, Patricia A. Noguera1 and Trygve T. Poppe2
(1)
Marine Scotland Science, Aberdeen, UK
(2)
Norwegian School of Veterinary Science, Oslo, Norway
Abstract
The microscopic examination of stained sections and the ability to interpret the relationship between fine structure and function is essential. The recognition and interpretation of physiological and pathological processes requires a thorough understanding of normal tissue structure and microanatomy, and importantly the variations within species are crucial for correct interpretation. This chapter covers the physiological changes, sexual maturation and aging processes that are ‘normal’, and inherently different from those resulting from injury, infection or disease.
Keywords
HistologyAnatomyNormal structureSalmonTroutMicroanatomy or histology, the science of tissues, is the microscopic examination of thin, stained sections that allows the interpretation of the relationship between fine structure and function. Individual cells and tissues may undergo changes during physiological responses, sexual maturation and aging, that are ‘normal’ and largely different from those resulting from injury, infection or disease processes. Therefore, knowledge of the normal structure and variations within species is of crucial significance for correct interpretation and understanding of pathological changes.
Within the body, the coelomic cavities (pericardial and abdominal), various organs and components, are surrounded or held in position by layers of a serous membrane, the peritoneum, a mesothelium and connective tissue containing blood and secondary (lymph) circulation which also lines the septum transversum that separates both cavities. In this way the outermost layer covering the different regions of the alimentary canal known as the ‘serosa’, is effectively the ‘visceral peritoneum’, and covers other viscera lying within the ‘peritoneal cavity or space’, hence they are not in the body cavity but enveloped within a double layer of peritoneum, and analogous to pushing a finger within a balloon. This also determines that some organs are ‘retroperitoneal’, for example, the kidney.
Throughout this chapter we will refer to the functional unit of an organ as the parenchyma, while the space in between these parts, cells or functional units, is referred as the interstitium. The latter may contain various cellular and extracellular elements, supporting structures or secretions. For example, in the kidney the nephrons and the blood vascular system are embedded in the meshwork of the interstitium, with non-renal elements such as haematopoietic, secretory and supportive cells; whereas the liver consists of hepatocytes, with a meshwork of connective tissue, namely the framework of the organ.
In this chapter an overview of the anatomy of salmonids is presented by ‘systems’ (e.g. respiratory system, excretory system) and organised by a functional approach.
The staining of light micrographs are only identified when haematoxylin and eosin (H&E) is not used, and the magnification for the images are given either as a scale bar or if without a scalebar are identified as low power ×4–10; medium power ×20–40; high power ×60–100 (Fig. 2.1).
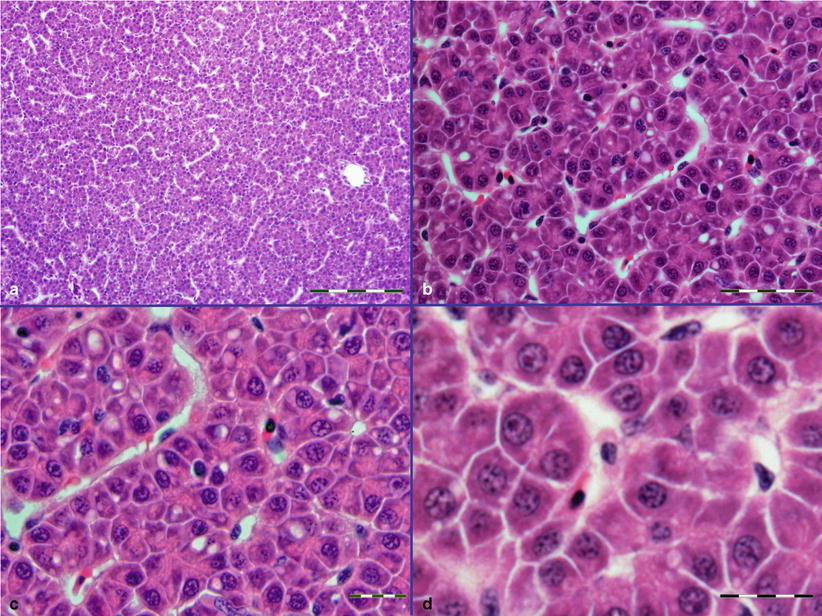
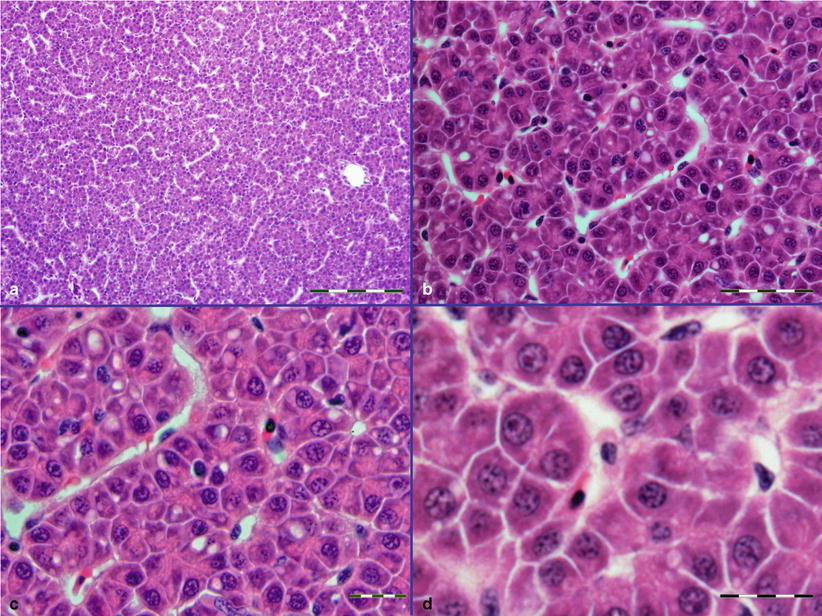
Fig. 2.1
Sections to illustrate different magnifications with representative bar scales. (a) Bar scale = 100 μm, ×20. (b) Bar scale = 50 μm, ×40. (c) Bar scale = 20 μm, ×60. (d) Bar scale = 20 μm, ×100
2.1 Respiratory System (including the operculum and pseudobranch)
Salmonids have four gill arches bilaterally placed on each side of the head, each supporting a holobranch with its two hemibranchs, the double vertical rows of gill filaments (Fig. 2.2). A series of cartilaginous or bony projections, the gill rakers, protrude forwards from the pharyngeal margin of the gill arch. These are relatively sparse in most salmonids but show a wide variation of morphologies in different species, and may form a fine grid that helps filter planktonic organisms from the water, at the same time preventing food particles from entering the gill chamber. Each hemibranch comprises a row of posterior-laterally oriented filaments with its respiratory epithelium covered lamella on each side (Fig. 2.3). Anatomically the filaments looks like a ‘feather’, supporting on each side a continuous symmetrically-spaced individual lamella. The filaments are supported along their proximal half by an interbranchial septum of connective and muscle tissues, but the septum is reduced to about a third of the filament length or even absent in more advanced fish. Each lamella consists of a supportive scaffold of pillar cells among which blood supply enters and leaves the lamella, which are covered by a thin double-layer epithelium separated by a space in which migrating inflammatory cells may be seen. The inner layer of the epithelium sits on a basement membrane that traverses the opposing face of the lamella in grooves located within the pillar cells, and in this way provides additional tensile support. However, the bulk of the respiratory epithelium obvious through light microscopy, is the outer squamous layer that provides a large and intimate interface with the water for exchange of gases, acid–base regulation, osmoregulation and excretion of nitrogenous waste products. Chloride and mucous cells, normally found near the base of the lamellae, may also be found distally under pathological conditions, especially the mucous cells (Fig. 2.4). Chloride cells are highly rich in mitochondria and responsible for the secretion of sodium chloride from the blood, they are prominent in fish living in the marine environment and characteristic during smoltification, but their number may also increase in several pathological conditions. Other cells within the filament interstitium include lymphocytes, eosinophilic granular cells (EGCs) (see Fig. 4.15), macrophages, neuroepithelial cells and rodlet cells. Granular and neuroepithelial cells are obvious at the base of the lamella and more frequent in marine than in freshwater fish. Intraepithelial lymphocytic cell accumulations can be seen at the caudal edge of interbranchial septum in Atlantic salmon, and shown to be a lymphoid tissue and probably of significance for surveillance of gill infections (Fig. 2.5).
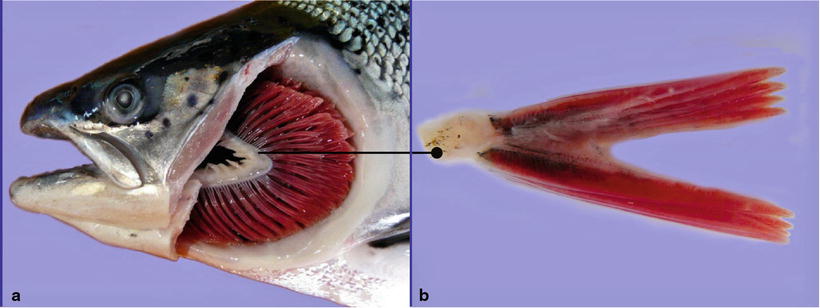
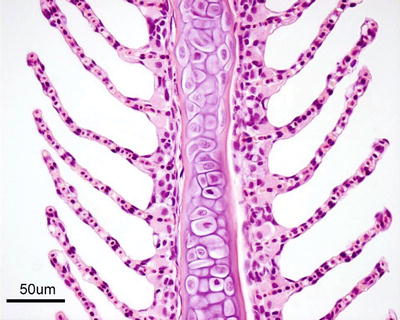
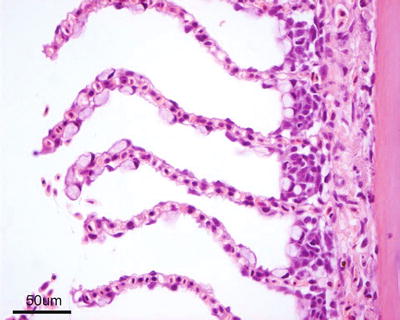
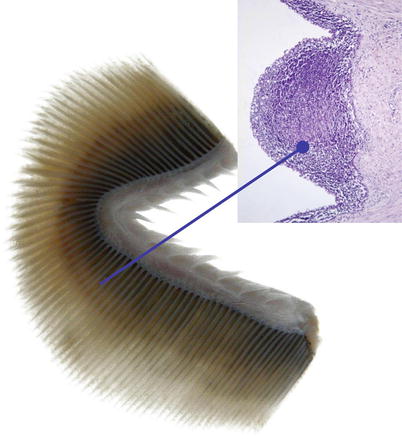
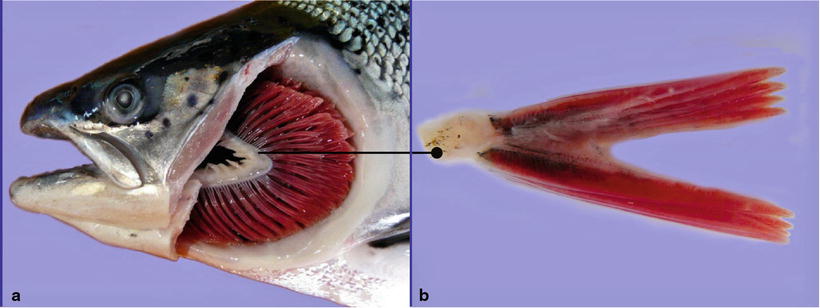
Fig. 2.2
(a) Gills of an adult wild Atlantic salmon, left operculum is removed. The complete gill arch with its gill rakers can be seen. (b) Transversal section of one holobranch showing the hemibranchs with the vertical rows of gill filaments
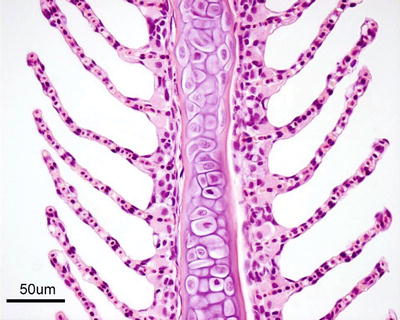
Fig. 2.3
Gill filament, central cartilage and lamellae from adult Atlantic salmon. Chloride cells are located near the base of the lamellae
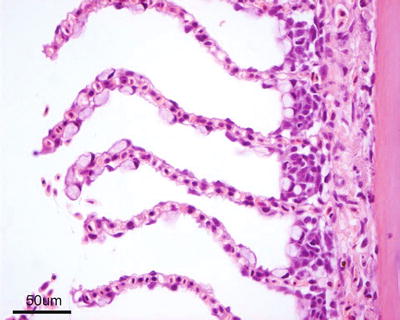
Fig. 2.4
Goblet (mucous) cells on lamellae of farmed adult Atlantic salmon
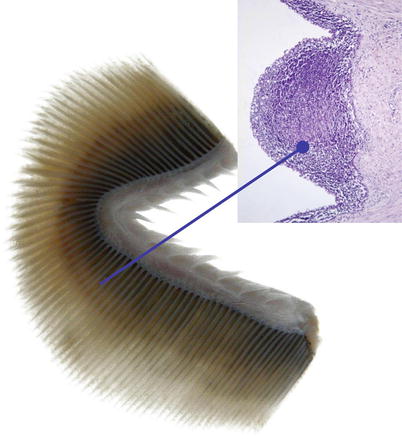
Fig. 2.5
Gills and location of interbranchial lymphoid tissue from Atlantic salmon. Insert medium power
Blood flow in the lamella capillaries is opposite to the ventilatory water flow (‘counter current’) ensuring effective gas exchange between blood and water across the respiratory epithelium. Venous blood from the ventral aorta diverges to the afferent arterioles and capillaries of the lamellae where gas exchange takes place and becomes arterial blood. Arterial blood is drained through the efferent branchial artery and into the dorsal aorta. The water flow over the lamellae is continuous and achieved by means of the buccal-opercular pump.
Dorsally, on the inner surface of each operculum is the pseudobranch, a rudimentary first gill arch (Figs. 2.6 and 2.7). The pseudobranchial cells are in close proximity to a network of blood vessels and may play a role in the blood supply to the retina and in osmoregulation and sensing.
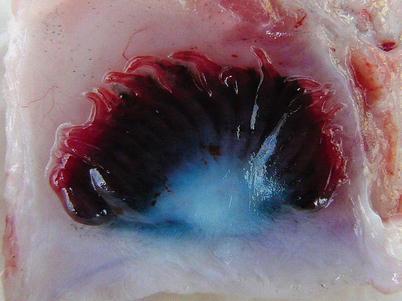
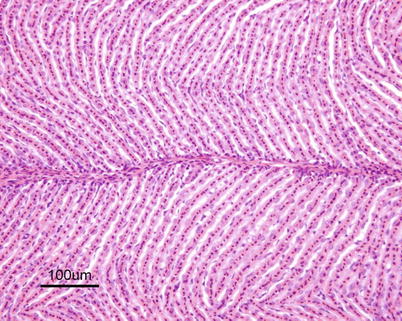
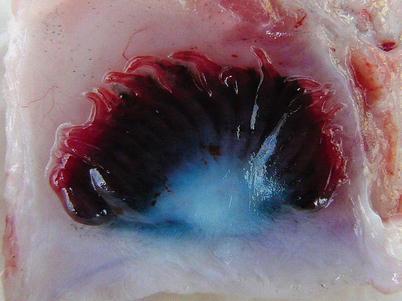
Fig. 2.6
Pseudobranch from an adult sea water farmed Atlantic salmon
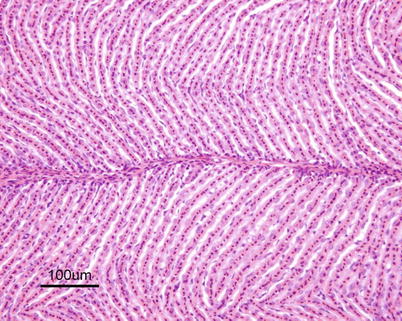
Fig. 2.7
Pseudobranch lamellae of adult Atlantic salmon
The gills are frequently involved in several pathological conditions of diverse aetiology, but lesions should be differentiated from artefacts and post-mortem changes. Due to their delicate structure, exposed location, abundant blood supply and large surface, gills quickly undergo post-mortem changes that can make histopathological interpretation difficult (see Fig. 4.31).
2.2 Circulatory System (Cardiovascular and Secondary Circulation)
2.2.1 The Cardiovascular System
The cardiovascular system is a simple loop with the heart, gills and systemic circulation in series. The deoxygenated blood is pumped from the ventricle to the gills where it leaves as oxygenated blood to be delivered directly to body organs and tissues.
The heart is located in the pericardial cavity antro-ventrally to the peritoneal cavity, and is separated from the latter by the septum transversum (Fig. 2.8). It consists of four compartments: the sinus venosus, the atrium, the ventricle and the bulbus arteriosus, interposed by the conus arteriosus. The former was considered to have been lost through evolution but recent work has clearly shown its presence and fundamental role in the heart outflow tract, where it supports the conus valves previously named bulbo-ventricular valves. Deoxygenated blood from the cardinal and hepatic veins flows into the thin-walled sinus venosus and passes through the sino-atrial valve and then into the thin-walled spongy atrium. Blood is drawn from the atrium via the atrio ventricular valves into the thick-walled and muscular ventricle. The ventricle has a pyramidal shape and consists of two muscular layers: the outer compact myocardium with its own supply of oxygenated blood, and the inner spongy myocardium (Fig. 2.9). The blood supply to the outer myocardium is via the coronary artery (Fig. 2.10), a branch of the hypobranchial artery from the second gill arch that runs caudally on the ventral side of the bulbus arteriosus, before it bifurcates and spreads over the ventricle surface. The inner spongy myocardium has no blood supply of its own, but is supplied with oxygen and nutrients from the venous blood being pumped through the organ. An example of the microanatomy of an artery and vein is shown in Fig. 2.11. The last chamber is the highly compliant bulbus arteriosus with thick walls composed of fibro-elastic and connective tissue. This chamber functions as a depulsator and delivers a steady blood flow to the ventral aorta. The adventitia of the organ consists of blood vessels and large nerve bundles in a collagen matrix. All chambers of the heart are covered by a flat epithelium called the epicardium which fuses with the pericardium that covers the inner surface of the pericardial cavity. All inner surfaces are covered by the endocardium. The thickness of the outer, compact myocardium may vary with age, sex and the habitat of the fish (Fig. 2.12). The absolute and relative thickness of the compact myocardium increases with age and is thicker in males than in females, and thicker in fish living in running water than those living in lakes. Generally, wild fish have a thicker compact myocardium than farmed fish of the same size. The cardiac striated muscle (myocardium) is differentiated from skeletal striated muscle by the branching structure of the fibres and centrally located nuclei (Fig. 2.13).
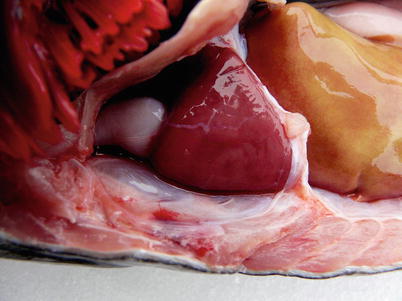
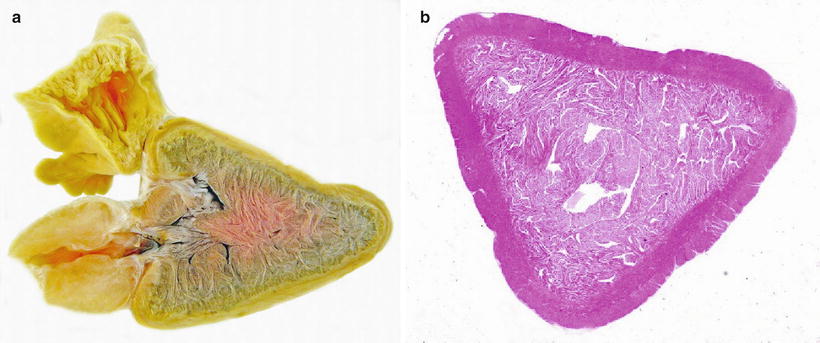
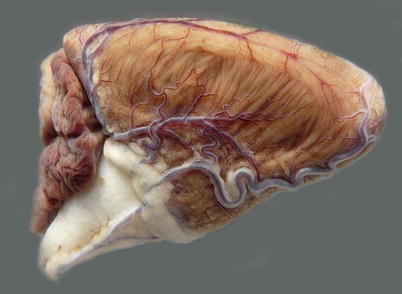
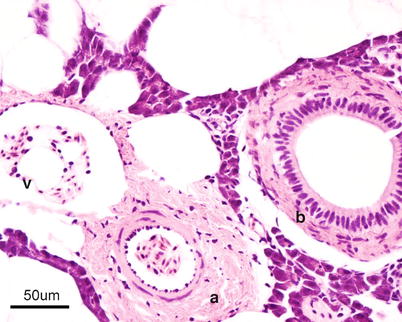
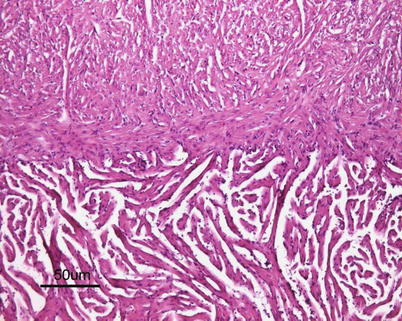
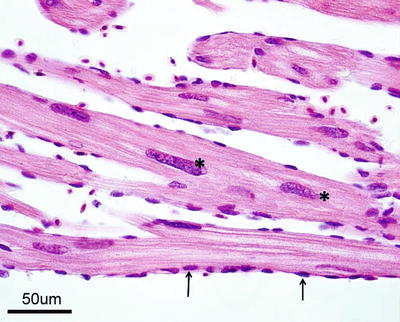
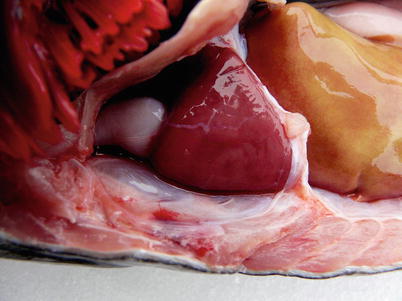
Fig. 2.8
Position of normal heart in the pericardial cavity of adult Atlantic salmon
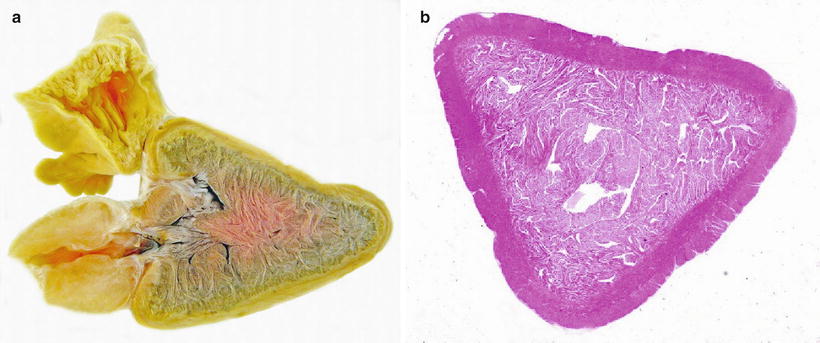
Fig. 2.9
(a) Sagittal section through the heart of wild Atlantic salmon showing atrium, ventricle with spongy and compact myocardium and bulbous arteriosus. Formalin fixed specimen. (b) Stained transverse section of ventricle from Atlantic salmon
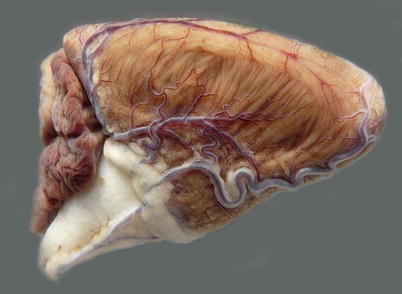
Fig. 2.10
Coronary vessels on the surface of bulbus arteriosus and the ventricle in adult farmed Atlantic salmon
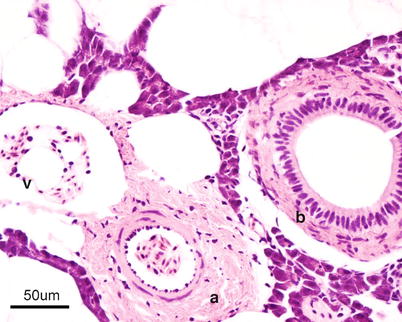
Fig. 2.11
Transverse section through thick walled artery (a) and thin walled vein (v) from Atlantic salmon, note presence of bile duct (b)
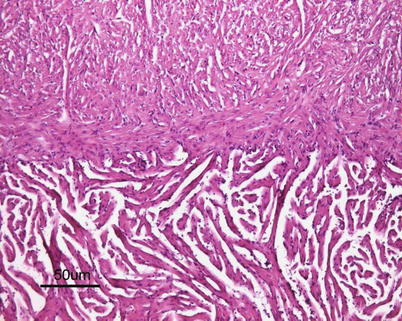
Fig. 2.12
Interphase between compact and spongy myocardium of ventricle of Atlantic salmon parr
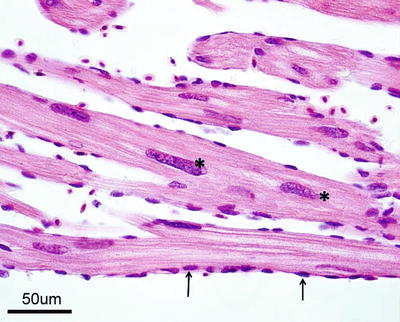
Fig. 2.13
Longitudinal section of spongy myocardium of Atlantic salmon; note central location of nuclei (*) and endocardial cell nuclei (arrows)
Blood Cells
Blood is composed of humoral (plasma) and cellular (blood cell) components. In contrast to mammals, fish red blood cells (erythrocytes) are nucleated and ovoid in shape, 13–16 μm long and 7–10 μm broad. Erythrocyte numbers may vary, but are usually in the range of 1.05 × 106–3.0 × 106 mm3. Giemsa staining shows that mature cells have a dense chromatin, purple-red centrally-located nucleus, and a clear homogenous, light red cytoplasm. The later reflects the absence of organelles and the quantity of haemoglobin present which in mature cells is very abundant. The peripheral blood is mainly composed of mature erythrocytes, although immature and developmental stages can be distinguished. Immature erythrocytes are known as reticulocytes, they are rounder with a relatively larger nucleus. Five categories can be recognized based on structure, distribution and quantity of basophilic substances within their cytoplasm. Normally, these represent about 1 % of the total count in healthy fish.
Lymphocytes in fish constitute 70–90 % of the total number of leucocytes. Lymphocytes are arbitrarily separated into categories of large (10–15 μm diameter) and small (7–10 μm diameter), which may represent different functional stages. The round or oval nucleus virtually occupies the whole cell leaving a narrow margin of basophilic cytoplasm. The cytoplasm may show pseudopodia-like projections on the surface. Both T and B lymphocytes forms are recognised in fish, playing a most significant role in the innate and the acquired immune responses.
Thrombocytes are responsible for blood clotting and are important in homeostasis and defence. Typically they are elongated but can be also be spindle-shaped and ovoid, with an indentation. They are of variable size (5–8 μm long) with a light basophilic rim of cytoplasm and a densely staining nucleus that occupies most of the cell. Between 1 and 6 % of the total white cells in rainbow trout are thrombocytes.
Neutrophils are morphologically similar to those in mammals and are commonly found at sites of inflammation. The eccentric nucleus is often kidney-shaped, although in mature cells two or five lobed nuclei may be recognized which are connected to each other by threads of nuclear material. These cells vary from 4 to 13 μm in diameter. There is evidence of phagocytic capacity in Atlantic salmon however much lower than the ‘professional’ macrophages, therefore unlikely that phagocytosis is their primary function. Their role seems to be more important in extracellular killing through enzymatic and other antimicrobial secretions.
Monocytes form about 0.1 % of the circulating leucocytes and are partially differentiated end cells which under appropriate conditions will develop into mature cells of the mononuclear phagocyte system. Monocytes are 9–25 μm in diameter with a lighter staining cytoplasm than small lymphocytes and contain small granules with a large nucleus.
Differentiation of phagocytes into macrophages usually takes place when they become extravascular, migrating from the vessels into the tissues and therefore usually not seen in circulation. Their phagocytic capability is well documented, but resting macrophages in tissues are difficult to distinguish from fibrocytes in H&E stained sections.
2.2.2 Secondary Circulation
Studies indicate that fish lack a true lymphatic system but a second vascular system derived from and connected to the primary circulation is present. Analogies between the lymphatic and the secondary system have been noted and observed in skin, fins, gills, oral mucosa and lining of the peritoneum, however it remains to be determined whether the secondary system is the antecedent of a lymphatic system or a coincidentally similar structure.
The spleen is usually dark red or almost black in colour and a discrete organ with sharply defined edges located near the greater curvature of the stomach. A thin serous capsule covers the surface. The spleen functions as a haematopoietic organ, a temporary blood bank and as a remover of circulating antigens and effete blood cells. Occasionally, two or more spleens may be recorded and the organ may also be located elsewhere in the abdominal cavity (Fig. 2.14). The spleen structure is provided by a capillary and a connective tissue meshwork among which the cells fill up the interspaces i.e. erythroblasts, mature and immature erythrocytes, lymphocytes, monocytes and macrophages. The parenchyma of the spleen is composed of white pulp, namely lymphoid tissue, surrounding small arteries which diffusely intermeshes with the haematopoietic red pulp, composed of a reticular cell network and supporting blood-filled sinusoids. There is no sharp demarcation between red and white pulp as the parts rich in erythrocytes and those rich in lymphocytes are intermingled (Fig. 2.15). The ellipsoids form the main elements of the spleen and are a thick-walled filter capillary network gradually forming from the artery which enters the organ. Each ellipsoid comprises a thick basement membrane-bound tube within which the vessels run and is separated from the membrane by a layer of sheathed components. Degradation products of senescent erythrocytes are stained yellow by H&E and known as haemosiderin, a common feature in the spleen parenchyma. Perl’s staining is used to differentiate haemosiderin deposits (see Fig. 4.22). Variable numbers of melanomacrophages may be found scattered in the spleen tissue. Phagocytic cells capable of trapping large quantities of particulate matter from the circulating blood, once replete migrate to the ellipsoids to the melanomacrophages.
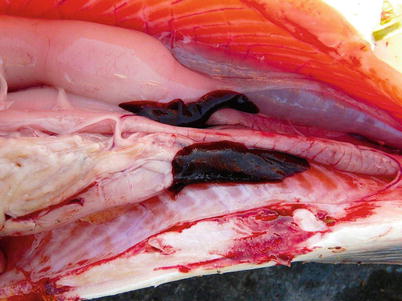
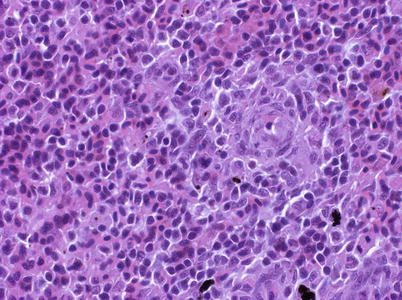
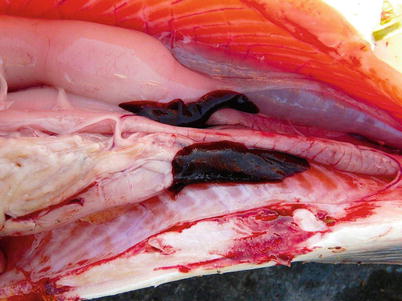
Fig. 2.14
Spleen in abdominal cavity of Atlantic salmon, note duplicate organ in this case
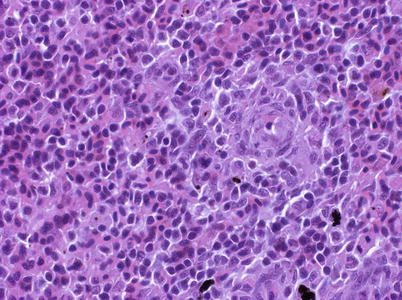
Fig. 2.15
Spleen of adult Atlantic salmon. Medium power
2.3 Integument System
The structure of the skin varies to some extent among species but is basically composed of two layers: an outer epidermis and an underlying dermis. The epidermis constitutes the barrier between the body and the aquatic environment and can be divided into two additional layers. The outer epithelium is composed of stratified squamous cells and a basal layer of undifferentiated cuboid germinal cells. The depth is greatest on the head and over the fins where scales are absent. The thickness of epidermis and number of mucous-secreting goblet cells in the epidermis varies between species and location on the body. Their number may increase during sexual maturation and spawning migration, and lymphocytes can also be present in the epidermis (Fig. 2.16). Mucous cells have characteristic basal, compact nuclei. The epidermis sits on a thin acellular layer that can be observed by light microscopy and is the fusion of the basal and the reticular lamina known as the basement membrane, although the components, i.e. the basal lamina, can only be observed by electron microscopy.
