A third generation was also genotyped, as samples from five of the six grandparents were also available. This allowed the generation of a linkage map for ECAX as recombination in the mares could also be assayed. The founding animals represented a number of breeds, namely Arabian, Thoroughbred, Welsh Cob, European Warmblood, and Icelandic Horse, thereby maximizing the chances of heterozygosity. Only 17.1% of markers tested were homozygous across both halves of this pedigree and were therefore uninformative for mapping. Drawbacks to this family were that no phenotypes were available for the embryos, and so could not be mapped, in contrast to the half-sibling families.
The maps resulting from genotyping on this reference family have been published in two stages. The first stage (Swinburne et al., 2000) described the genotyping of all 61 F2 embryos, together with the parental and grandparental individuals, with 353 microsatellites and 6 SNPs. These were placed into 42 linkage groups of which 37 could be anchored to the physical map. The X chromosome and all autosomes except ECA28 had linkage groups assigned to them. The average spacing between the markers was 10.5 cM, and the total map length was 1780 cM.
The subsequent publication (Swinburne et al., 2006) described the genotyping of 734 microsatellites and 8 SNPs. These were assigned to 32 linkage groups, one for each of the 31 autosomes and one for ECAX. Each of these groups was assigned to a chromosome and oriented by virtue of FISH-mapped markers. The total length of this sex-averaged genetic map was 2,772 cM, with the average distance between markers being 3.7 cM. This map length is very close to the predicted length of 2,720 cM estimated from chiasma counts in horses (Scott & Long 1980). Figure 2.2 presents these 32 linkage maps as seen in Swinburne et al. (2006). In addition, the linkage maps are aligned with the contemporary RH map as described in Chowdhary et al. (2003). Alignments were also made to the human genome by in silico mapping, that is, comparing the unique flanking sequence of each microsatellite with the human genome sequence using BLAST; half of those markers tested identified significant and unique matches to the human genome. In a recent study (Mittmann et al., 2010), in which the order of these markers was compared with the horse genome assembly EquCab2.0 (http://www.broadinstitute.org/mammals/horse), only two discrepancies were found: marker order of TYK601 and COR020 was switched, and COR062 was incorrectly positioned on ECA19.
Figure 2.2 The Newmarket map (Swinburne et al., 2006). Sex-averaged genetic linkage maps of the horse autosomes and female-specific map of the X chromosome are shown as grey bars in the center of each figure. The positions of markers along the chromosome are shown in centiMorgans (cM) to the left. Framework markers are shown in bold italics. Markers that could not be ordered with a threshold of LOD > 1 are shown to the right in vertical text. The adjacent vertical line describes their probable location with their most likely position indicated by a short horizontal line. The contemporary RH map is shown to the far right (Chowdhary et al., 2003). Grey bars to the far left indicate proposed segments of conserved synteny between the horse linkage map and the human genome sequence. The identity of the human chromosome is shown adjacent to the bars. The position of match with the human chromosome is shown in megabases. The maps were orientated by reference to FISH mapped markers and genes (Chowdhary et al., 2003).

The International Equine Gene Mapping Workshop (IEGMW) linkage map; the merging of contemporary linkage maps
In an attempt to produce a comprehensive linkage map that would bring together linkage mapping data available at that time, the IEGMW produced a merged map (Penedo et al., 2005). A further 359 microsatellites were genotyped on the IHRFP (Guérin et al., 2003), and this was merged with the Uppsala map (Lindgren et al., 1998) and the Phase I Newmarket map (Swinburne et al., 2000), together with data from a further two half-sibling families. Since the majority of this data originated from half-sibling families, only recombination occurring in the sires was included in the analyses. In total, 766 markers were assigned to linkage groups, with only 59 markers not displaying significant linkage to another marker. The map spans a total of 3,740 cM, with an average of 6.3 cM between markers; it may be that genotyping error – which was estimated at 0.6% – has artificially inflated the map length.
The Selection of Microsatellite Mapping Panels for the Dissection of Inherited Conditions in the Horse
Once substantial linkage maps became available, efforts turned to the selection of well-spaced panels of markers for gene mapping purposes. An important contribution to this choice was the development of horse/human comparative maps to identify the likely positions of genes. Various aspects of comparative mapping are discussed in other chapters, but an important component was the use of in silico mapping to locate horse microsatellites with respect to the human genome (Farber & Medrano 2004; Swinburne et al., 2006; Tozaki et al., 2007). Specifically this involved the sequence comparison of unique microsatellite-flanking sequence to the human genome sequence using BLAST-like alignment tool (BLAT). Some of the comparative mapping performed during this period is illustrated in Figure 2.2.
Microsatellite genome scan panels include multiplex sets of microsatellite markers that are evenly spread over the genome. Sets of markers that amplify robustly under similar PCR conditions are put together for multiplex PCR. Sets of markers that do not PCR-amplify well together are sometimes combined post-PCR before fragment separation. Microsatellite markers in the same set that have a similar fragment size are labeled with different fluorescent dyes. Panels of markers have been developed by a number of research groups; for example, a panel of 316 markers was developed by the Veterinary Genetics Laboratory, University of California, Davis (http://www.vgl.ucdavis.edu/genomic/GenomeScanPanel.pdf). More recently, with the advantage of reference to the genome sequence, Mittmann et al. (2010) identified a highly polymorphic microsatellite scan panel of 322 evenly spaced markers that covers all autosomes and ECAX.
The Continuing Usefulness of the Equine Linkage Map
Despite the recent sequencing of the horse genome and subsequent development of a SNP microarray, the utility of microsatellite panels as a first step in identifying the approximate location of a genetic variant of interest is still apparent. Further, information from the equine linkage map was used to assemble the genome sequence of this species.
For monogenic trait mapping
Genetic linkage maps can be used to identify genes or chromosome regions that regulate various phenotypic traits. Both monogenic traits, controlled by a single gene, and polygenic traits, controlled by an unknown number of genes and often environmental factors, can be studied. As described in previous sections, linkage mapping entails following the segregation of alleles in families to establish whether or not the alleles at one locus co-segregate with alleles at other loci. To be able to do this, it is necessary to determine the parental origin of each allele in the progeny. A trait can be mapped using linkage analysis by genotyping a pedigree in which this trait is segregating using a genome-wide marker panel. Markers that are inherited together (i.e., co-segregating) with the trait are genetically linked to a gene that influences that trait, and thereby indicate its approximate chromosome location. This method, known as linkage mapping, has relatively low resolution and generally results in the identification of large regions (megabases) that require further investigation by fine mapping.
The ultimate aim is to identify the gene responsible for the trait and ideally the causative mutation. To this end the critical region can be reduced in size by haplotype mapping, where more individuals of the same breed – or if possible, other breeds – are genotyped for markers in the region. Ultimately the re-sequencing of affected and control animals would be performed to search the reduced critical interval for candidate mutations. Further confirmatory studies could involve the evaluation of candidate mutations by investigating their biological function within cells.
Linkage mapping has been used to identify chromosome regions and subsequently genes for a number of disorders and phenotypic traits in horses. These include, for instance, the identification or mapping of several coat color genes (Henner et al. 2002; Locke et al., 2002; Swinburne et al., 2002; Terry et al., 2004; Brunberg et al., 2006); mapping of glycogen branching enzyme deficiency (Ward et al., 2003); and mapping of multiple congenital ocular anomalies (MCOA) (Andersson et al., 2008). These studies demonstrate the use of microsatellites for genetic mapping of monogenic traits in the horse. The mapping of monogenic traits is described in more detail in Chapters 11–18.
In assembling the genome sequence
In the same way as linkage groups were assigned to chromosomes and oriented along the chromosome arms, contiguous sequences generated during the horse genome sequencing project were anchored and oriented. This was accomplished by using the existing equine linkage maps (Guerin et al., 2003; Penedo et al., 2005; Swinburne et al., 2006) to place markers with known chromosomal locations onto the equine assembly. This procedure also ensures that the assembly is accurate. The procedure for genome assembly is described in Chapter 7.
References
Allen, W. R., & Brasher, V. (1992). Videoendoscopic evaluation of the mare’s uterus. III. Findings in the pregnant mare. Equine Veterinary Journal, 24, 285–291.
Allen, W. R., & Pashen, R. L. (1984). Production of monozygotic (identical) horse twins by embryo micromanipulation. Journal of Reproductive Fertility, 71, 607–613.
Andersson, L. S., Juras, R., Ramsey, D. T., Eason-Butler, J., Ewart, S., Cothran, G., and Lindgren, G. 2008. Equine multiple congenital ocular anomalies maps to a 4.9 megabase interval on horse chromosome 6. BMC Genetics, 9, 88.
Barendse, W., Armitage, S. M. et al. (1994). A genetic linkage map of the bovine genome. Nature Genetics, 6(3), 227–235.
Beever, J. E., Da, Y., Ron, M., & Lewin, H. A. (1994). A genetic map of nine loci on bovine chromosome 2. Mammalian Genome, 5(9), 542–545.
Breen, M., Lindgren, G., Binns, M. M., Norman, J., Irvin, Z., Bell, K., Sandberg, K., & Ellegren, H. (1997). Genetical and physical assignments of equine microsatellites – first integration of anchored markers in horse genome mapping. Mammalian Genome, 8(4), 267–273.
Brunberg, E., Andersson, L., Cothran, G., Sandberg, K., Mikko, S., & Lindgren, G. (2006). A missense mutation in PMEL17 is associated with the Silver coat color in the horse. BMC Genetics, 7, 46.
Chowdhary, B. P., Raudsepp, T., Kata, S. R. et al. (2003). The first-generation whole-genome radiation hybrid map in the horse identifies conserved segments in human and mouse genomes. Genome Research, 13(4), 742–751. Erratum in: Genome Research (2003) 13(6a), 1258.
Crawford, A. M., Dodds, K. G. et al. (1995). An autosomal genetic linkage map of the sheep genome. Genetics, 140(2), 703–724.
Ellegren, H., Johansson, M., Chowdhary, B. P. et al. (1993). Assignment of 20 microsatellite markers to the porcine linkage map. Genomics, 16(2), 431–439.
Ellegren, H., Johansson, M., Sandberg, K., & Andersson, L. (1992). Cloning of highly polymorphic microsatellites in the horse. Animal Genetics, 23(2), 133–142.
Farber, C. R., & Medrano, J. F. (2004). Identification of putative homology between horse microsatellite flanking sequences and cross-species ESTs, mRNAs and genomic sequences. Animal Genetics, 35(1), 28–33.
Godard, S., Vaiman, D., Oustry, A., Nocart, M., Bertaud, M. et al. (1997). Characterization, genetic and physical mapping analysis of 36 horse plasmid and cosmid-derived microsatellites. Mammalian Genome, 8(10), 745–750.
Guérin, G., Bailey, E., Bernoco, D. et al. (1999). Report of the International Equine Gene Mapping Workshop: Male linkage map. Animal Genetics, 30(5), 341–354.
Guérin, G., Bailey, E., Bernoco, D. et al. (2003). The second generation of the International Equine Gene Mapping Workshop half-sibling linkage map. Animal Genetics, 34(3), 161–168.
Henner, J., Poncet, P. A., Guérin, G., Hagger, C., Stranzinger, G., & Rieder, S. (2002). Genetic mapping of the (G)-locus, responsible for the coat color phenotype “progressive greying with age” in horses (Equus caballus). Mammalian Genome, 13(9), 535–537.
Kosambi, D. D. (1944). The estimation of map distances from recombination values. Annals of Eugenics, 12, 172–175.
Lampe, V., Dierks, C., Komm, K., & Distl, O. (2009). Identification of a new quantitative trait locus on equine chromosome 18 responsible for osteochondrosis in Hanoverian warmblood horses. Journal of Animal Science, 87(11), 3477–3481.
Lander, E. S., & Green, P. (1987). Construction of multilocus genetic linkage maps in humans. Proceedings of the National Academy of Sciences, 84, 2363–2367.
Lindgren, G., Sandberg, K., Persson, H., Marklund, S., Breen, M. et al. (1998). A primary male autosomal linkage map of the horse genome. Genome Research, 8(9), 951–966.
Locke, M. M., Penedo, M. C., Bricker, S. J., Millon, L. V., & Murray, J. (2002). Linkage of the grey coat colour locus to microsatellites on horse chromosome 25. Animal Genetics, 33, 329–337.
Ma, R. Z., Beever, J. E., Da, Y. et al. (1996). A male linkage map of the cattle (Bos taurus) genome. Journal of Heredity, 87(4), 261–271.
Matise, T. C., Perlin, M., & Chakravarti, A. (1994). Automated construction of genetic linkage maps using an expert system (MultiMap): A human genome linkage map. Nature Genetics, 6(4), 384–390.
Mittmann, E. H., Lampe, V., Mömke, S., Zeitz, A., & Distl, O. (2010). Characterization of a minimal microsatellite set for whole genome scans informative in warmblood and coldblood horse breeds. Journal of Heredity, 101(2), 246–250.
Penedo, M. C., Millon, L. V., Bernoco, D. et al. (2005). International Equine Gene Mapping Workshop Report: A comprehensive linkage map constructed with data from new markers and by merging four mapping resources. Cytogenetic and Genome Research, 111(1), 5–15.
Scherzer, J., Fayrer-Hosken, R. A., Ray, L., Hurley, D. J., & Heusner, G. L. (2008). Advancements in large animal embryo transfer and related biotechnologies. Reproduction in Domestic Animals, 43(3), 371–376.
Scott, I. S., & Long, S. E. (1980). An examination of chromosomes in the stallion (Equus caballus) during meiosis. Cytogenetics and Cell Genetics, 26(1), 7–13.
Swinburne, J. E., Bogle, H., Klukowska-Rötzler, J., Drögemüller, M., Leeb, T. et al. (2009). A whole-genome scan for recurrent airway obstruction in Warmblood sport horses indicates two positional candidate regions. Mammalian Genome, 20(8), 504–515.
Swinburne, J. E., Boursnell, M., Hill, G. et al. (2006). Single linkage group per chromosome genetic linkage map for the horse, based on two three-generation, full-sibling, crossbred horse reference families. Genomics, 87(1), 1–29.
Swinburne, J., Gerstenberg, C. et al. (2000). First comprehensive low-density horse linkage map based on two 3-generation, full-sibling, cross-bred horse reference families. Genomics, 66(2), 123–134.
Swinburne, J. E., Hopkins, A., & Binns, M. M. (2002). Assignment of the horse grey coat colour gene to ECA25 using whole genome scanning. Animal Genetics, 33(5), 338–342.
Terry, R. B., Archer, S., Brooks, S., Bernoco, D., & Bailey, E. (2004). Assignment of the appaloosa coat colour gene (LP) to equine chromosome 1. Animal Genetics, 35(2), 134–137.
Tozaki, T., Swinburne, J., Hirota, K., Hasegawa, T., Ishida, N., & Tobe, T. (2007). Improved resolution of the comparative horse-human map: Investigating markers with in silico and linkage mapping approaches. Gene, 392(1–2), 181–186.
Vaiman, D., Schibler, L., Bourgeois, F., Oustry, A., Amigues, Y., & Cribiu, E. P. (1996). A genetic linkage map of the male goat genome. Genetics, 144(1), 279–305.
Wade, C. M., Giulotto, E., Sigurdsson, S. et al. (2009). Genome sequence, comparative analysis, and population genetics of the domestic horse. Science, 326(5954), 865–867.
Ward, T. L., Valberg, S. J., Lear, T. L. et al. (2003). Genetic mapping of GBE1 and its association with glycogen storage disease IV in American Quarter horses. Cytogenetic and Genome Research, 102(1–4), 201–206.
Werner, P., Mellersh, C. S., Raducha, M. G. et al. (1999). Anchoring of canine linkage groups with chromosome-specific markers. Mammalian Genome, 10(8), 814–823.
Zeitz, A., Spötter, A., Blazyczek, I., Diesterbeck, U., Ohnesorge, B. et al. (2009). Whole-genome scan for guttural pouch tympany in Arabian and German warmblood horses. Animal Genetics, 40(6), 917–924.
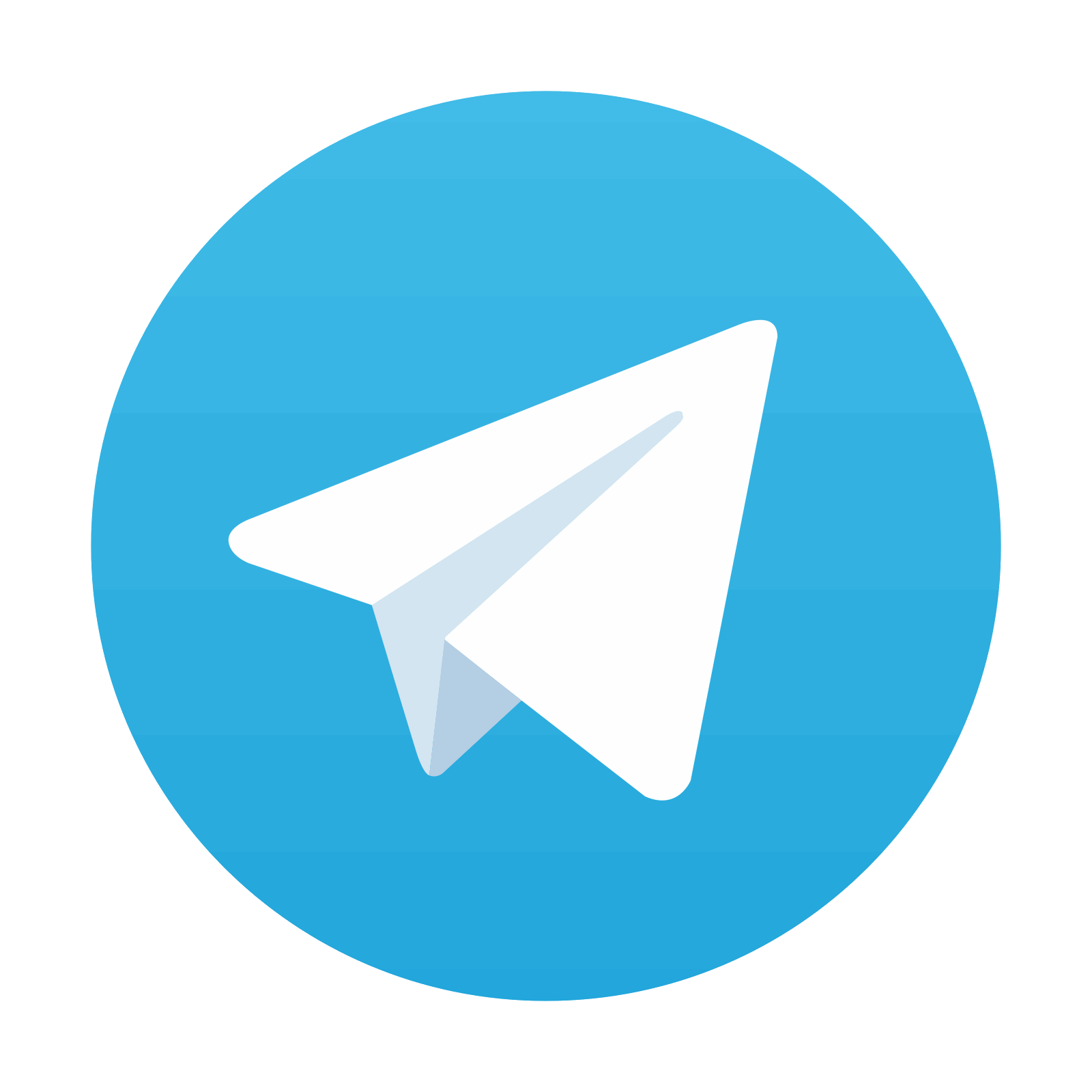
Stay updated, free articles. Join our Telegram channel

Full access? Get Clinical Tree
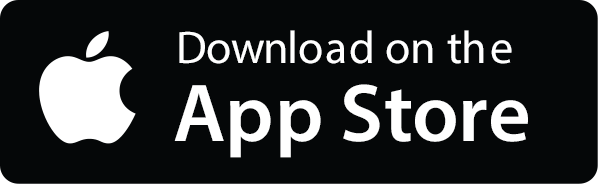
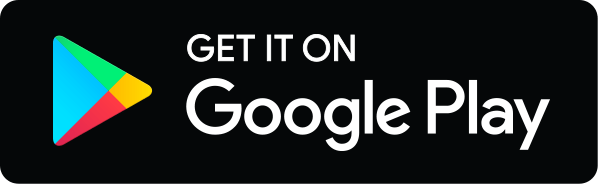