleral canal as a complex, multilayered set of collagenous plates (Fig. 9-7).5 The multiple plates of the scleral lamina cribrosa contain a hydrodynamic extracellular matrix of elastic and collagen fibers (types I, III, and VI), astrocytes, and capillaries (Fig. 9-8).
The prelaminar optic nerve is known as the optic disc, optic nerve head (ONH), or optic papilla and is surrounded by the white peripapillary scleral ring of Elschnig noted at the 6 o’clock position in the horse ONH. Myelin extends anterior to the equine lamina cribrosa to cover the surface of the optic disc. The myelinated axons generally stop at the edge of the scleral canal, although myelinated axons in the retinal nerve fiber layer are noted in the horizontal retinal quadrants of some horses. Scanning laser ophthalmoscopy of the equine ONH indicates that the neuroretinal rim area is smallest at the superior and inferior rims of the horse optic disc and larger at the nasal and temporal rims (Fig. 9-9). The intrapapillary region of the ONH is the area inside Bruch’s membrane at the scleral canal and consists of the neuroretinal rim and the optic cup (Figs. 9-10 and 9-11).
ONH cup enlargement or “cupping” is associated with advanced glaucoma in the horse (Fig. 9-12) and occurs as a result of axonal loss, laminar plate compression (Fig. 9-13), rotation of the scleral insertion zone posteriorly, outward bowing of the lamina cribrosa, and a widening of the scleral canal behind Bruch’s membrane. The associated enlargement of the ONH cup with these laminar and axonal changes is unique to glaucoma and not found in other optic neuropathies. The ratio of the cup-to-disc area is used to evaluate progression of glaucomatous optic nerve damage in humans. Enlargement of the cup-to-disc ratio indicates optic nerve axonal loss and is associated with deterioration in visual fields.
The intralaminar optic nerve of horses with glaucoma contains a significantly larger total number of laminar pores, pores of significantly smaller individual area, and pores that are rounder than those in the intralaminar optic nerve of healthy horses.5 The intralaminar optic nerves of horses with glaucoma contain a higher percentage of connective tissue (74%) than the optic nerves of healthy horses (67%). These differences may represent an anatomic variation found in horses predisposed to develop glaucoma, as well as changes resulting from IOP-induced radial stress forces causing stretching of the scleral lamina cribrosa.
The scleral lamina cribrosa is a transition zone of high to low hydrostatic tissue pressure caused by the prominent pressure gradient formed by the IOP and intraorbital pressure. The axoplasmic flow in the optic nerve axons is subjected to this abrupt change in tissue pressure.6 The elevated IOP found in glaucoma exacerbates this pressure gradient and is associated with posterior displacement of the lamina cribrosa and disruption of optic nerve axon axoplasmic flow (see Figs. 9-11, 9-12, and 9-13). Disruption of axoplasmic flow may be exacerbated by the sharp edge of Bruch’s membrane at the edge of the scleral canal. The lamina cribrosa is thus an active and compliant structure in the horse; its movement during normal and abnormal fluctuations in IOP can both protect and obstruct optic nerve axoplasmic flow.5
Intraocular Pressure
IOP measured with a Tono-Pen applanation tonometer (Reichert, Inc, Depew, NY) in normal horses had a mean of 23.3 ± 6.9 mm Hg with a range from 7 to 37 mm Hg in one study (Fig. 9-14).7 Other studies have identified a similar normal range.8–11 Rebound tonometers appear to provide accurate estimate of IOP in horses, although values tend to be 1 mm Hg higher with the rebound compared to the applanation tonometer (Fig. 9-15).11 The TonoVet rebound tonometer (Icare Finland OY, Helsinki, Finland) may be easier to use in horses than the Tono-Pen applanation tonometer. No topical anesthetic is needed with the TonoVet, and the TonoVet instrument is held quietly relative to the Tono-Pen (Fig. 9-16).
A consistent technique for measuring IOP should be utilized in the horse. The IOP should be measured with the same amount of sedation, the use of a motor lid nerve block, touching the same area of the cornea, by the same person, and at the same time of day. Failure to use auriculopalpebral nerve blocks during tonometry may result in slight overestimates of IOP and is recommended in most horses but especially fractious ones. Horses who require sedation for ocular examination may show dramatic decreases in IOP, as illustrated by a study in which xylazine decreased IOP by 23% to 27%.12 Head position effects IOP in horses such that IOP is increased when measured with the head below the heart, as with heavy sedation.13 Head position should be consistent between consecutive IOP measurements to prevent variation in IOP caused by head position alone. Although the horse does not seem to experience significant diurnal changes in IOP, in one study the IOP in the afternoon was slightly higher than the IOP in the morning.12 Horses appear more tolerant of ocular hypertension than other species. Some anecdotal reports indicate that IOP can remain high (>50 mm Hg) for several days and horses can retain vision, unlike dogs and other species. See Chapter 1 for more information regarding tonometry and tonography in the horse.
Pathophysiology of Glaucoma
Pathologic elevation of IOP is always an outflow obstructive phenomenon. Physical obstruction of the aqueous humor outflow pathways can occur as result of contraction of pre-iridal postinflammatory membranes, obstruction of the ICA with inflammatory debris, and posterior synechia causing pupillary block, iris bombe, and trabecular compression and angle closure.14 Postinflammatory uveal atrophy may predispose the ICA to collapse. Anterior lens luxation and vitreal prolapse appear common in Appaloosas and Rocky Mountain horses and may obstruct the pupil or the ICA. ICA obstruction by melanoma or other tumor cells can also occur.14,15 Infectious endophthalmitis can cause secondary glaucoma.
IOP-induced compressive conformational distortion within the scleral lamina cribrosa in glaucoma results in rotation, misalignment, and collapse of the laminar pores and laminar channels such that optic nerve axoplasmic flow is reduced and eventually blocked to cause RGC death. Glaucoma in the horse results in extensive and diffuse optic nerve damage, and the optic nerve axon density in horses with glaucoma is reduced by 65%.4 Gliosis is dramatic, with primarily glial cells remaining in the severely atrophied optic nerves. The median individual optic nerve axon cross-sectional areas were smaller in glaucomatous eyes than in normal eyes (1.60 mm2 versus 1.35 mm2), indicating that horses with glaucoma lose optic nerve axons of large individual cross-sectional area more rapidly than medium- and small-sized axons.4 Axons of all sizes are eventually injured by elevated IOP, but some RGCs and their axons appear to be IOP resistant; vision can often be retained for substantial periods in horses, despite extreme elevations in IOP.
Diagnosis and Clinical Signs of Glaucoma
Types of Glaucoma
The glaucomas are a group of diseases resulting from reductions in aqueous humor outflow that cause an IOP increase above that which is compatible with normal function of the retina and optic nerve. All glaucomas consist of five stages: (1) an initial event or series of events that progressively reduce function of the aqueous humor outflow system, (2) morphologic alterations of the aqueous outflow system that eventually lead to obstruction of aqueous humor outflow and IOP elevation, (3) elevated IOP that is too high for normal RGC and optic nerve axon function, (4) RGC and optic nerve axon degeneration, and (5) progressive visual deterioration that eventually leads to blindness.16
True glaucoma was once thought to be a variant of ERU and an uncommon ocular problem in horses. Glaucoma is still an infrequently diagnosed disease in the horse. This is due in part to the limited availability of tonometers in equine practice but also to the fact that diurnal fluctuations in IOP, even in chronic ERU cases, may make documentation of elevated IOP difficult. Equine glaucoma may not be easily recognized in the early stages of the disease because of the subtle nature of the clinical signs. This may be explained by the prominent role of uveoscleral aqueous humor outflow in the horse, which may be higher than that in other species, and by microanatomic differences in the aqueous humor outflow pathways in horses compared with those in other species.1–3
Equine glaucoma is frequently categorized into primary, secondary, and congenital types. The terms may not be completely relevant, because all glaucomas are secondary to some causative mechanism. Primary glaucomas have a bilateral and heritable potential, have no overt ocular abnormality to account for the increase in IOP, and have not been conclusively reported in the horse. Secondary glaucomas in horses have an identifiable cause such as iridocyclitis, lens luxation, or intraocular neoplasia. Iris and ciliary body neoplasms can obstruct aqueous humor outflow to cause secondary glaucoma. Congenital glaucoma caused by developmental anomalies of the ICA (goniodysgenesis) has been reported in foals (Box 9-1
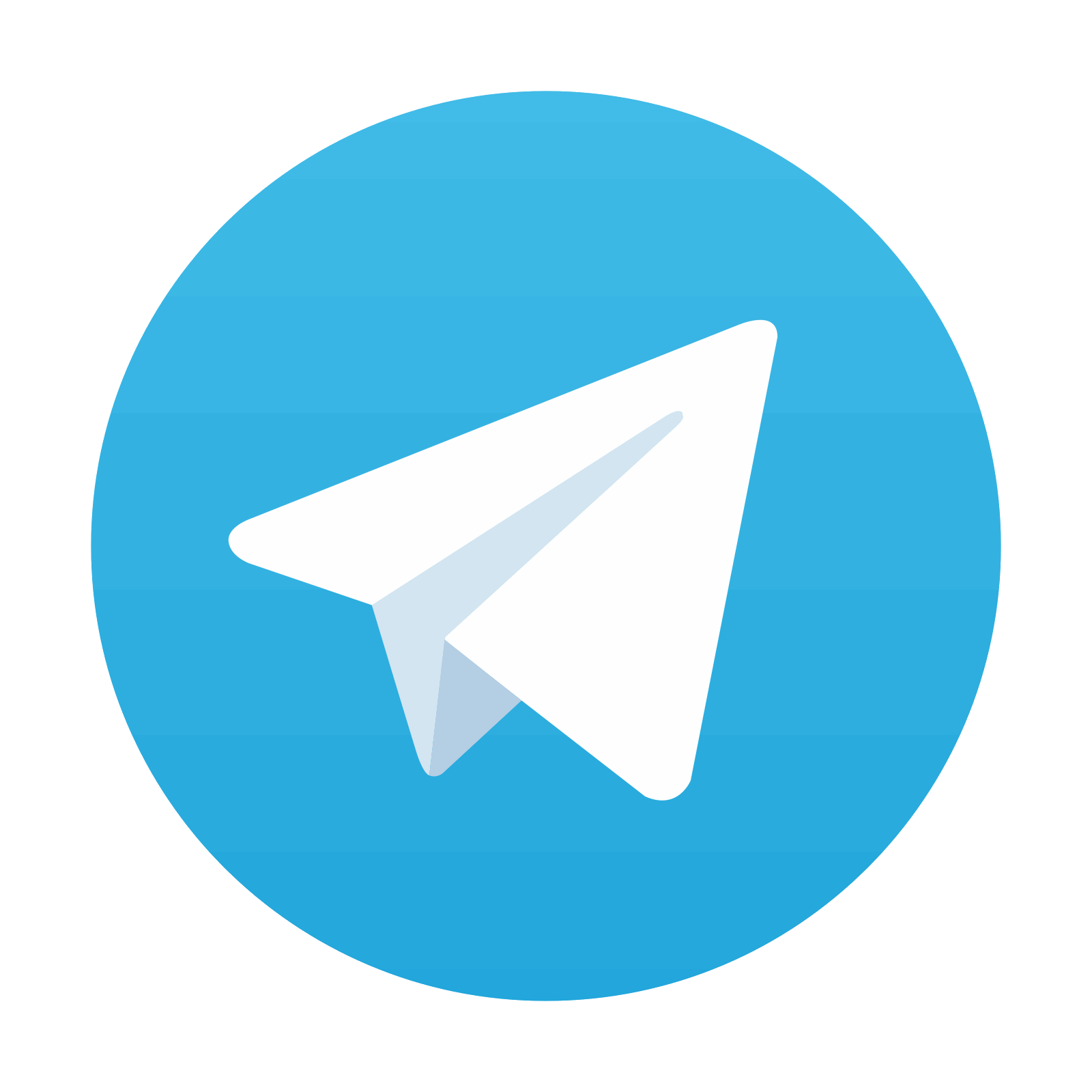
Stay updated, free articles. Join our Telegram channel

Full access? Get Clinical Tree
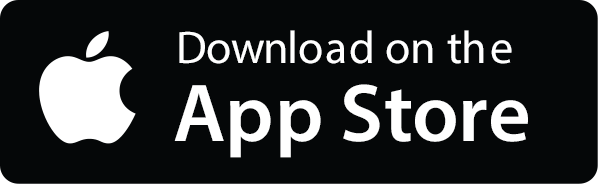
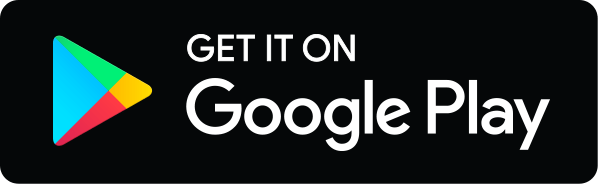