37 Mark G. Papich The fluoroquinolone antibacterial agents are among the most important antimicrobials used in veterinary medicine. They are used in practically all species and have a broad spectrum of activity that includes most of the important veterinary bacterial pathogens. Since their first introduction in the late 1980s (discussed in previous editions of this book), the use of fluoroquinolones has expanded tremendously. The fluoroquinolones are synthetic antibacterial agents introduced in veterinary medicine first as enrofloxacin. Since then, there has been a great deal of research on this class of drugs to better understand their mechanism of action, antimicrobial spectrum, pharmacokinetics in a wide variety of animal species, and clinical uses. In addition, pharmaceutical companies have developed new agents in this class to increase the number of drug choices available to veterinarians. The advantages of the fluoroquinolones are that they are rapidly bactericidal against a wide variety of clinically important bacterial organisms, are potent, are well tolerated by animals, and have been administered via a variety of routes (orally via tablets and drinking water, subcutaneously, intravenously, intramuscularly, and topically). Fluoroquinolones approved for use in veterinary medicine for small animals are shown in Table 37.1. Fluoroquinolones that are approved for humans and are of potential interest for veterinary medicine include ciprofloxacin. The newest generation of fluoroquinolones, with increased activity against gram-positive cocci and anaerobic bacteria, includes levofloxacin, moxifloxacin, gatifloxacin, and the veterinary drug pradofloxacin. Table 37.1 Examples of fluoroquinolones used in veterinary medicine The currently available fluoroquinolones have the same quinolone structure (Figure 37.1). The addition of the fluorine group at position 6 gives these the characteristics of a fluoroquinolone, and various other chemical substitutions and side groups account for the different physical characteristics of each drug. Enrofloxacin has one fluorine substitution, difloxacin has two fluorine substitutions, and orbifloxacin has a three-fluorine substitution, but the presence of more than one fluorine does not increase antibacterial effects (Asuquo and Piddock, 1993). When lipid solubility is expressed as the octanol: water partition coefficient, enrofloxacin has among the highest lipophilicity. By comparison, ciprofloxacin has a partition coefficient that is approximately 100-fold less than that of enrofloxacin; the corresponding partition coefficients of orbifloxacin and marbofloxacin are slightly higher than that of ciprofloxacin (Asuquo and Piddock, 1993; Takács-Novák et al., 1992). But there are no studies available to show that these chemical differences among the drugs can account for differences in clinical response. However, chemical differences may account for some variation in absorption and distribution. For example, ciprofloxacin oral absorption is approximately one-half that of enrofloxacin in dogs; ciprofloxacin absorption in horses is less than 10%, compared to 60% for enrofloxacin. The less lipid-soluble fluoroquinolones (marbofloxacin, orbifloxacin) have a lower volume of distribution (Vd) than the ones with higher lipid solubility (enrofloxacin) (Table 37.2 ). One explanation for this observation is that the more lipid-soluble drugs have higher intracellular concentrations, but higher tissue binding also could explain the differences in volume of distribution. Figure 37.1 Structure of the fluoroquinolones. Features necessary for antibacterial activity are fluorine at position 6, ketone at position 4, and carboxyl at position 3. (Figure 37.2 shows other additions.) Addition of cyclopropyl, ethyl, or fluorophenyl at position 1 and of piperazine at position 7 increases the spectrum of antibacterial activity. Substitutions at position 8 broaden the spectrum of activity. Table 37.2 Interpretive categories for susceptibility break points (CLSI, 2015) *The breakpoint listed for ciprofloxacin is based on human use, not veterinary use. The most important structural difference in recent years is the addition of a substitution at position 8. These are usually referred to as third-generation fluoroquinolones to distinguish them from the second-generation drugs such as ciprofloxacin and enrofloxacin. For some human drugs, the addition is a methoxy (moxifloxacin), and for one of the veterinary drugs, it is a cyano group at position 8 that confers increased spectrum of activity. Quinolones are amphoteric molecules that can be protonated at the carboxyl and the tertiary amine portion of the molecule (Martinez et al., 2006). The pKa varies among the drugs slightly, but generally the pKa for the carboxyl group is 6.0–6.4 (5.5–6.3 in some references) and the pKa for the nitrogen of the piperazine group is 7.5–8 (Nikaido and Thanassi, 1993) (as high as 7.6–9.3 in some references). For two common drugs, enrofloxacin and ciprofloxacin, the pKa for the carboxyl group is 6.0 and 6.1, respectively, and 8.8 and 8.7 for the amine, respectively (Martinez et al., 2006). The isoelectric point is midway between the pKa for each ionizable group. Therefore, at physiological pH fluoroquinolones exist as zwitterions, in which both of the respective anionic and cationic groups are charged. It is at the isoelectric point (near the physiological pH) that fluoroquinolones are the most lipophilic and capable of diffusing across lipid membranes (Takács-Novák et al., 1992; Martinez et al., 2006). Figure 37.1 shows the basic quinolone structure. The carboxyl group at position 3 and the ketone at position 4 are necessary for the antibacterial activity. The fluorine at position 6 differentiates the quinolones from the fluoroquinolones and accounts for the improved gram-negative and gram-positive activity over the nonfluorinated quinolones, increased potency, and increased entry into bacteria. At position 1, addition of a cyclopropyl (as for enrofloxacin and ciprofloxacin in Figure 37.2), an ethyl or a fluorophenyl improve the spectrum of activity against gram-positive and gram-negative bacteria. Addition of a piperazine at position 7, as demonstrated for ciprofloxacin, marbofloxacin, and enrofloxacin (Figure 37.2), improves the spectrum of activity to include pseudomonads, among other gram-negative bacteria. The change from a nitrogen (nalidixic acid) to a carbon at position 8 decreased some of the adverse central nervous system effects and increased activity against staphylococci. Figure 37.2 Structure of the fluoroquinolones. These representative structures show modifications from the basic structure presented in Figure 32.1. Features necessary for antibacterial activity are fluorine at position 6, ketone at position 4, and carboxyl at position 3. Addition of cyclopropyl, ethyl, or fluorophenyl at position 1 and of piperazine at position 7 increases the spectrum of antibacterial activity. The third-generation fluoroquinolones have a bicyclic substitution at position 7, instead of a piperazine. This increased the activity to include a wider range of bacteria. In addition, a substitution at the 8 position on the ring enhances the bactericidal effects and improves the spectrum of activity to include more gram-positive and anaerobic bacteria. For example, the 8-methoxy substitution produces the drug moxifloxacin, a human quinolone with improved activity against gram-positive bacteria (Behra-Miellet et al., 2002; Aktaç et al., 2002; Pestova et al., 2000). Pradofloxacin, a veterinary quinolone with similar activity has a cyano substitution at position 8, which improves the activity against gram-positive and anaerobic activity compared to enrofloxacin and ciprofloxacin (Silley et al., 2007). This drug is discussed in more detail in Section Pradofloxacin Use in Dogs and Cats. A comparison of the activities of the veterinary fluoroquinolones against gram-positive and gram-negative bacteria can be illustrated in Figure 37.3 (using data from Wetzstein, 2005). Figure 37.3 Comparison of fluoroquinolone MIC values for veterinary drugs, and compared to two human drugs, moxifloxacin and ciprofloxacin. Source: Data from Wetzstein, 2005. Quinolones are bactericidal by inhibiting bacterial DNA replication and transcription. Two-stranded DNA is tightly coiled in the cell and must be separated for transcription and translation. To facilitate coiling, winding, and unwinding, the enzyme DNA gyrase allows the strands to be cut and reconnected. This allows coiling because negative supercoils can be introduced. DNA gyrase, a topoisomerase, consists of A and B subunits. The most common target site for quinolones is the A subunit of DNA gyrase coded by the gene gyrA. Mammals are resistant to the killing effects of quinolone antimicrobials because topoisomerase II in mammalian cells is not inhibited until the drug concentration reaches 100–1000 µg/ml. Bacteria are inhibited by concentrations of 0.1–10 µg/ml or less. Concentrations achieved in animals and people are much lower as seen by the Clinical Laboratory Standards Institute (CLSI, 2015) breakpoints (Table 37.2 ). Another target is the topoisomerase IV enzyme composed of subunits parC and parE. This site of action is less important for gram-negative bacteria but is a target of fluoroquinolones in some gram-positive bacteria such as streptococci and staphylococci (Ferrero et al., 1995). Among the newer generation fluoroquinolones – for example the veterinary drug pradofloxacin, and the human drugs moxifloxacin and gatifloxacin – the primary target for gram-positive bacteria may be the DNA-gyrase rather than topoisomerase IV, or these drugs also may be dual inhibitors against both targets (Intorre et al., 2007). Therefore, older fluoroquinolones have high activity against DNA-gyrase in gram-negative bacteria, but less against the topoisomerase IV of gram-positive bacteria. But the broader activity of newer fluoroquinolones can be attributed to higher affinity for both the DNA-gyrase in gram-negative and gram-positive bacteria, as well as activity for the topoisomerase IV of gram-positive bacteria (Drlica and Zhao, 1997; Blondeau et al., 2004). Fluoroquinolones in general exhibit good activity against most gram-negative bacteria, especially those of the Enterobacteriaceae. Representative minimum inhibitory concentration (MIC) values are shown in Table 37.3. Escherichia coli, Klebsiella spp., Proteus spp., Salmonella spp., and Enterobacter spp. are usually susceptible. Pseudomonas aeruginosa is variably susceptible and, when it is susceptible, usually has a higher MIC than other susceptible organisms. Against P. aeruginosa, ciprofloxacin is the most active (Rubin et al., 2008). Other bacteria susceptible to fluoroquinolones include intracellular organisms (Rickettsia spp., Chlamydia, and Mycobacterium spp.) and Mycoplasma spp. Table 37.3 Comparative microbiological data for common pathogens. Sources: Pirro et al., 1997, 1999; Asuquo and Piddock, 1993; Stegemann et al., 1996; Spreng et al., 1995; pradofloxacin FOI summary, and manufacturer data. MIC values listed are MIC90 and represent an average from available published literature or manufacturer technical information. Gram-positive bacteria are variably susceptible. Staphylococcus aureus, Staphylococcus pseudintermedius, and other Staphylococcus species usually are susceptible. However, the MIC values for staphylococci typically are higher than for gram-negative bacteria, and staphylococcal resistance to fluoroquinolones can be common. In general, ciprofloxacin is more active than enrofloxacin against gram-negative bacilli, and enrofloxacin is more active against Staphylococcus species (Grobbel et al., 2007; Blondeau et al., 2012). Marbofloxacin activity usually falls between enrofloxacin and ciprofloxacin. The newer fluoroquinolones, such as moxifloxacin, gatifloxacin, and the veterinary drug pradofloxacin, have increased activity against gram-positive cocci and anaerobic bacteria (Behra-Miellet et al., 2002; Aktaç et al., 2002; Pestova et al., 2000; Silley, 2007) (Figure 37.3). Factors that may affect activity are cations at the site of infection and low pH. Cations such as Al+3, Mg+3, Fe+2, and Ca+2 can bind a carboxyl group on the drug and significantly decrease activity. Low pH at the site of action also can affect the MIC (Ross and Riley, 1994), especially for drugs that have a piperazine at position 7 (Figures 37.1 and 37.2). For example, in urine, the MIC for fluoroquinolones may increase due to the presence of cations in the urine and low pH (Fernandes, 1988). This activity in urine may increase the MIC from fourfold to 64-fold. Fluoroquinolone activity in an abscess is not diminished despite the observation that in pus there is cellular material that can bind drugs, a low pH, and slow-growing bacteria (Bryant and Mazza, 1989). The presence of a foreign body, albumin, globulin, pus, anaerobic conditions, and dead bacteria did not affect the activity of fluoroquinolones (Rubinstein et al., 2000). The activity of fluoroquinolones in this milieu may explain its efficacy for treating infections associated with abscessation. Susceptibility testing is performed either by the agar-disk-diffusion (ADD) method or broth dilutions (MIC test). The CLSI (CLSI, 2015) has published interpretive categories for breakpoints for most fluoroquinolones (Table 37.2 ). For isolates in the “intermediate” range, this is a category that implies that an infection due to the isolate may be appropriately treated in body sites where the drugs are physiologically concentrated or when a high dosage of drug can be used. Therefore, the dose can be increased in these instances, or successful treatment might be possible of the drug is applied topically or for a urinary tract infection. The MIC quality control ranges for wild-type organisms are also available from CLSI. There are multiple resistance mechanisms that have been identified for fluoroquinolones. These include: decreased drug permeability, increased drug efflux (efflux pumps), gyrase protecting enzymes, altered targets, and plasmid-mediated resistance. Of all the mechanisms listed, resistance most frequently develops via the gyrA mutation that codes for the A subunit of the DNA gyrase enzyme. A mutation at the serine-83 residue has been one of the most common, but at least 10 additional mutations at the gyrA gene have been identified to confer resistance (Ferrero et al., 1995). A mutation in the parC gene that codes for topoisomerase IV enzyme also is important (grlA mutation in staphylococci). A mutation in parC coding for resistant topoisomerase IV causes resistance when detected with mutations of gyrA, thus presence of both mutations usually produces high-level resistance. The multidrug resistance membrane efflux mechanisms reduce the accumulation of antibiotics in the bacteria. These efflux mechanisms are known to produce a high-level resistance to fluoroquinolones when they are present with other target site mutations (Zhanel et al., 2004). Because the efflux membrane pumps may affect other drugs, this may produce cross-resistance to other antimicrobials. Generally, there is cross-resistance among the fluoroquinolones. That is, although MIC values among susceptible strains can vary by a few dilutions, a fully resistant strain will typically be resistant to all the fluoroquinolones. Resistance can occur through a multistep process (Everett et al., 1996). A single mutation can increase the MIC slightly (perhaps one dilution), and each subsequent mutation produces a progressively higher level resistance in a stepwise fashion. Unlike plasmid-mediated bacterial resistance, in which resistance may disappear after selective antibiotic pressure is removed, chromosomal (mutational) resistance exhibited by fluoroquinolone-resistant bacteria can be maintained in bacteria after drug administration is discontinued. Plasmid-mediated resistance has been found in E. coli and Klebsiella organisms, but the clinical significance of plasmid-mediated resistance has not been identified (Martinez-Martinez et al., 1998). Resistance to fluoroquinolones has become a problem in human medicine and some investigators have attributed this to over-prescribing of these drugs. Resistance to fluoroquinolones by E. coli, Staphylococcus aureus, and Streptococcus pneumoniae has been well documented (Chen et al., 1999; Murphy et al., 1997; Sanders et al., 1995; Neu, 1992; Peña et al., 1995; Perea et al., 1999; Everett et al., 1996). Clinical resistance in human hospitals among staphylococci appeared relatively quickly after introduction of ciprofloxacin (Neu, 1992; Sanders et al., 1995; Hedin and Hambreus, 1991). Resistant bacteria also have been identified in practically all animal species. Resistance in small animals has been documented for E. coli, P. aeruginosa, Enterobacter, Proteus, and other gram-negative bacteria. Resistance among staphylococci isolated from small animals has also been documented, particularly with methicillin-resistant Staphylococcus pseudintermedius (MRSP) (Couto et al., 2014; Bemis et al., 2009; Jones et al., 2007; Cole et al., 1998). The acquisition of specific gryA/grlA genes associated with phenotypic resistance to fluoroquinolones is strongly correlated with multidrug resistant methicillin-resistant Staphylococcus pseudintermedius (MRSP) (McCarthy et al., 2015). Pseudomonas organisms have been particularly troublesome because single-step mutations are common for this bacteria, which can easily progress to high-level resistance. This is an important problem in companion animal medicine because, except for the fluoroquinolones, there are no other oral drugs with which to treat infections caused by Pseudomonas aeruginosa. Factors leading to resistant P. aeruginosa are an inadequate dosage and extended treatment at low doses. Various studies have confirmed increased frequency of resistance among Pseudomonas aeruginosa isolated from chronic infections in dogs (Petersen et al., 2002; Martin Barrasa et al., 2000; Cole et al., 1998). Infectious disease experts have warned that administration of fluoroquinolones to animals may be a public health concern. Transfer of fluoroquinolone resistance from animals to people has been suggested to occur for Campylobacter species (Endtz et al., 1991) and Salmonella typhimurium type DT-104 (Threlfall et al., 1995, 1997; Griggs et al., 1994). An increase in the incidence of resistant Campylobacter jejuni infecting people was linked to consumption of Campylobacter-contaminated chicken. The increased resistance occurred primarily after 1995, which coincides with the time that fluoroquinolones were approved for use in poultry as an additive to drinking water (Smith et al., 1999). Investigators have also associated resistance in salmonellae with veterinary use of fluoroquinolones in livestock (Piddock et al., 1998). However, resistant strains of Salmonella typhimurium may have occurred spontaneously because some of the resistant salmonellae have come from farms in which fluoroquinolones were not administered to animals (Griggs et al., 1994). Nevertheless, some scientists have warned that use of fluoroquinolones in livestock is a public health risk because it can potentially lead to resistant mutants of salmonellae being passed on to humans through the food chain. Because of these concerns, there have been limited approvals of fluoroquinolones for food-producing animals, and the extralabel use of fluoroquinolones is prohibited in food-producing animals in the United States. Because of the risk of Campylobacter resistance, all fluoroquinolone formulations for poultry have been removed in the USA. In order to provide continued monitoring of fluoroquinolone resistance bacteria from food animals, the National Antimicrobial Resistance Monitoring System (NARMS) performs routine surveillance of antibiotic resistance data from human clinical samples, slaughter samples and retail meat samples. In their most recent report (available at: http://www.cdc.gov/narms/), NARMS concluded that “Overall, ciprofloxacin resistance has been consistently low among Salmonella isolated from all sources. Similarly, ciprofloxacin resistance in E. coli was absent or very low (0–1.7%) in isolates from retail meats, chicken and cecal samples.” They also found that for Campylobacter jejuni in retail chicken samples, resistance to ciprofloxacin was at its lowest level to date (11%), while ciprofloxacin resistance in C. jejuni isolated from chicken slaughter samples has not declined (22% in 2013). Extensive pharmacokinetic data is provided in Table 37.4. These are presented as examples because there are far more pharmacokinetic studies available in the literature than can be presented in this table. A literature search of pharmacokinetic studies in animals will reveal many available studies in the entire array of species treated by veterinarians – ranging from large animal species of elephants and whales, to mice and small invertebrate species. Table 37.4 Pharmacokinetic comparison of fluoroquinolones 4.4 (IV) 2.7 (oral) 9.5 (IV) 11 (oral) 2.1 at 5 mg/kg, 2.9 at 7.5 mg/kg 69 tablets, 58% suspension 5.0 (IV, IM) 5.0–7.5 (oral) 0.78 (5 mg/kg) 4.9 (IV) 10.7 (IM) 1.68 (IV) 5.9 (IM) 5.55 (SC) 0.73 (IM) 0.98 (SC) 5.0 mg/kg q48h IM 1.64 (IM) 0.8 (oral) 5.0 mg/kg q24h oral 0.29 (oral) 0.54 (oral) 89.0 (IP) 46 (oral) Na = Data not available or not applicable. Nd = Not determined, NR = not recommended. t1/2 = Half-life of the terminal portion of the plasma concentration vs. time curve. AUC = Area under the curve of the plasma concentration vs. time curve. Cmax = Maximum plasma concentration after administration of oral or IM dose. %F = Percentage of oral or IM administered dose absorbed (determined from comparison of IV dose). aHalf-life, volume of distribution, and AUC are from an IV dose unless otherwise noted. Apparent volume of distribution is typically from the area method. Where the dose was administered by a non-IV route, the Vd reported is Vd/F. bAssay type = Assay using HPLC is able to distinguish between enrofloxacin and ciprofloxacin, and values shown in table represent enrofloxacin. Assays performed by bioassay represent the parent drug and active metabolites. Bioassay may include concentrations of ciprofloxacin. cRegistered dose with the FDA in the United States. In some European countries doses may vary or may not include the flexible range. In most cases, when treating non-Pseudomonas infections, the lowest dose in the range listed is used. dAfter multiple dosing with ciprofloxacin, the Cmax was 1.18 mg/ml and the 12 hr AUC was 9.58 mg/hr/ml. eOral absorption of ciprofloxacin estimated from a comparison of independent oral and IV studies. fThese parameters determined after administration of 5.8 mg/kg of enrofloxacin. Note that in enrofloxacin studies, ciprofloxacin also was detected at varying concentrations (depending on species and study). Ciprofloxacin pharmacokinetics were measured in those studies but is not reported in this table. The ciprofloxacin data can be found in the studies referenced. Mammals are relatively consistent in elimination half-life and volume of distribution. Reptiles with lower renal clearance generally demonstrate longer half-lives – as long as 55 and 36 hours for enrofloxacin in alligators and monitor lizards, respectively (Papich, 1999). There may be an allometric relationship in pharmacokinetic parameters among mammals ranging in size from mice to cattle (Bregante et al., 1999; Cox et al., 2004). The allometric relationship was improved considerably when the pharmacokinetic parameters were corrected for the percentage of protein-unbound enrofloxacin in the plasma (Table 37.5 ). Table 37.5 Plasma/serum protein binding of fluoroquinolones in animals (% bound) Sources: Villa et al., 1997; Gavrielli et al., 1995; Nouws et al., 1988; Aramayona et al., 1994; Idowu et al., 2010; Davis et al., 2007; Bidgood and Papich, 2005, and author Papich’s unpublished data. When two or more values are listed, they represent results from independent studies. Because most of these drugs are administered either once daily or as a one-time administration, the elimination half-lives shown in Table 37.4 may have little effect on clinical outcome. For example, it does not appear that differences in half-life can account for different clinical results for skin infection treatment in dogs because enrofloxacin, which has the shortest half-life, and marbofloxacin, which has a long half-life, have both been reported to be effective when administered once daily (Paradis et al., 1990; Carlotti et al., 1995; Carlotti, 1996; Gruet et al., 1997; Koch and Peters, 1996; Ihrke, 1996, 1998; Cester et al., 1996). In cattle and pigs, enrofloxacin is approved for either a one-time dose of 7.5–12.5 mg/kg, or 2.5–5 mg/kg once daily for three to five treatments. With either regimen, the outcome is the same. Likewise, danofloxacin is approved for use in cattle at a dose of 6 mg/kg administered twice, or a single dose of 8 mg/kg, with similar outcomes. Although the volume of distribution varies among animals (and among studies), these differences have not translated into differences in clinical efficacy. Marbofloxacin and orbifloxacin, which have a volume of distribution in the range of 1–2– l/kg, achieve effective skin concentrations and appear as clinically effective as drugs such as enrofloxacin with a volume of distribution of 2.5–5 l/kg. Heinen (2002) demonstrated that even though approved doses may vary among enrofloxacin, marbofloxacin, and orbifloxacin in dogs, they are all capable of achieving pharmacokinetic–pharmacodynamic (PK-PD) targets (these targets are discussed in Section Pharmacokinetics / Pharmacodynamics). The data published (Bidgood and Papich, 2005, Walker et al., 1990, 1992; Messenger et al., 2012; Davis et al., 2007) or available from manufacturer technical information show that the fluoroquinolones, regardless of their volume of distribution and lipophilicity, achieve effective concentrations in tissues. Some exceptions are tissues not easily penetrated such as the central nervous system and eye. Whether fluoroquinolones are administered with or without food has little effect on oral absorption. Feeding may delay the time to peak concentration (Tmax), but this has little effect on clinical outcome because the extent of absorption, determined by the total AUC (area under the curve of the plasma concentration versus time curve) is not affected significantly. Administration with food has prolonged the terminal half-life when enrofloxacin was administered orally to reptiles (Papich, 1999), sheep, pigs (Gyrd-Hansen and Nielsen, 1994), and chickens (Anadón et al., 1995). This may be caused by a “flip-flop” pharmacokinetic effect introduced in Chapter 3 and discussed below in this section. In clinical situations involving animals difficult to dose, fluoroquinolones may be added to a patient’s food in order to provide a more convenient dosing form. For example, enrofloxacin tablets were placed in whole fish, which were fed to dolphins to produce good absorption (Linnehan et al., 1999), and enrofloxacin was injected into mice and fed to monitor lizards, also producing good absorption (Hungerford et al., 1997). Chewable tablets of enrofloxacin (Taste-Tabs) do not affect oral absorption (manufacturer’s data). In dogs, cats, and pigs, oral absorption of fluoroquinolones approaches 100% (Table 37.4 ), but in large animals, the extent of absorption has been less. Oral absorption of fluoroquinolones is variable in horses. Ciprofloxacin showed an oral absorption of only 6.8% in ponies (Dowling et al., 1995) and only 10% (mean) in adults (Yamarik et al., 2010). But enrofloxacin absorption is 63% (Giguère et al., 1996) in adult horses and 42% in foals (Bermingham et al., 2000). The value reported for adult horses is probably artificially high because the study used a bioassay that overestimates the concentration of enrofloxacin in plasma. With the exception of ciprofloxacin, other studies listed in Table 37.4
Fluoroquinolone Antimicrobial Drugs
Drug
Brand name(s)
Approved species
Enrofloxacin
Baytril, and generic
Dogs, Cats, Cattle, Pigs
Danofloxacin
Advocin
Cattle
Orbifloxacin
Orbax
Dogs, Cats
Pradofloxacin
Veraflox
Cats, (also dogs in some countries)
Marbofloxacin
Zeniquin, Marbocyl
Dogs, Cats (other species outside the U.S.)
Ciprofloxacin
Cipro and generic
Not approved for animals; human drug
Ibafloxacin
Ibaflin
No longer available (formerly dogs, cats)
Difloxacin
Dicural
No longer marketed (formerly dogs, and in some countries, cattle, and poultry)
Chemical Features
Drug
Species
Susceptible (μg/ml)
Intermediate (μg/ml)
Resistant (μg/ml)
Enrofloxacin
Dogs, cats
≤0.5
1–2
≥ 4
Orbifloxacin
Dogs, cats
≤1.0
2–4
≥ 8
Ciprofloxacin*
People
≤1.0
2
≥ 4
Marbofloxacin
Dogs, cats
≤1.0
2
≥ 4
Enrofloxacin
Pigs (gram negative)
≤0.25
0.5
≥ 1
Enrofloxacin
Pigs (gram positive)
≤0.5
1
≥ 2
Enrofloxacin
Horses
≤0.25
0.5
≥ 0.5
Enrofloxacin
Cattle (BRD)
≤0.25
0.5–1
≥ 2
Pradofloxacin
Dogs, Cats
≤0.25
0.5–1
≥ 2
Danofloxacin
Cattle (BRD)
≤0.25
0.5
≥ 1
Structure–Activity Relationships
Mechanism of Action
Antibacterial Spectrum
MIC of bacteria (µg/ml)
Drug
Pasteurella multocida
Escherichia coli
Staphylococcus pseudintermedius
Pseudomonas aeruginosa
Ciprofloxacin
0.015
0.03
0.25
0.5
Difloxacin
<0.05
0.11–0.23
0.25–0.91
0.92
Enrofloxacin
0.03
0.03–0.06
0.125
2.0
Marbofloxacin
0.04
0.125–0.25
0.23–0.25
0.94
Orbifloxacin
0.05
0.125–0.39
0.25–0.39
6.25–12.5
Pradofloxacin
0.008
0.03
0.06
Susceptibility Testing
Resistance
Clinical Resistance Problems
Human Health Risks from Drug Resistant Bacteria
Pharmacokinetics
Drug
Dose studied (mg/kg)
Recommended daily dose (mg/kg)
Half-lifea (hr)
Volume of Distributiona (L/kg)
Peak Concentration (Cmax) (µg/ml)
AUCa (µg · hr/ml)
%F
Assayb
Reference
Dogs
Enrofloxacin
5
5
4.61
ND
1.75
11.65
Nd
HPLC
Frazier et al., 2000
Enrofloxacin
5
5
2.23
3.64
1.24
4.46
63.22
HPLC
Bidgood and Papich, 2005
Enrofloxacin
5.0 IV
5.0
2.7–3
5.0–5.6
—
4.05–4.34
—
HPLC
Intorre et al., 1995
Enrofloxacin
5.0
5.0
2.52
2.5
1.12 (oral)
7.27
72.3
HPLC
Cester et al., 1996
Enrofloxacin
5.8
5.0
4.5
1.44 (oral)
8.2
83.0
HPLC
Monlouis et al., 1997
Enrofloxacin
5.5
2.75–11.0
4.0 (oral)
nd
2.45 (oral)
16.32
Nd
Bioassay
Walker et al., 1992
Enrofloxacin
5.0
5.0
2.4
4.5
1.16 (oral)
3.9
100
HPLC
Küng et al., 1993a
Enrofloxacin
5.0
5.0–20.0c
4.8
4.2
1.6
8.15
—
HPLC
Stegemann et al., 1996
Ciprofloxacin
5.0
nd
3.17
2.23
0.35
4.18
43.0
HPLC
Cester et al., 1996
Ciprofloxacin
5.8f
nd
5.2
nd
0.34
7.2
Nd
HPLC
Monlouis et al., 1997
Ciprofloxacin
10.0
10.0–20.0
2.4
3.0
—
12.93
—
HPLC
Abadia et al., 1994
Ciprofloxacin
10.0
10.0–20.0
7.5
—
1.18 (oral)d
9.58 (oral)d
46e
Bioassay
Walker et al., 1990
Ciprofloxacin
25 (oral)
25–30 once daily
2.59
Na
4.44
22.5
58.36
HPLC
Papich, 2012
Ciprofloxacin
10 IV
15 IV once daily
3.72
2.39
Na
16.67
Na
HPLC
Papich, 2012
Difloxacin
5.0
5.0–10.0c
9.3
4.63
1.8 (oral)
12.93
96.0
nd
Manufacturer’s data
Orbifloxacin
2.5
2.5–7.5c
5.6
1.5
2.33 (oral)
14.3
97–100
HPLC
Manufacturer’s data
Marbofloxacin
5
5
7.63
1.54
3.63
42.08
104.6
HPLC
Bidgood and Papich, 2005
Marbofloxacin
2 SC
2 SC
13
Na
1.52
19.76
98.7
HPLC
Schneider et al., 1996
Marbofloxacin
4 SC
4 SC
13.4
Na
3.04
41.54
HPLC
Schneider et al., 1996
Marbofloxacin
4 oral
4 oral
12.5
Na
2.93
45.60
107
HPLC
Schneider et al., 1996
Marbofloxacin (2 studies)
2 IV
2 IV
12.4, 13.9
1.90, 2.25
Na
20.95, 18.60
Na
HPLC
Schneider et al., 1996
Marbofloxacin
2 oral
2 oral
14.0
Na
1.38
22.01
105
HPLC
Schneider et al., 1996
Marbofloxacin
2.0
2.0
9.8
1.4
1.35 (oral)
23.31
99.8
HPLC
Cester et al., 1996
Marbofloxacin
5.55 and 2.8 mg/kg
2.75–5.55c
1.27
2.0 at 2.8 mg/kg oral; 4.2 at 5.55 mg/kg oral
59.0
94.0
HPLC
Manufacturer’s data
Marbofloxacin
5
5
4.07
Nd
1.41
8.74
Nd
Bioassay
Heinen, 2002
Marbofloxacin
2
2
9.07
Nd
1.47
13.07
Nd
Bioassay
Heinen, 2002
Marbofloxacin
2.75
2.75
10.9
Nd
2.53
35.44
Nd
HPLC
Frazier et al., 2000
Orbifloxacin
2.5
2.5
2.42
Nd
1.37
12.72
Nd
Bioassay
Heinen, 2002
Difloxacin
5
5
6.9
Nd
1.1
9.34
Nd
Bioassay
Heinen, 2002
Pradofloxacin
3
3–5
7.2–8
2.22
1.6
13.7
100
HPLC
Manufacturer’s data
Cats
Levofloxacin
10
10
8.37
1.57
4.38
57.5
71.2
Bioassay
Albarellos et al., 2004
Enrofloxacin
5
5
6.7
2.5
1.0
20.3
107
HPLC
Seguin et al., 2004
Enrofloxacin (kitten)
5
5
3.7–6.3
1.8–3.9
0.5–1.1
5–12.7
33.7–72.8
HPLC
Sequin et al., 2004
Enrofloxacin
4.7
5c
6.7
6.3
1.66 (oral)
7.2
100
HPLC
Richez et al., 1997b
Orbifloxacin
2.5
2.5–7.5c
5.5
1.4
2.06
10.82
100
HPLC
Manufacturer’s data
Ciprofloxacin
10 oral
10 mg/kg q12h oral
2.37
12.52
0.73
3.17
33
Bioassay
Albarellos et al., 2004
Ciprofloxacin
10 IV
10 mg/kg q12h
4.53
3.85
Na
17.20
Na
Bioassay
Albarellos et al., 2004
Pradofloxacin
5, 7.5 oral
5–7.5
7.3
4.45
9.4 at 5 mg/kg; 13.3 at 7.5 mg/kg
HPLC
Manufacturer’s data
Horses
Moxifloxacin
5.8
NR
34
5.3
3.12
50.9
Nd
HPLC
Gardner et al., 2004
Ciprofloxacin
5
NR
5.8
4.9 (Vdss)
0.6 (PO)
8.1
10.5
HPLC
Yamarik et al., 2010
Enrofloxacin
7.5
7.5 (oral)
5.9 (IV)
4.2
2.22
14.37
65.6
HPLC
Boeckh et al., 2001
Enrofloxacin
7.5
7.5 (oral)
5.3 (IV)
2.9
1.85
21.0
78.3
Bioassay
Haines et al., 2000
Enrofloxacin
5
5 (IV)
6.7
1.9
12.4 (IV)
25.3
Nd
HPLC
Papich et al., 2002
Enrofloxacin
2.5 and 5.0
5.9–6.1
5.44
58.3
62.5
Bioassay
Giguère et al., 1996
Enrofloxacin (foals)
5.0
2.5–5.0
16.5
2.31
2.12 (10 mg/kg oral)
48.54
42.0
HPLC
Bermingham et al., 2000
Enrofloxacin
5
Nd
6.15 (IV)
2.32 (Vdss)
1.0 (approx)
13.8
55
HPLC
Peyrou et al., 2006
Marbofloxacin
2
Nd
7.42 (IV)
1.6 (Vdss)
0.8 (approx)
7.36
59
HPLC
Peyrou et al., 2006
Marbofloxacin
2
2
4.74 (IV)
1.17 (Vdss)
1.42 (IM)
11.27
87.9 (IM)
HPLC
Carretero et al., 2002
Marbofloxacin
2
2
7.56 (IV)
2.83
0.89 (PO)
8.26
62 (PO), 98 (IM)
HPLC
Bousquet-Melou et al., 2002
Ciprofloxacin
3.0
Not recommended
0.147
0.77 (IM)
6.97
96.0 (IM)
HPLC
Yun et al., 1994
Ciprofloxacin
5.0
Not recommended
2.6 (IV)
3.88
Na
4.83
6.8
Bioassay
Dowling et al., 1995
Ciprofloxacin
5 IV
Not recommended
5.80
4.93
Na
8.13
Na
HPLC
Yamarik et al., 2010
Ciprofloxacin
20 oral
Not recommended
3.63
38.075
0.63
3.30
10.47
HPLC
Yamarik et al., 2010
Orbifloxacin
2.5
2.5–5.0 (oral)
5.1
2.4
1.25 (oral)
9.06
68.3 (oral)
HPLC
Davis et al., 2006
Cattle
Enrofloxacin
12.5
12.5
6.79
ND
0.96
14.95
ND
HPLC
Davis et al., 2007
Enrofloxacin
7.5
7.5
9.23
–
0.89
17.79
ND
HPLC
Foster et al., 2016b
Enrofloxacin
7.5
7.5
4.53
–
1.07
13.22
ND
HPLC
Foster et al., 2016b
Enrofloxacin
12.5
12.5
6.79
–
1.82
31.13
ND
HPLC
Foster et al., 2017)
Enrofloxacin
8
8
7.28
NS
0.81
7.51
ND
HPLC
TerHune et al., 2005
Enrofloxacin (1-day-old calves)
2.5
2.5–5 per day or 7.5–12.5 SC once
6.61
1.70
nd
13.94
nd
HPLC
Kaartinen et al., 1997
Enrofloxacin (1-week-old calves)
2.5
2.5–5 per day or 7.5–12.5 SC once
4.87
2.61
nd
6.73
nd
HPLC
Kaartinen et al., 1997
Enrofloxacin (lactating cows)
5.0
nd
1.63
7.42
82.0 (IM) 137.0 (SC)
HPLC
Kaartinen et al., 1995
Enrofloxacin (cows)
2.5
nd
2.82
2.98
nd
5.28
nd
HPLC
Bregante et al., 1999
Enrofloxacin (adult cattle)
5.0
2.5–5 per day or 7.5–12.5 SC oncec
2.3
1.65
0.73 (SC)
10.08
88.0 (SC)
HPLC
Richez et al., 1997a
Enrofloxacin (calves)
5.0
2.5–5 per day or 7.5–12.5 SC oncec
2.2
1.98
0.87 (SC)
7.99
97.0 (SC)
HPLC
Richez et al., 1997a
Enrofloxacin (beef steers)
5 IV
Na
5.15
1.59
Na
7.18
Na
HPLC
Idowu et al., 2010
Enrofloxacin (dairy cows)
5 IV
Na
3.69
1.56
Na
3.62
Na
HPLC
Idowu et al., 2010
Ciprofloxacin (calves)
2.8
nd
2.4
2.5
0.27
nd
53.0 (oral)
HPLC
Nouws et al., 1988
Danofloxacin
6
6
4.21
ND
1.7
9.72
ND
HPLC
TerHune et al., 2005
Danofloxacin
1.25
Nd
2.25
2.04
0.28
132.85
289
Bioassay
Shem-Tov et al., 1998
Danofloxacin
5
Nd
2.9
ND
0.82
4.7
78 (IM)
HPLC
Mann and Frame, 1992
Danofloxacin
1.25
Nd
6.26
4.0
0.18
2.9
91
HPLC
Apley and Upson, 1993
Marbofloxacin
10 IM
10 IM, once
17.5
Nd
7.92
52.66
Na
HPLC
Vallé et al., 2012
Marbofloxacin (Buffalo calves)
4 SC
4 SC x 3 days
7.37
Nd
1.63
14.17
Na
HPLC
Baroni et al., 2014
Goats
Danofloxacin
1.25
Nd
4.67
3.8
0.33
2.29
110 (SC)
HPLC
Aliabadi and Lees, 2001
Enrofloxacin
5
5
1.1
1.3
6.7
6.7
ND
HPLC
Rao et al., 2000
Marbofloxacin
2
2
7.2
1.3 (Vdss)
1.9
8.44
100.7 (IM)
HPLC
Waxman et al., 2001
Sheep
Enrofloxacin
5
10
4.8
1.15
2.69
57.5
98.07
HPLC
Bermingham and Papich, 2002
Enrofloxacin
2.5
5.0 mg/kg/day SC
3.73
2.18
0.78 (IM)
5.47
85.0 (IM)
HPLC
Mengozzi et al., 1996
Enrofloxacin
2.5
5.0 mg/kg/day SC
3.8
1.3
0.6 (oral)
10.4
60.6 (oral)
Bioassay
Pozzin et al., 1997
Enrofloxacin
2.5
nd
2.5
1.53
nd
8.98
nd
HPLC
Bregante et al., 1999
Alpacas
Enrofloxacin
5 and 10
5–10
13.0
1.6
7.8 (SC), 15.3 (PO)
58.4
90 (SC), 29 (PO)
HPLC
Gandolf et al., 2005
Elephants
Enrofloxacin
2.5 (PO)
2.5 (PO)
18.4
Nd
1.31
34.93
Nd
HPLC
Sanchez et al., 2005
Mice
Enrofloxacin
10.0
nd
1.48
10.5
nd
2.45
na
HPLC
Bregante et al., 1999
Rats
Enrofloxacin
7.5
nd
1.8
4.78
nd
5.65
nc
HPLC
Bregante et al., 1999
Rabbits
Enrofloxacin
7.5
nd
2.2
4.94
nd
5.52
nd
HPLC
Bregante et al., 1999
Enrofloxacin
7.5 (IV)
nd
1.87
3.97
na
5.38
na
HPLC
Aramayona et al., 1996
Enrofloxacin
5.0 (IV)
5.0
2.18 (IV)
4.4
na
3.89
na
HPLC
Cabanes et al., 1992
Enrofloxacin
5.0 (IM)
5.0
1.8
na
3.04
3.84
92.0
HPLC
Cabanes et al., 1992
Enrofloxacin
5.0 (IV)
5.0
2.5
2.12
na
8.6
na
HPLC
Broome et al., 1991
Enrofloxacin
5.0 (oral)
5.0
2.4
na
0.45
5.4
61.0
HPLC
Broome et al., 1991
Moxifloxacin
5
nd
1.84
2.12
1.3 (PO)
6.28
75.5 (PO)
HPLC
Fernández-Varón et al., 2005
Marbofloxacin
2 (IM)
2
4.33
1.65
1.8
12.44
100
HPLC
Abo-El-Sooud and Goudah, 2010
Marbofloxacin
5 oral
5 oral
8
Na
1.73
10.5
Na
LC-MS
Carpenter et al., 2009
Chickens
Ciprofloxacin
5.0
5.0–15.0 IM, SC, oral
9.01
2.02
4.67
78.04
70.0
Bioassay
Atta and Sharif, 1997
Enrofloxacin
10.0
10.0
5.6 (IV)
5.0
1.88 (oral)
16.17
89.2
HPLC
Knoll et al., 1999
Ciprofloxacin
5.0
5.0
9.0
2.0
4.67
78.04
70.0 (oral)
Bioassay
Atta and Sharif, 1997
Enrofloxacin
10.0
10.0
10.3 (IV)
4.3
2.44 (oral)
34.51
64.0
HPLC
Anadón et al., 1995
Enrofloxacin
10 (PO)
NR
14
Nd
1.5
35
Nd
HPLC
Da Silva et al., 2006
Camels
Enrofloxacin
2.5
2.5 IM, SC
3.58
1.4
1.44 (IM)
18.95
85.0 (IM)
Bioassay
Gavrielli et al., 1995
Harbor Seals
Marbofloxacin
5
5
4.96
1.29
3.44
30.91
Nd
HPLC
KuKanich et al., 2007
Invertebrates
Enrofloxacin Cuttlefish
10
10
1.0
Nd
10.95
26.71
Nd
HPLC
Gore et al., 2005
Sea Urchin
10 inject
10 inject or 10 mg/L water bath
38.8
0.45
90.92
1,200
Na
HPLC
Phillips et al., 2016
Sea Stars
5 inject
5 inject or 5 mg/L water bath
42.76
0.713
18.9
345.85
Na
HPLC
Rosenberg et al., 2016
Pigs
Enrofloxacin
2.5
345
3.34
1.17
5.97
150
HPLC
Zeng and Fung, 1997
Enrofloxacin
2.5 IM
2.5 IM
12.06
1.85
1.17
19.18
74.53
HPLC
Anadón et al., 1999
Enrofloxacin
2.5 IV
Nd
9.64
1.41
Na
25.69
Na
HPLC
Anadón et al., 1999
Enrofloxacin
2.5 IM
2.5 IM
9.3
3.22
0.695
8.903
Na
HPLC
Bimazubute et al., 2010
Enrofloxacin
2.5
7.73
3.5
0.61 (IM)
9.94
95.0 (IM)
HPLC
Richez et al., 1997a
Enrofloxacin
2.5
5.5
3.95
0.75 (IM)
5.03
101 (IM)
Bioassay
Pijpers et al., 1997
Enrofloxacin
10.0 oral
nd
nd
nd
27.0
73.0–80.0
HPLC
Gyrd-Hansen and Nielsen, 1994
Enrofloxacin
7.5 SC
7.5 SC
26.6
6.4
1.10
47.86
Na
HPLC
Messenger et al., 2012
Ciprofloxacin
3.06
nd
2.57
3.83
0.17
2.88
37.0
HPLC
Nouws et al., 1988
Danofloxacin
5
Nd
8.0
ND
0.8
6.0
76 (IM)
HPLC
Mann and Frame, 1992
Marbofloxacin (12 weeks)
8 IV
8 IM or IV
13.5
Nd
Nd
88.6
Na
HPLC
Schneider et al., 2014
Marbofloxacin (12 weeks)
8 IM
8 IM or IV
13.2
1.58
5.55
79.9
89.6
HPLC
Schneider et al., 2014
Marbofloxacin (16 weeks)
8 IV
8 IM or IV
18
Nd
Nd
102
Na
HPLC
Schneider et al., 2014
Marbofloxacin (16 weeks)
8 IM
8 IM or IV
16.7
1.75
5.86
106
105
HPLC
Schneider et al., 2014
Marbofloxacin (27 weeks)
8 IV
8 IM or IV
18.4
1.58
Nd
127
Nd
HPLC
Schneider et al., 2014
Marbofloxacin (27 weeks)
4 IM
8 IM or IV
15.4
Nd
3.38
56.9
90.0
HPLC
Schneider et al., 2014
Marbofloxacin (27 weeks)
8 IM
8 IM or IV
15.1
Nd
6.3
115
91.5
HPLC
Schneider et al., 2014
Marbofloxacin (27 weeks)
16 IM
8 IM or IV
15.2
Nd
15.5
228
90.1
HPLC
Schneider et al., 2014
Fish
Enrofloxacin trout
5.0 and 10.0
5.0 mg/kg q24h
24.0 and 30.0
3.22 and 2.56
0.945 and 1.28
109.2 and 171.3
42 and 49
Bioassay
Bowser et al., 1992
Enrofloxacin red pacu
5.0
29.0
nd
46.3 (IM)
57.0 (relative)
HPLC
Lewbart et al., 1997
Enrofloxacin Atlantic salmon
10.0
131.0
22.4
84.3 1.3 (IP)
Bioassay 66 (IM)
Stoffregen et al., 1997
Enrofloxacin Korean Catfish
10 IV
10
17.4
3.93
Na
60.34
Na
LC/MS
Kim et al., 2006
Enrofloxacin Korean Catfish
10 oral
10 oral
34.13
Na
1.02
38.43
64.6
LC/MS
Kim et al., 2006
Turtles
Enrofloxacin Yellow-bellied sliders
5 IM
5 IM
17.6
Na
6.28
75
Na
HPLC
James et al., 2003
Enrofloxacin Yellow-bellied sliders
10 oral
10 oral
32.8
Na
3.44
53.2
93.6
HPLC
James et al., 2003
Enrofloxacin, Red-eared sliders
10 IP
10 IP
47.6
2.5
10.36
308.8
Na
HPLC
Giorgi et al., 2013
Enrofloxacin Loggerhead sea turtles
10 oral
Na
37.8
2.17
4.1
261
Na
Bioassay
Jacobson et al., 2005
Enrofloxacin Loggerhead sea turtles
20 oral
20 (once per week)
54.4
0.93
21.3
1799
Na
Bioassay
Jacobson et al., 2005
Frogs
Enrofloxacin African Clawed Frog
10 IM
10 IM
5.32
0.84
10.85
57.59
Na
HPLC
Howard et al., 2010
Enrofloxacin African Clawed Frog
10 SC
10 SC
4.08
0.92
9.79
47.42
Na
HPLC
Howard et al., 2010
Animal
Enrofloxacin
Ciprofloxacin
Marbofloxacin
Orbifloxacin
Pradofloxacin
Camel
17–24
Cattle
56, 36–45, 60, 46
31, 70, 19, 49.6, 33.8
Sheep
69
Horse
22
37
21 (at 1 µg/ml)
Pig
27
23.6, 35
Dog
15–25, 27, 35, 72
44, 18.5
9.1, 22
13.24 (at 1 µg/ml)
30–35
28.8–31
Rabbit
53, 50, 35, 6.0
33, 28
Chicken
21
30
Mouse
42
Rat
50
Oral Absorption
Stay updated, free articles. Join our Telegram channel

Full access? Get Clinical Tree
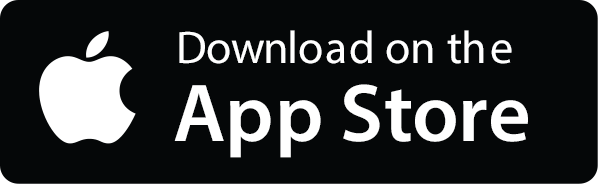
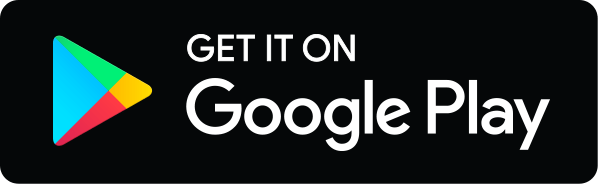