Fig. 1.
Photographs of hand made and commercially available filaments. From top to bottom, a filament made from fishing line, which has been blunted at the tip, a commercial filament from Doccol Company, USA, and a Xantopren coated Prolene filament.
1.
Wearing gloves, cut 3.0 surgical monofilament (Resorba, Germany), preferably nylon but Prolene or Mopylen have also been used, into 30-mm lengths.
2.
Blunt one end with heat. The material determines how smooth the blunt end will be.
3.
Mix Xantopren L silicon and Universal Activator (Heraeus Kulzer, Germany) and quickly dip the blunted end of the filament into the mixture before it hardens.
4.
Measure the diameters of the silicon-coated tips under the microscope and select those with a range of 380–410 μm. Discard filaments that appear sharp.
5.
Bend or mark the uncoated end approximately 20 mm away from the coated tip.
6.
Store in a clean environment to avoid dust.
7.
Ideally, filaments should be used only once. Alternatively, clean with 70% alcohol if re-use will be common.
2.2 Surgical Procedures
2.2.1 Animal Preparation and Care
1.
Ensure the surgical area and instruments are clean, preferably sterilized, between animals.
2.
Anaesthetize the animals with inhalable isoflurane in a 70:30 nitrous oxide:oxygen mixture.
3.
Core body temperature must be maintained at 36.7 ± 1°C throughout the duration of the procedure. The preferred method is automated heat blankets (Harvard, UK).
4.
Shave and clean the incision sites with 70% alcohol or iodine scrub.
5.
Provide adequate post-operative care. Animals that exhibit dehydration can be re-hydrated with daily subcutaneous injections of 2.5 mL of physiological saline and 5% glucose (Animal Care Limited, UK). Moistened diet should also be provided until weight stabilizes in a dish that is either secured to the cage or too heavy to allow disruption.
6.
Assistance with pain relief can be provided according to the guidelines of your local veterinarian. We routinely mix 1 mg/mL of Paracetamol (Boots, UK) into the drinking water for 1 day before and up to 3 days after the surgery.
2.2.2 Laser Doppler Flowmetry
For an indirect measure of cerebral blood flow in the MCAO territory to confirm correct filament placement, Laser Doppler Flowmetry (LDF) can be used. Several different systems and probes exist (Moor Instruments, UK; PeriMed, Germany) but we will describe the method we find the most useful.
1.
Place the animals on their side (the same side that the MCAO will take place). Shave and clean the area between the eye and ear taking care not to destroy the vibrissae. Make a small vertical incision (from top to bottom of head) using blunt dissection.
2.
Place a calibrated 1-mm Laser probe against the skull through a small incision in the temporal muscle. Carefully rotate the rat into a supine position and secure the probe so that a stable baseline value can be observed.
3.
It is advisable to record the measurements.
2.2.3 Middle Cerebral Artery Occlusion
The description that follows is to perform the MCAO on the right side of the animal, though there is no reason why it can not also be performed on the left side.
1.
Make a 1- to 2-cm vertical incision along the midline in the prepared neck using blunt dissection.
2.
Gently retract the right mandibular glands, pretrachial strap and sternomastoid muscles to expose the carotid artery (CA). The surgeon should be familiar with the anatomy of the CA (Fig. 2).
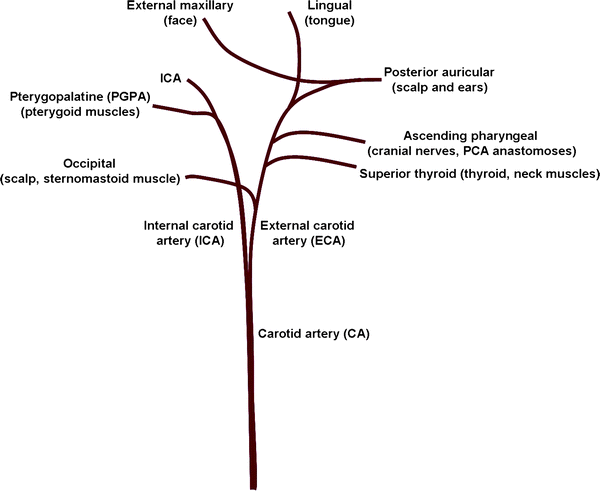
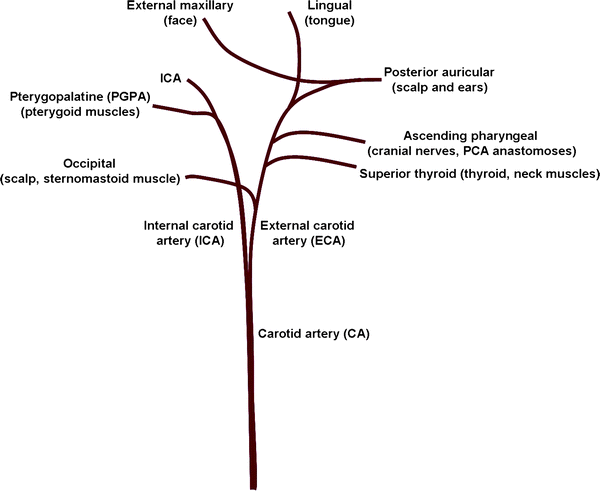
Fig. 2.
The vasculature supplying the rodent head. The CA branches into the ECA and ICA and both give off extra-cranial projections: pterygopalatine, occipital, superior thyroid, ascending pharyngeal, posterior auricular, external maxillary and lingual arteries.
3.
Gently dissect and retract the vagus nerve away from the CA.
4.
Tie a silk suture around the CA as near to the chest as you can get.
5.
Gently detach connective tissue from the CA using opening and closing movements of blunt forceps until the bifurcation of the external and internal carotid arteries (ECA and ICA, respectively) is visible.
6.
Tie a silk suture on the ECA and another loose suture on the ICA taking care not to disrupt the small branches (Fig. 2).
7.
Place a microclip above the loose suture on the ICA.
8.
Once a closed system is ensured, use Vannas scissors to make a small hole in the CA below the bifurcation.
9.
Insert the blunted end of the filament into the incision and advance it to the microclip.
10.
Tighten the loose suture around the filament.
11.
Release the microclip and advance the filament up the ICA. Take care to make sure the filament does not enter the pterygopalatine artery. Retract the ECA away (towards the left) to facilitate this.
12.
The distance from the ECA and ICA bifurcation to the MCA is 17–22 mm in rats. Once the mark or bend of the filament nears the CA incision slow the advancement and watch the Laser Doppler carefully for decreases. Many labs use the Laser Doppler decrease as criteria for inclusion or exclusion. Generally, a stable decrease of approximately 80% of baseline predicts a large cortical and subcortical stroke (Fig. 3).
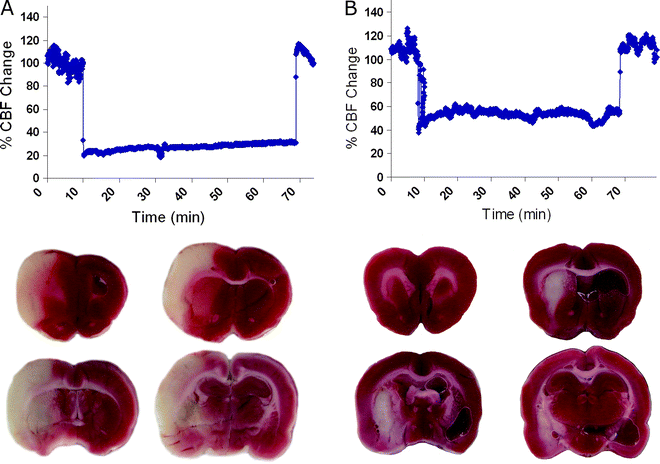
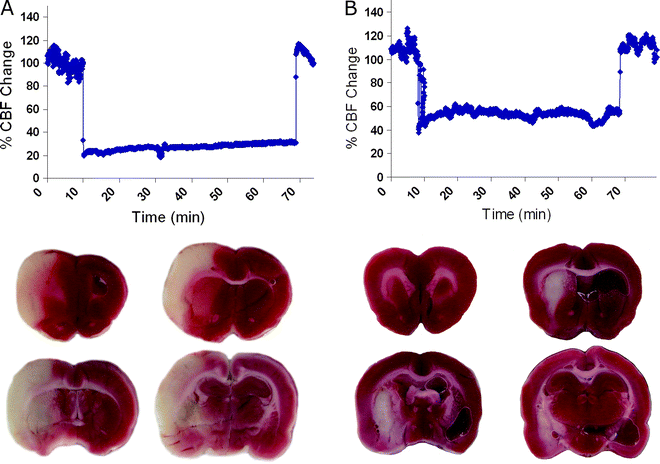
Fig. 3.
Using Laser Doppler flowmetry to predict pathology. Laser Doppler recordings in the middle cerebral artery territory of an animal that exhibited a large cortical and subcortical stroke (a) and an animal with an insult confined to the striatum (b). The ischemic region is depicted in white on the 2,3,5-triphenyltetra-zolium chloride (TTC)-stained sections.
13.
Once a decrease is observed, note the time.
14.
During the desired occlusion time, typically 30, 60, 90, or 200 min, the animals can be left in place (for LDF) or recovered. It is also possible to produce a permanent occlusion by leaving the filament in place and recovering the animal. Generally, 30 min of MCAO produces striatal insults whereas longer occlusion times (60 min or more) produce larger strokes (typically cortical and subcortical). However, this must be tested and established in each laboratory with the desired animal strains and filaments.
15.
Retract the filament and replace the microclip to allow release of the suture for filament withdrawal. Then tighten the suture again and remove the microclip.
16.
Remove the ECA suture to re-establish perfusion in the extracranial territories.
17.
Carefully replace the muscles and glands and suture the wounds.
2.3 Behavioural Tests
The most common symptom of human MCAO is contralateral hemiplegia and hemianesthesia, which is a combined sensorimotor deficit in the injured (contralateral) side of the body, face and extremities. As such, traditionally, the most common behavioural tests for rodents with stroke were designed to examine the difference in function between the intact (ipsilateral) and impaired (contralateral) side of the body (asymmetry tests). These tests include the bilateral asymmetry (adhesive removal test) (8, 9), spontaneous forelimb use asymmetry (cylinder test) (10, 11), paw reaching (staircase test) (12), stepping/paw placing tests (13, 14), drug-induced rotation (15) and the disengage test (16). Many of these tests were originally developed for other unilateral models of basal ganglia disorders, such as Parkinson’s and Huntington’s disease. However, these tests also reveal deficits in animals with ischemia confined to subcortical areas, predominantly the striatum (17).
The majority of these tests examine combined sensorimotor function; however, clever manipulations can be used to distinguish the sensory component from the motor aspect (see Note 7). It should be noted that animals with purely subcortical lesions following MCAO are prone to behavioural recovery. This is particularly evident if rigorous selection criteria are followed, such as excluding animals with any sign of haemorrhage on magnetic resonance imaging (MRI), and using a rigorous surgical method (see Note 1) to minimize the secondary effects of surgery. In our hands, tests of skilled motor function (paw reaching) and apomorphine-induced rotations are the most robust and least likely to recover (see Note 4).
2.3.1 General Considerations Prior to Behavioural Testing
Study Design
One of the first considerations when designing a behavioural experiment involving MCAO rats should be appropriate control groups. When using the intraluminal filament model it is necessary to have a sham surgery group. These sham animals should undergo the same surgical preparation in the neck as the lesioned animals. This is necessary as the surgical procedure itself will have an effect on the behavioural responses of the animals, particularly if the rats are tested within the first week following surgery.
The choice of behavioural tests should also be carefully considered, and a number of questions need to be addressed when making this decision. What is the purpose of the experiment? If you are assessing a potential therapy, what is the mechanism of the treatment? For example, unless you are examining a treatment that is potentially going to protect/replace a significant number of medium spiny neurons within the striatum, you are unlikely to see a recovery in the number of apomorphine-induced rotations. Will there be sufficient time prior to surgery to train rats? Is food restriction a feasible option for tests such as paw reaching? What time points post-surgery are you testing? Testing in the first few days following surgery can result in spurious results because animals have not sufficiently recovered and can be lethargic. It is also important to consider how many times rats are going to be exposed to each test. Tasks such as the cylinder and adhesive removal can become ineffective if the rats are exposed to them too many times. Animals with lesions may also develop compensatory mechanisms on some tasks and/or recover function regardless of treatment. Rats should be tested long enough to see if there is recovery in non-treated animals. Many different factors govern whether functional recovery is evident on behavioural tests and learned compensation can contribute to recovery (for a review see (18)). For example, if you see improvement with a potential therapeutic, you must ask whether or not the therapeutic strategy truly caused “brain repair”; or merely sped up the compensation process via secondary mechanisms. One obvious secondary mechanism could be that the treatment merely increased the motivation of the animal, i.e. an injection of substance x could reduce lethargy by increasing hydration. Stringent controls and longitudinal testing with appropriate tasks should overcome such issues. Once the overall design of the experiment has been carefully planned it is then important to consider the smaller points that can have a large affect on behaviour.
Housing, Handling and Test Environment
For behavioural testing, it is important that animals are well handled and unstressed. Rats are social animals and should ideally be housed in groups. We have found with the less severe subcortical lesions it is not necessary to house lesion and sham animals separately, provided moist diet is given regularly following surgery. However, occasionally one animal may need extra care and single housing following surgery. If possible, the rat should be reintroduced to the group once it has recovered sufficiently. However, this should be done with caution as adult male rats can become aggressive; although we have not found this to be an issue with male Wistar rats. If it is necessary to house sham and lesioned animals separately, ensure that the order of testing is arranged so that both groups are mixed, to prevent confounds in the data.
The time of day rats are tested may affect performance and therefore it is also important to keep this constant. Rats should be habituated to both the test rooms and equipment before testing (except for the cylinder test). This will calm them and reduce the levels of anxiety. For the same reason, the test environment should be kept as uniform as possible, with unexpected noises and smells being avoided. A quiet radio in the background of the testing rooms will reduce startling of the animals when an unexpected noise happens. However, a radio should only be used when it will not affect the parameters of the task. For example, it is unwise to use a radio during spatial learning tasks, as the noise will act as an unintentional cue to the animals.
Equally important as maintaining a constant test environment is having the same well-trained experimenter handling the animals. The rats may behave differently if being handled in an unfamiliar way, and small differences in technique on tests such as the lateralized stepping task can produce a large difference in the data obtained. It is also important that testing is carried out by somebody who is blinded to the condition of the animals, as some of the tests are easily unintentionally influenced.
Food Restriction
Food restriction is required for a number of tests and there are a number of protocols that can be used. The method we use aims to maintain rats at 85–90% of their free feeding weight (the weight they are prior to food restriction). To achieve the desired weight range, the rats are given a set amount of food each day and weighed three times a week to ensure the quantity of food is correct. The level of food can be adjusted depending on the level of performance of the rats, provided that the weight remains above 85% of their free feeding weight. If the rats are not yet fully grown, the free feeding weight must be adjusted on a weekly basis in line with a growth curve, which can be obtained from the supplier. We do not food restrict animals until they have fully recovered from surgery and have regained lost weight. This therefore limits how close to surgery tests that require food restriction can be performed.
2.3.2 Apomorphine-Induced Rotations
Apomorphine is an agonist to post-synaptic dopamine receptors, which are expressed on the medium spiny neurons of the striatum. In essence, when a rat with a unilateral lesion to the striatum is administered apomorphine, the drug will stimulate the intact striatum more that the lesioned striatum. This results in the rat circling ipsilateral to the side of injury, an action which can be quantified. However, the location of the striatal injury will influence the degree and direction of rotations (19). It should be noted that the mode of action of apomorphine when administered to rats with striatal MCAO lesions is similar to that of animals with excitotoxic lesions of the striatum, but different from that in rats with unilateral 6-OHDA lesions. In the latter instance, very low doses of apomorphine are administered to activate only the super-sensitive dopamine receptors of the dopamine-depleted striatum. This results in the rats circling contralateral to the side of injury (see Dunnett and Torres, volume 1, chapter 15). Within our laboratory we find that apomorphine-induced rotations provide a robust measure of striatal damage following MCAO. We describe here what we have found to be the best protocol to perform apomorphine-induced rotation in MCAO rats.
1.
Inject the rat subcutaneously with 1 mg/kg of R-(−)-Apomorphine hydrochloride hemihydrate (Sigma-Aldrich, UK), 0.2% ascorbate in physiological saline (see Note 5).
2.
Place the rat into the bowl of an automated rotometer (Med Associates Inc, USA), with an elastic band or harness secured around the ribcage of the rat (Fig. 4). The band is connected to a wire, which is in turn attached via a pivot to the rotometer head. This head transforms the turns of the rat into electrical pulses that are interpreted by a computer interface. The rotometer bowl is located in a cylinder with removable lid to prevent rats climbing out.
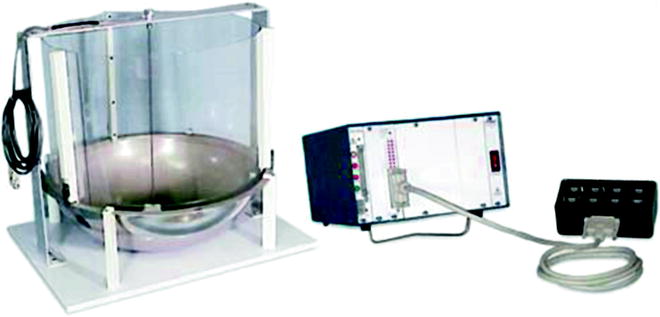
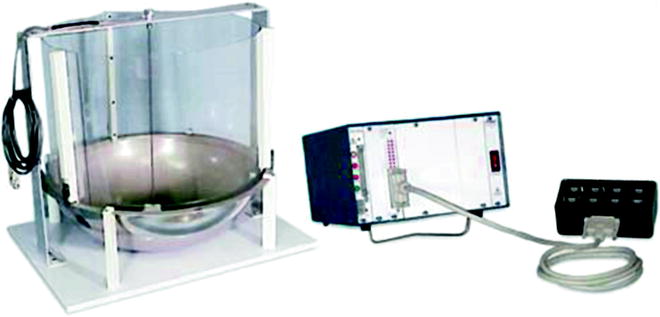
Fig. 4.
Rotometer. The model shown is from Med Associates, USA.
3.
Start program, and repeat above steps for each rat.
4.
Record rotations for 60 min.
5.
The software (MedPC Rotorat software, Med Associates Inc, USA) records the total number of rotations in both directions.
6.
Net total number of rotations is determined (# clockwise – # counterclockwise turns). This can be expressed as rotations per minute or net total rotations.
7.
Many companies sell automated rotometers, but the basic principles of all these setups are the same. If the equipment is not available, it is possible to score rotational behaviour manually. The injected rat is placed into a bowl either observed or filmed so that whole rotations clockwise and counterclockwise can be counted.
2.3.3 Lateralized Stepping and Vibrissae Evoked Paw Placing
Lateralized stepping is a method for assessing forelimb akinesia in the rat and was originally developed to evaluate unilateral lesion models of Parkinson’s disease (13, 14). The rat is restrained and moved in a fashion that should evoke adjusting movements of the free forelimb. The absence of these movements indicates deficits in initiation of motor responses of the forelimb. For each forelimb, the rat can be moved in two directions, towards the midline (forehand) or away from the midline (backhand). We have found that the forehand measure is more robust for assessing functional deficits in the MCAO model. This may be due to backhand measure invoking a vestibular response, due to a slight twisting in the rat’s trunk caused by the body being moved across the forelimb.
Vibrissae-evoked placing requires the rat to be restrained in a similar way and examines the initiation of forelimb movement from the stimulation of the whiskers unilaterally. We have not found the vibrissae-evoked placing to be sensitive to the 30-min MCAO model, due to recovery. However, with larger lesions this test is effective. For issues with lateralized stepping and vibrissae invoked paw placing, see Note 7. Both tests are performed by restraining the rat in a similar manner and manipulating them.
1.
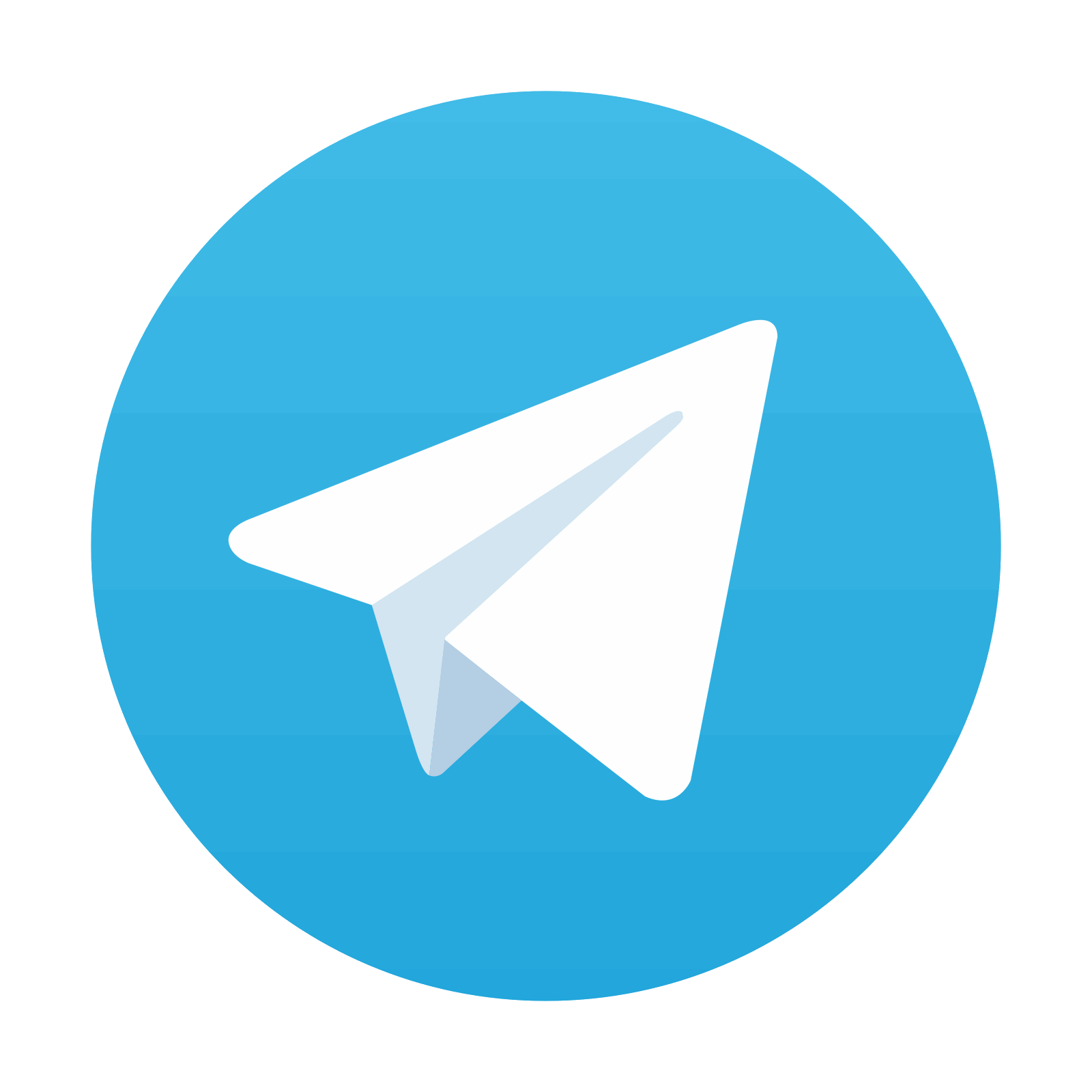
Handle the rats prior to testing and introduce the restraining technique so that they are habituated to the procedure and are calm during data collection.
< div class='tao-gold-member'>
Only gold members can continue reading. Log In or Register a > to continue
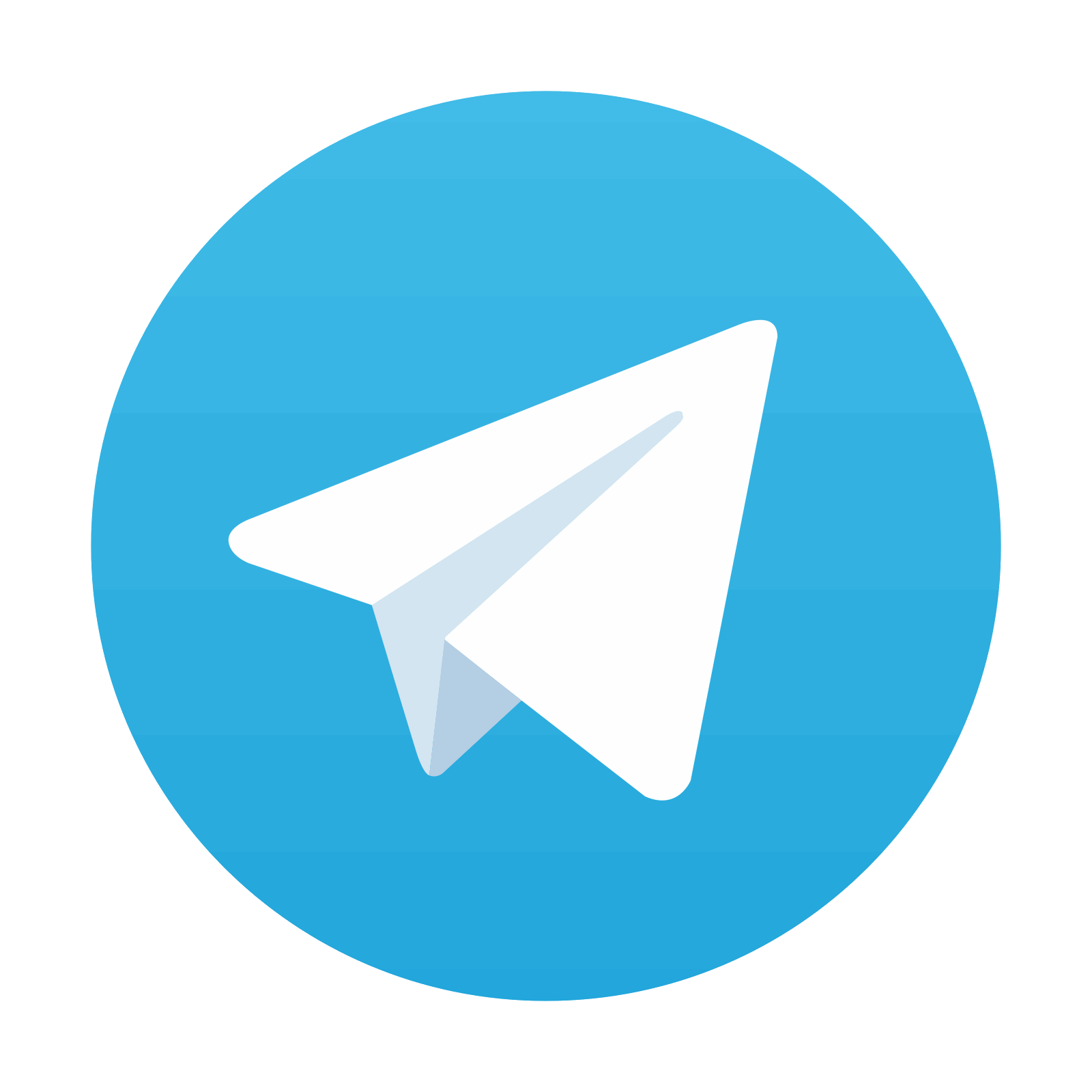
Stay updated, free articles. Join our Telegram channel

Full access? Get Clinical Tree
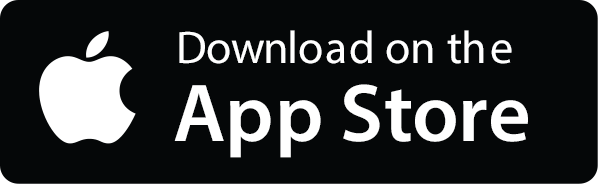
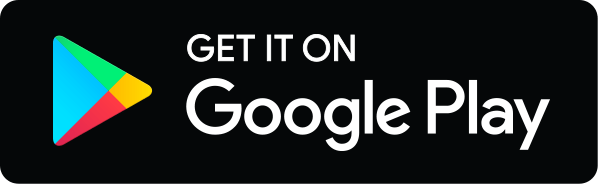
