I. BIOCHEMICAL BASIS OF FLUID THERAPY




When this ratio is disturbed, the result is either acidosis or alkalosis.






FIGURE 18-1. The compensatory mechanisms for acid–base disturbances involving intra- and extracellular H+, Na+, and K+ exchanges. As a result of these exchanges, acidosis and alkalosis can lead to hyperkalemia, and hypokalemia, respectively. By the same token, hyperkalemia and hypokalemia can lead to acidosis and alkalosis, respectively.

FIGURE 18-2. The compensatory mechanisms for simple acid–base disturbances as explained by the changes in the Henderson–Hasselbalch equation.

Since is the major buffering system in the body, respiratory and renal control of the blood CO2 and
concentrations intends to keep body pH normal. Under normal physiological condition, the ratio of blood [
] and [H2CO3] is 20:1, where
is the metabolic component and H2CO3 (or dissolved CO2) is the respiratory component. This ratio will change by addition or loss of CO2 and
to the system. Figure 18-2 depicts changes in the ratio of [
] and [H2CO3] that might occur during simple acid–base disturbances.
During hypoventilation (respiratory acidosis), retention of CO2 will lower the ratio. In order to return the ratio to 20:1, the body must retain more through metabolic compensation.
During metabolic acidosis, loss of will decrease the ratio. In order to return the ratio to 20:1, body must expel more CO2 to lower the ratio through respiratory compensation.
These changes in also account for the compensatory processes during respiratory alkalosis (hyperventilation) and metabolic alkalosis (Figure 18-2).


II. GENERAL CONCEPTS OF FLUID AND ELECTROLYTE THERAPY
The clinical signs for detection of dehydration include: loss of skin elasticity, dry buccal mucosa and tongue, and sunken eyeballs should be taken into account.
FIGURE 18-3. Daily water, calorie, and electrolyte requirements for dogs and cats. (Reprinted with permission from Fluid, Electrolyte, and Acid–Base Disorders, 3rd ed. Edited by DiBartola S. P. Saunders/Elsevier, 2006, Figure 14-1. This figure was modified from Harrison J. B., Sussman H. H., and Pickering D. E. Fluid and electrolyte therapy in small animals. JAVMA 137:637–645, 1960, Figure 1.)

Replacement volume (L) = %dehydration × body weight (kg)

TABLE 18-1. Composition of Selected Fluid Therapy Solutions



FIGURE 18-4. The metabolism of lactate into bicarbonate by the Krebs cycle.

H2CO3 + NaCl → NaHCO3 + HCl
HCl will then be absorbed from the small intestine. GI stasis will prevent/delay the absorption of HCl into the circulation, thereby resulting in metabolic alkalosis.
Alkalosis becomes apparent when a large amount of HCl is converted into NH4Cl.
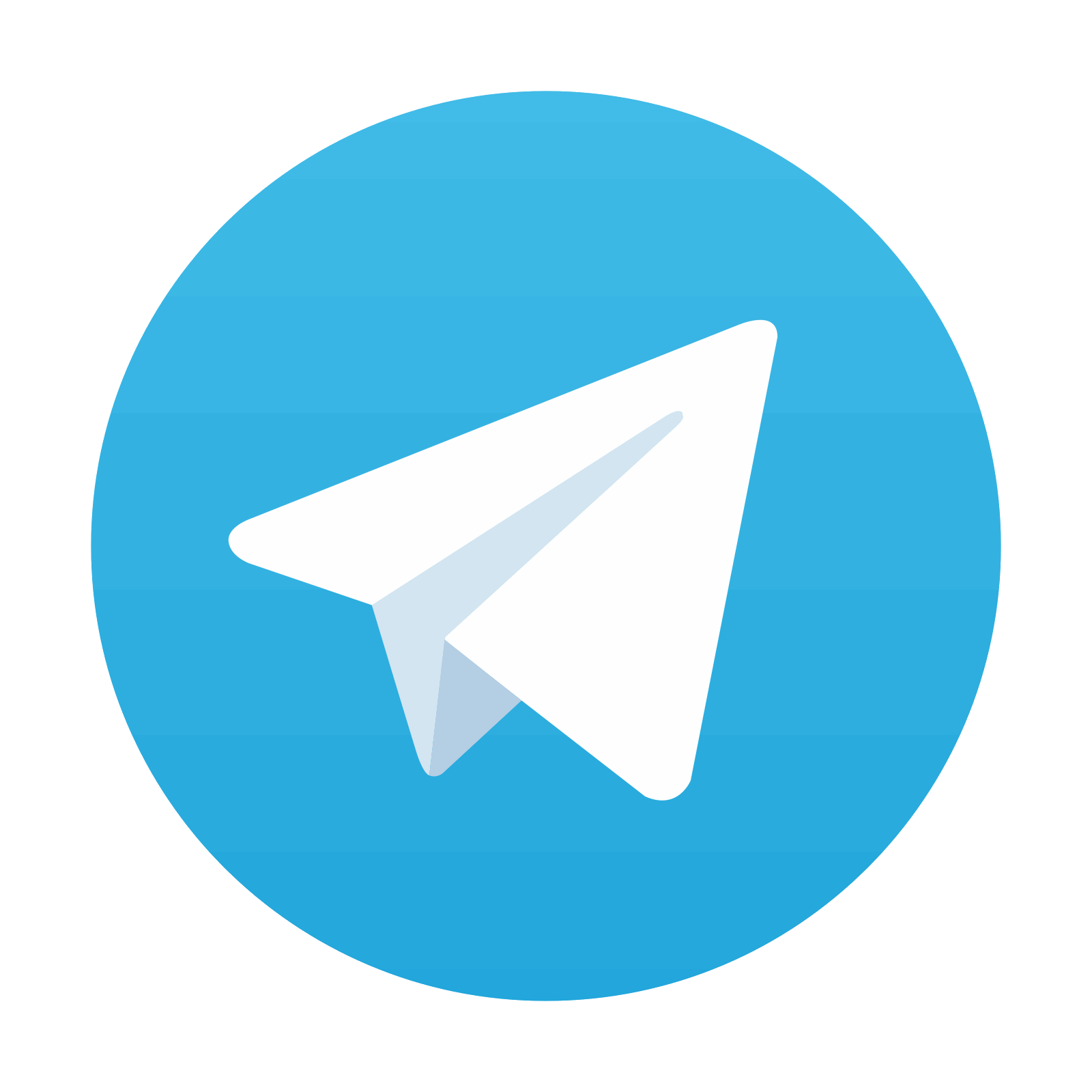
Stay updated, free articles. Join our Telegram channel

Full access? Get Clinical Tree
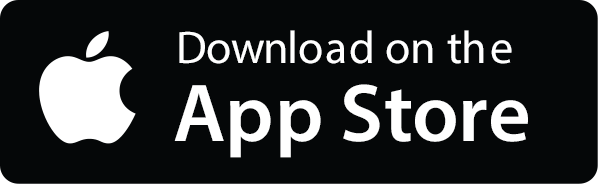
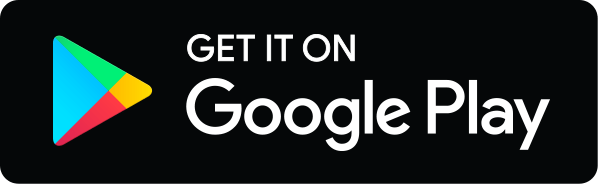
