CHAPTER 18 Several interfaces between horse and rider exist. Ridden horses are normally equipped with a bridle and a saddle, which serve as the main transmitters of signals from the rider. Other tools such as spurs and whips are also used to communicate with the horse. Tack often is connected to both horse and rider and, therefore, is suitable to incorporate measuring devices. The most commonly used tools in this respect are strain gauges. The electric resistance of strain gauges changes with the force exerted on them. Strain gauges can be used to assess forces on bits, reins, and stirrups. Furthermore, flat pressure (or “normal”) force sensors incorporated in pads can be placed underneath the saddle, underneath blankets or even simply between the horse and the rider’s legs. Horse–rider combinations with instrumented tack are shown in Figure 18-1. The instrumented tack can be used not only to study the effect of tack itself but also to study the interaction between rider and horse (see below). The use of a saddle, or saddle-like structure, goes back to a long time before the Christian era, but the stirrup is a more recent addition that was invented by the Chinese in the third century ad (Dunlop and Williams, 1996). Although the concept of the saddle has not essentially changed since then, research into the effect of the saddle on the horse is much more recent. Harman (1994) was the first researcher who used a computerized pressure measuring device (SaddleTech) to evaluate the effect of a saddle and saddle pads on the horse.1 This first saddle pressure system was equipped with 256 sensors, which used pressure-sensitive ink printed on a polyester film that changed resistance when subjected to pressure. Harman (1994) studied several saddle pads: cotton-quilted pads, open-cell foam pads, gel pads, and a balancing shim. The most common saddle fitting problem she identified was “bridging.” In “bridging,” the front and the rear panels were contacting the horse, but there was no or reduced pressure in the central area in between. Only 35% of the tested saddle pads improved the saddle fit or at least did not change it in a negative way. The remainder, in fact, increased pressure, which was compared by Harman (1994) to the effect of a sock in a shoe that is too tight. The study of Harman encouraged other researchers to take up the topic. Pullin et al. (1996) used a newer technique, force-sensing array (FSA), for the evaluation of an equine athletic saddle pad and saddle liners.2 They identified several potential sources of error within the system that could affect the objectivity of data collection and interpretation. They stressed the importance of numerical scoring based on specific measurements rather than relying on subjective impressions, the importance of the calibration procedure, the position of the sensor pad, and the position of both rider and horse. They further stated that measuring without a rider is not useful, as the weight of the rider is required to evaluate the weight distribution underneath the saddle. Jeffcott et al. (1999) investigated the validity of the FSA technology. In principle, according to Newtonian laws, the force on the horse’s back should be the sum of the weight of the saddle, the force exerted by a tightened girth, and the weight of a rider. There should, therefore, be a linear relationship between total weight and the pressure measured underneath the saddle. They tested this hypothesis on both a wooden horse and a live horse placed in the standing position. The correlation between weight and measured pressure appeared to be high, indeed. They also presented preliminary data on characteristic changes of the center of pressure at the walk, sitting trot, rising trot, and canter. De Cocq et al. (2006) focused on the validity and repeatability of the FSA technology. They not only confirmed the overall results of Jeffcott et al. (1999) but also demonstrated that there may be considerable drift during a single measurement day, urging frequent (re)calibration. Furthermore, repeatability of the measurement was low when a rider dismounted and remounted a horse. The FSA device was also not able to distinguish satisfactorily between two saddle-fitting procedures, although the filling of the panels (special air-filled panels were used) was significantly different. The newest saddle force measurement technique uses capacitometric sensors (Pliance).3 De Cocq et al. (2009a) tested the Pliance system with respect to validity and repeatability. The reliability of the calibration of the Pliance system decreased during one measurement day but remained within acceptable values. Repeatability of measurements was acceptable as long as the saddle device and the saddle remained on the horse in-between measurements. This stresses the importance of the position of the device on the horse’s back. The Pliance system was able to detect differences when weight was added to the saddle or the rider and between rider positions. The Pliance system has been used in several studies on the effect of saddles and saddle pads. Werner et al. (2002) compared standard and custom-made saddles. They combined the saddle measurements with a clinical examination of horses, which included back palpation. A correlation was found between high pressure values (>3.5 newtons [N]/cm2) and both pain reactions and the occurrence of muscle atrophy affecting the area of the back under the saddle. The following criteria for good saddle fit were identified: a wide, uniform contact area between saddle and horse, maximal pressure values not exceeding 3.0 N/cm2, and no pressure peaks or “bridging.” Meschan et al. (2007) studied the effect of the width of the tree on the forces and force distribution underneath the wooden tree saddle. They demonstrated that the load under poorly fitting saddles was distributed over a smaller area than under properly fitting saddles, with the potential to lead to harmful pressure peaks. Mönkemöller et al. (2005) demonstrated that an adjustment of saddle fit can enlarge the contact surface underneath the saddle and hence, by reducing pressure in the region of the caudal thoracic spine, reduce the number of horses demonstrating back pain. Nyikos et al. (2005) subdivided the contact area of the saddle into six regions. They found that the lumbar area was more sensitive to pressure than the area of the withers. They concurred with Harman (1994) that “bridging” was the worst problem related to saddle fit. Harman (1994) had stated that saddle pads under poorly fitting saddles may often be detrimental, and Kotschwar et al. (2010) investigated the use of a pad under an overly wide saddle, theorizing a beneficial effect. Although significant intrahorse effects were demonstrated, there was no significant interhorse effect. The choice of a saddle pad to improve the fit of an excessively wide saddle (if such a saddle is to be used anyway) should, therefore, be based on highly individual criteria for each horse. Latif and colleagues (2010) used the Pliance system to measure saddle pressures generated underneath three different racing saddle types: wooden tree, treeless, and flexible tree. With a rider taking the jockey seat, canter and gallop exercise peak pressures occurred underneath the front of the saddle, with these shifting caudally during the trot exercise. They showed that horses were at greatest risk of high peak forces during trotting exercise, especially in the mid-saddle region in a treeless saddle, and in the hind region of the wooden tree saddle. The resultant effect of high saddle pressures is saddle soreness, so owners should be encouraged to check their horse for dry spots underneath the saddle which may be an indicator of high saddle pressures and resultant soft tissue damage (von Peinen et al., 2010). Research on the effect of the bridle has, thus far, been focused on the bit. The bit is probably the oldest piece of tack that has been introduced in horse riding. Evidence of the use of bits has been found in two different archeological sites of the Botai, a culture located in the Aqmola province of Kazakhstan, famous for its very early use of the horse dating from about 3500 to 3000 bc (Anthony and Brown, 2000). The bit is in direct contact with the horse via the mouth, and aids given by the hands of the rider are transmitted through the reins and the bit directly to the horse’s mouth. As a large part of the bit is hidden from view, fluoroscopic techniques have been used to evaluate the position and action of several bits (Clayton, 1985; Clayton and Lee, 1984). The first bit that was studied was a joined snaffle bit. In the resting position, the mouthpiece was interposed between the tongue and the hard palate, indenting the dorsum of the tongue. When applying an equal force to both reins simultaneously, the bit was moved caudally, deepening the indentation in the horse’s tongue. When applying asymmetrical force, the net effect depended on the relative forces applied to the active and opposing rein. It was not possible to produce an independent effect on one side of the mouth. The jointed mouthpiece was suspended in a more horizontal position when keepers were used to fix the position of the bit rings relative to the cheek pieces of the bit. The keepers also reduced the mobility of the bit within the oral cavity. Less intraoral mobility was also observed in bits with a single mouthpiece. A bit that had two joints connected to an angled plate could be positioned in ways that the plate lay either parallel or perpendicular to tongue and palate, which makes a marked difference with respect to the severity of the impact of the bit. The fluoroscopic studies demonstrated that the force exerted on the bit greatly influences the position and movement of the bit in the mouth of a horse. A next logical step was to measure the force that riders apply to the reins and which is transmitted to the bit. In several studies, force sensors have been attached in between the bit and reins to measure rein forces (Clayton et al. 2005; Preuschoft et al., 1999; Warren-Smith et al., 2005). Preuschoft et al. (1999) analyzed the functions of a number of bridles (“head gears”) biomechanically. Most bridles are designed to transmit tensile forces applied through the reins or a lunge to the sensitive parts of the horse’s mouth. The direction, duration, and magnitude of these forces are essential factors in controlling the horse. Several bridles and two major types of bits (with or without levers) were analyzed using a device that could roughly quantify these forces. Rein forces were found to show regular patterns and to be dependent on the horse’s gait. During competition, forces between 20 and 147 N were measured; in recreational use the range was 20 to 49 N. Clayton et al. (2005) used a more precise load cell and found similar rein force patterns consisting of a series of spikes with frequencies corresponding to two per stride in the walk and trot and one in the canter. They established a maximum force of 104 N in the canter. Warren-Smith et al. (2005) focused on developing a low-cost and practical sensor and recording system that could be used in everyday training. They tested two sensors on horses that were being led, lunged, and ridden and measured forces in the range of 0 to 30 N for light rein contact. As other studies had reported considerably higher forces, they claimed that horses might be subject to unnecessarily high forces and that the education of horses and riders could be improved. In a follow-up study, the same authors (Warren-Smith et al. 2007) focused on specific equitation exercises: left turn, right turn, going straight and halt, in both long-reining and riding. The rein force required for going straight was lower, and the force required to elicit the halt response was greater than for any other activity. The mean force exerted during long-reining was 10.7 ± 1 N and for riding was 7.4 ± 0.7 N. These, again, were lower values than those recorded by others in earlier studies. Heleski et al. (2009) used a combination of behavioral observations and rein force measurements to study the effect of martingales and rein inserts. There is controversy about the use of these tools. Some claim that they can reduce discomfort caused by inexperienced and unsteady hands. Others consider them inappropriate “crutches.” No differences in conflict behavior were observed in horses with or without martingales or rein inserts. Mean rein forces were higher for martingales than for controls or rein inserts. The head of the horse was lower for horses ridden with martingales. It can be concluded that carefully fitted martingales might have a place in riding schools that teach novice riders.
Functional biomechanics: Effect of the rider and tack
Effect of tack on the horse
Effect of saddle and saddle pads
Effect of reins and bridle
Stay updated, free articles. Join our Telegram channel

Full access? Get Clinical Tree
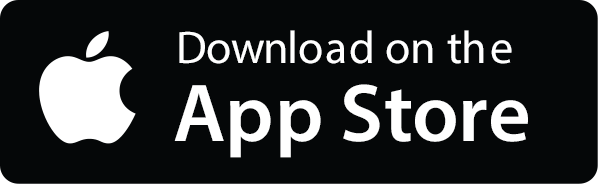
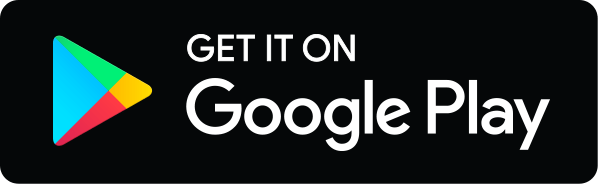