Agent of disease
Diseases
Type of the agents of disease
Authors
Polio
Poliomyelitis
Viruses
Greenberg (1965)
Shigella sp.
Dysentery
Bacteria
Levine and Levine (1991)
Vibrio cholerae
Cholera
Bacteria
Fotedar (2001)
EHEC O157:H7
Enteritis
Bacteria
Iwasa et al. (1999)
ETEC
Diarrhea
Bacteria
Jordi et al. (2000)
Campylobacter spp.
Diarrhea
Bacteria
Szalanski et al. (2004)
Helicobacter pylori
Enteritis
Bacteria
Grübel et al. (1997)
Salmonella spp.
Salmonellosis
Bacteria
Olsen and Hammack (2000)
Salmonella typhimurium
Typhus
Bacteria
Greenberg (1973)
Mycobacterium sp.
Tuberculosis
Bacteria
Fischer et al. (2001)
Staphylococcus aureus
Enteritis
Bacteria
Chlamydia trachomatis
Trachoma
Bacteria
Emerson et al. (2004)
Aspergillus, Penicillium
Multiorgan invasion
Fungi
Sales et al. (2002)
Cryptosporidium sp.
Diarrhea
Protozoa
Szostakowska et al. (2004)
Giardia lamblia
Giardiasis, diarrhea
Protozoa
Szostakowska et al. (2004)
Ancylostoma duodenale
Hookworm disease
Nematodes
Oyerinde (1976)
Table 9.2
Reports on fly-transmitted agents of animal diseases in the literature (selection)
Agent of disease | Diseases | Type of the agent of disease | Host | Authors |
---|---|---|---|---|
H5N1 and others | Bird flu | Virus | Birds/humans | |
Newcastle virus | Newcastle disease | Virus | Birds | Bram et al. (2002) |
Herpes virus | Aujeszky’s disease | Virus | Pigs | Medveczky et al. (1988) |
PRRSV | Respiratory syndrome | Virus | Pigs | Otake et al. (2004) |
FMDV | Foot and mouth disease | Virus | Cattle, pigs | Greenberg (1973) |
Bacillus anthracis | Anthrax | Bacteria | Cattle | Greenberg (1973) |
Mycobacterium sp. | Tuberculosis | Bacteria | Cattle, pigs | Fischer et al. (2001) |
Campylobacter jejuni | Diarrhea | Bacteria | Birds | Shane et al. (1985) |
Streptococcus suis | Diarrhea | Bacteria | Pigs | Enright et al. (1987) |
Corynebacterium pseudotuberculosum | Mastitis | Bacteria | Cattle | Yeruham et al. (1996) |
Brucella species | Brucellosis | Bacteria | Cattle, pigs | Greenberg (1973) |
Cryptosporidium species | Diarrhea | Protozoa | Birds, cattle, pigs | Graczyk et al. (1999) |
Poultry tapeworm | Diarrhea | Cestodes | Birds | Abrams (1976) |
Parafilaria species | Skin disease | Nematodes | Cattle | Bech-Nielsen et al. (1982) |
Thelazia species | Eye worm disease | Nematodes | Cattle | O’Hara et al. (1989) |
Thus, to obtain more recent data on the existing threat by microorganisms in industrial regions, the University of Düsseldorf initiated a long-term investigation (2006–2011) into the load of pathogens on flies close to stables/meadows used by chickens, pigs, cattle, horses, rabbits, and dogs and in addition checked the refinding rates after experimental exposure of flies to such agents of diseases (Förster et al. 2007, 2009; Förster 2009). In 2009, a Safeguard project (Campylobacter) was started by the governments of the Netherlands and North Rhine-Westphalia, which is the German state bordering the Netherlands. The aim of this project was to check the flies at selected downtown sites (with a huge number of restaurants) and recreation sites with respect to their load of agents of diseases in order to obtain basic data which may help to establish methods and better prophylaxis measures to interrupt transmission of agents of diseases. This chapter gives some of the results obtained with respect to bacteria and fungi which occurred on the surface of flies and/or in their intestine. Chapter 10 by Förster et al. (2012) deals with the transmission of different groups of parasites by various species of flies captured in the wild and reports from laboratory experiments showing the capacity of flies to transmit various parasites.
9.2 Methods and Targets
9.2.1 Fly Catching Close to Animal Houses
Flies were caught on warm and dry days (20–30°C) close to a dog meadow, poultry house, cattle barn, horse stable, pigpen, and rabbit cages in the surroundings of the small town of Dormagen near Düsseldorf in Germany. To avoid contamination by the tissues of typical insect catchers, the flies were first caught either with sterile vessels or with commercial sterile plastic bags and were immediately placed for 5–10 min in sterile petri dishes on blood agar. After this incubation, the flies were removed from the petri dishes, killed by CO2, and identified under a dissecting microscope. Later, larger numbers of flies were also caught with freshly washed butterfly nets. In these cases only the intestinal contents of these flies were examined.
9.2.2 Fly Catching at Recreation Sites
The catching sites at four selected recreation sites (often with crowds of people and where there were also numerous birds—geese, swans, ducks, and pigeons) were situated in larger towns or close by them. The following four sites were selected:
1.
The Old Town of Düsseldorf (Germany), with its many restaurants. The catching sites were meadows and bushes in the surroundings.
2.
A park in a suburb of Düsseldorf (at Castle Eller).
3.
A bathing lake close to Düsseldorf, which is crossed by the river Düssel, which gives its name to Düsseldorf. In summer thousands of people bathe there.
4.
The six-lakes-region close to the town of Duisburg (Germany), where the lakes are visited by huge numbers of people.
The flies were caught at intervals of 7–14 days (depending on the weather) with the help of butterfly nets, the opening of which had a diameter of 25 cm. The flies were transferred singly into sterile plastic vessels immediately after the catch.
The feces of the birds was collected in sterile, one-way plastic tubes and transported to the institutes for microbiological and/or parasitological investigations.
9.2.3 Determination of Microorganisms
The blood agar plates onto which the freshly caught flies had been placed were incubated at 37°C for 24 h. After the incubation, the petri dishes were checked for growth of microorganisms. For identification, the microorganisms were isolated and subcultured on blood agar and selective agar plates, and were finally incubated at 37°C for another 24 h. For identification of fungi, the microorganisms were isolated and were subcultured on Saboraud’s dextrose agar, and were then incubated at room temperature for 4 days. After the incubation period, the bacterial colonies were identified by standard bacteriological procedures (e.g., coagulase, oxidase test, Gram’s reaction, API®, Prolex®, VITEK®, etc.). Isolated E. coli bacteria were identified for their pathogenicity by in-house real-time polymerase chain reaction, differentiating enterotoxic E. coli, enteropathogenic E. coli (EPEC), EHEC, and enteroaggregative E. coli.
9.3 The Flies
9.3.1 Flies Around the Stables
The flies that were found close to the stables differed considerably with respect to their species relations from those found around dog meadows (Figs. 9.1–9.5). These differences are surely based on their different needs for different suitable breeding sites. Tables 9.3 and 9.4 summarize the occurrence of the different fly species at the different catching sites.
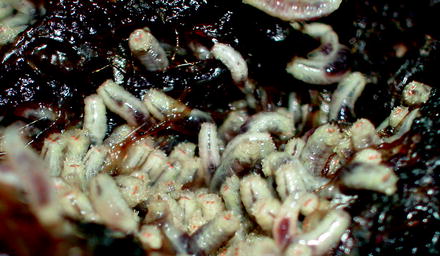
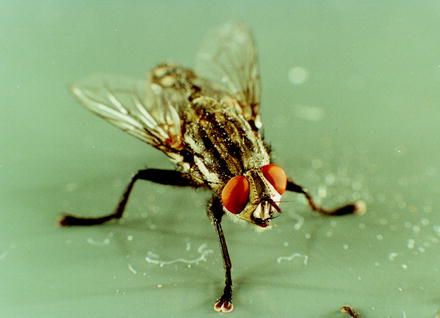
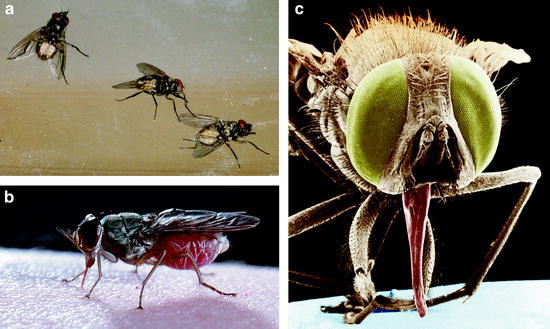
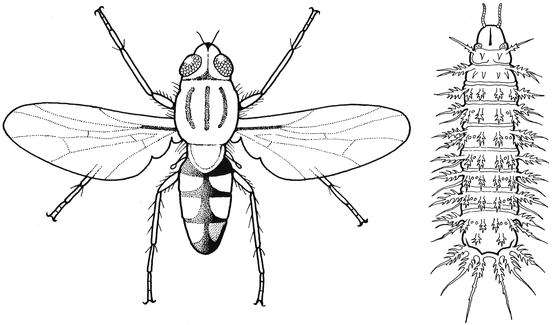
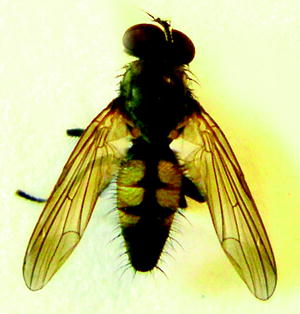
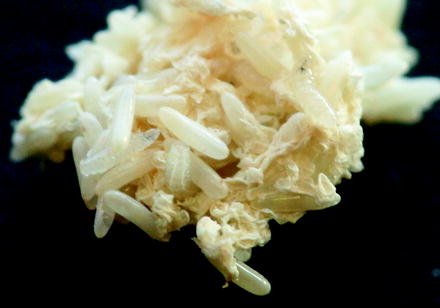
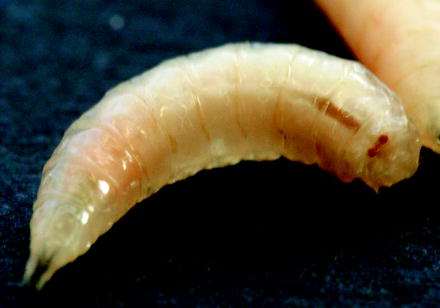
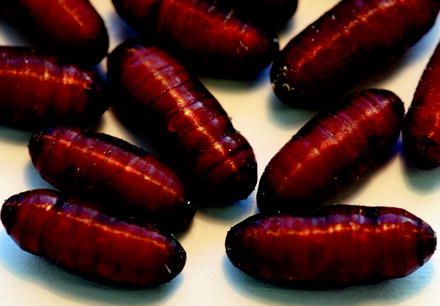
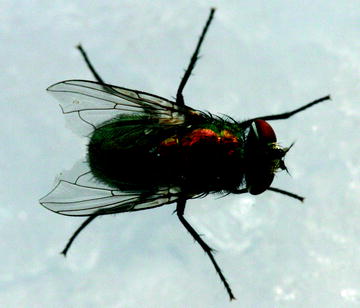
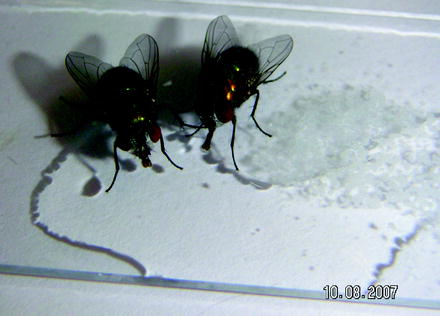
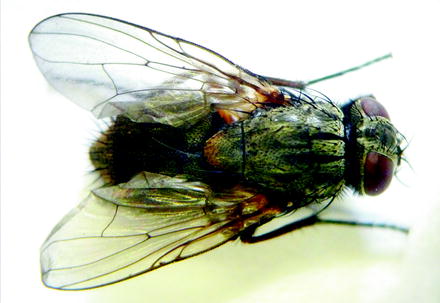
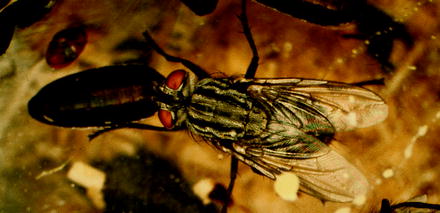
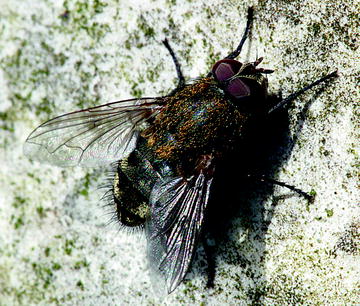
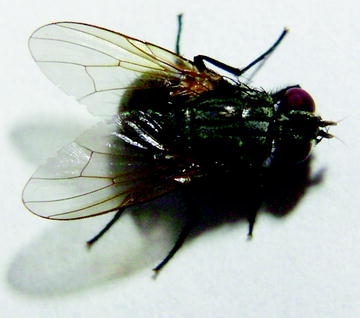
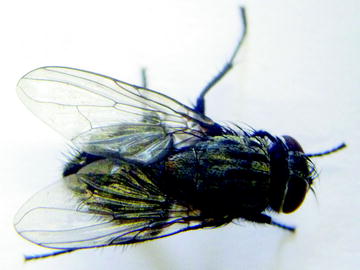
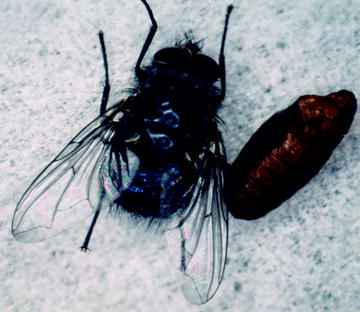
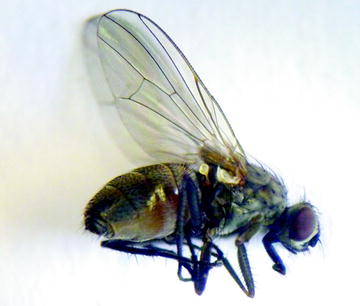
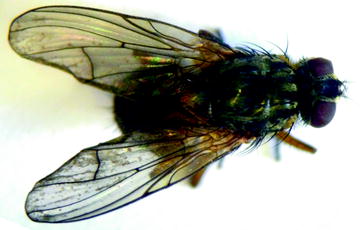
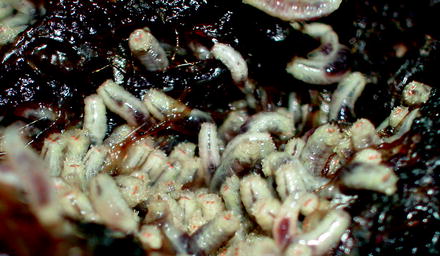
Fig. 9.1
Motile Musca domestica larvae on their meat substrate
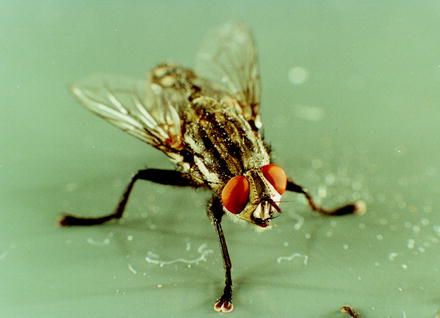
Fig. 9.2
Adult Musca domestica fly
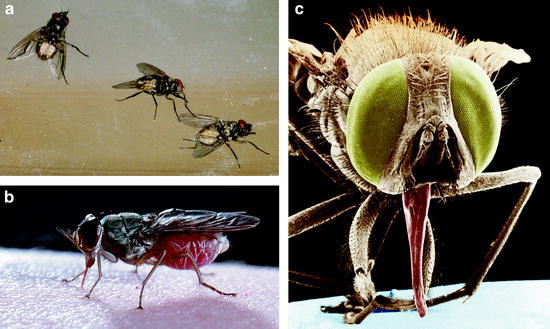
Fig. 9.3
Stomoxys calcitrans. (a, b) Macrophotographs of adults. (c) Scanning electron micrograph of the head with the piercing/sucking apparatus
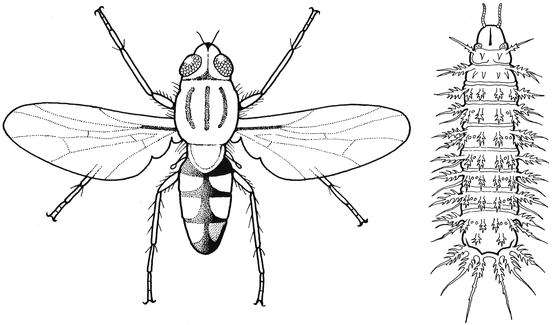
Fig. 9.4
Fannia canicularis, adult and larva
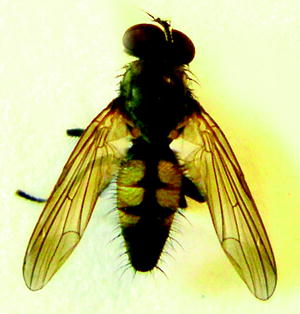
Fig. 9.5
Fannia canicularis, adult stage
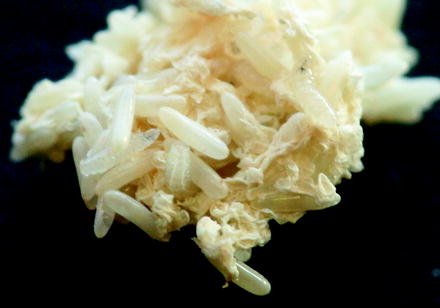
Fig. 9.6
Lucilia sericata, eggs
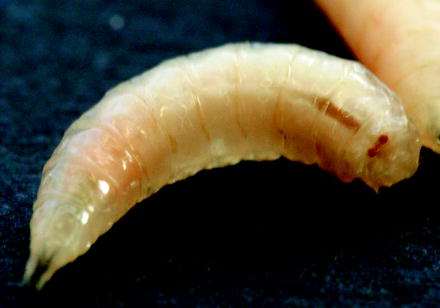
Fig. 9.7
Lucilia sericata, larva
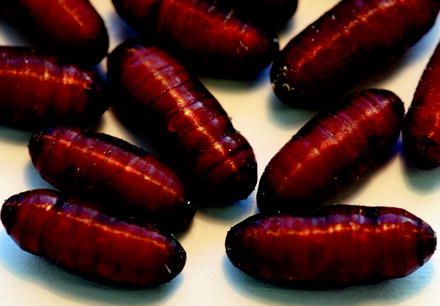
Fig. 9.8
Lucilia sericata, pupae
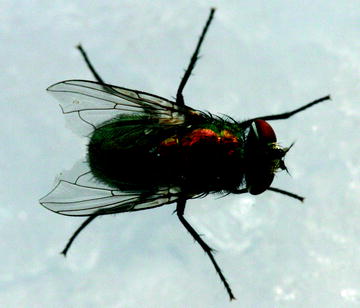
Fig. 9.9
Lucilia sericata, adult female
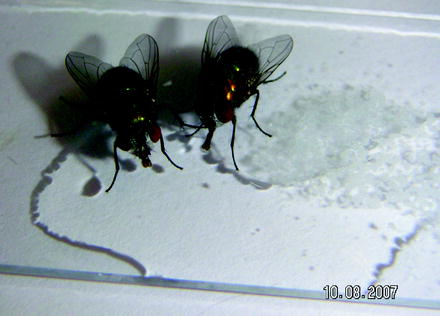
Fig. 9.10
Lucilia caesar, adult female and male
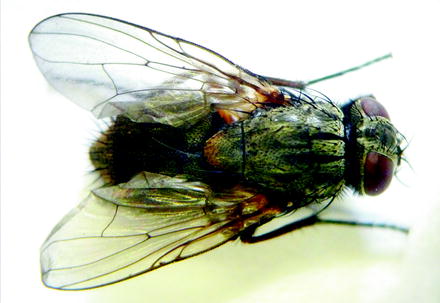
Fig. 9.11
Muscina stabulans, adult female
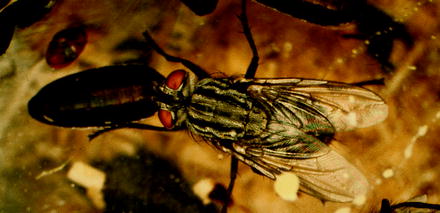
Fig. 9.12
Sarcophaga carnaria, adult and pupa
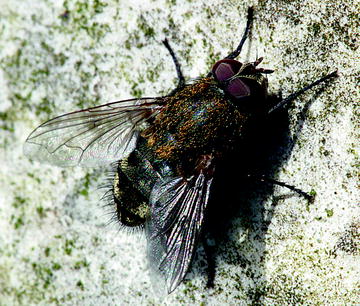
Fig. 9.13
Pollenia rudis. These flies (6–12 mm) have square silver dots on their abdomen. They enter houses in autumn and winter
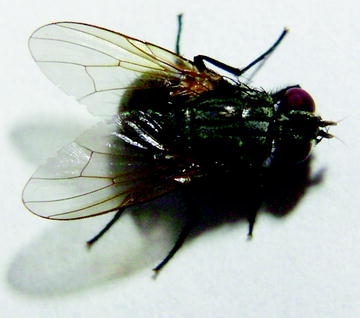
Fig. 9.14
Mydaea ancilla, adult female. This species belongs to the family Muscidae
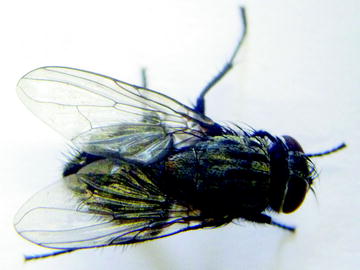
Fig. 9.15
Muscina pabulorum, adult female
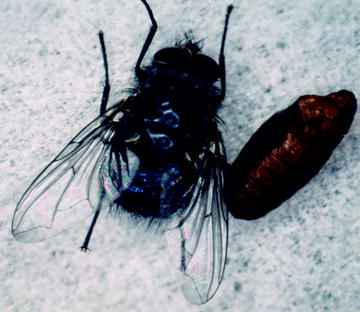
Fig. 9.16
Calliphora vomitoria, adult stage and pupa
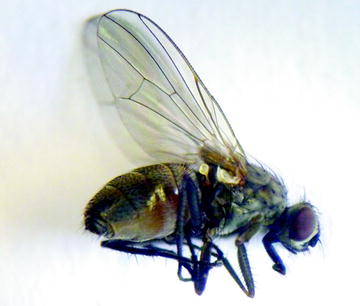
Fig. 9.17
Helina reversio, the head of which has a frontal black line. The yellow-gray abdomen is characterized by two black dots
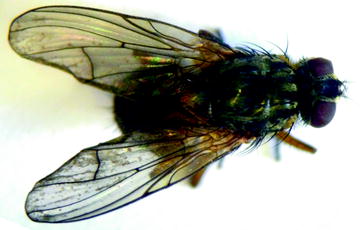
Fig. 9.18
Phaonia errans. This 9–12-mm-sized fly has completely yellow legs and the tip of the abdomen of the male appears reddish-yellow
Table 9.3
Main fly species close to stables (most of them are also known for their ability to introduce myiasis in humans)
Stable | Fly species |
---|---|
Cattle barns | |
Horse stables | Musca domestica, Stomoxys calcitrans |
Poultry houses | Mainly Musca domestica |
Pigpens | |
Rabbit cages | Mainly Musca domestica, Stomoxys calcitrans |
Table 9.4
Fly species on a meadow where dogs regularly deposit their feces (dog pond)
Fly species | Percentage of total catches |
---|---|
54% | |
Muscina stabulans (Fig. 9.11) | 15% |
Sarcophaga carnaria (Fig. 9.12) | 11% |
Pollenia rudis (Fig. 9.13) | 4% |
Mydaea urbana | 4% |
Mydaea ancilla (Fig. 9.14) | 2% |
Muscina pabulorum (Fig. 9.15) | 2% |
Calliphora vomitoria (Fig.9.16) | 2% |
2% | |
Each species appeared as 1% of the catches |
Around the stables, Musca domestica was the absolutely dominant species. In all catches in 3 years it was always present in the same relations:
44% of the catches in the pigpens
30% of the catches in horse stables
17% of the catches in rabbit cages
9% of the catches in cattle barns
In several cases its occurrence in poultry houses reached approximately 90%, which coincided with several important and massive fly outbreaks which were investigated by our group:
(a)
In a valley with large poultry houses and chicken feces stored in the open air
(b)
After several field fertilizations with chicken feces close to villages
(c)
In a small town with a compost plant, the units of which were opened nightly by feral pigs and in the early mornings by crows
In all these cases the private houses up to a distance of 1 km were filled with hundreds of Musca domestica adults, which were apparently attracted by the dark interior of the houses when arriving from the surrounding fields, from the compost plant, and/or from badly stored chicken feces.
Practically in all cases the sex ratio of the flies caught was nearly 50:50 independent of whether the flies were caught close to the stables (i.e., outdoors) or inside houses.
The bloodsucking species Stomoxys calcitrans was an exception: only 42% of the flies caught outside were females. This might be attributable to the behavior of the females to suck blood and then to lay their eggs inside stables.
Tables 9.5 and 9.6 provide information on the life cycles of several important fly species, which of course primarily attack farm animals, but, however, also molest humans by often numerous touchdowns. During these contacts, agents of diseases (see Tables 9.1 and 9.2) may be placed on humans and their food too. In these cases the flies could become vectors of zoonotic agents of diseases (see also Sect. 9.5 and Chap. 10).
Table 9.5
Development data of flies of central Europe
Species | Size of adult (mm) | Eggs | Hatching of larvae | Larval development | Pupal rest | Life span of adults |
---|---|---|---|---|---|---|
Musca domestica | 6–7 | 600–1,000 on feces (chicken, pig, horses, cattle) and other decaying organic matter | 15°C: 50 h | 15°C: 10–26 days | 15°C: 18–21 days | 60–70 days in stables |
20°C: 23 h | 20°C: 8–10 days | 20°C: 10–11 days | ||||
30°C: 10 h | 30°C: 4–7 days | 30°C: 4–5 days | ||||
Musca autumnalis | 5–7 | 600–900 on fresh feces | Temperature-dependent | 4–7 generations per year | 4–7 generations per year | Females of last generation hibernate |
Fannia canicularis | 4–6 | Feces and putrescent material | 25°C: 20–48 h | 6 days | 7–10 days | 6–7 generations per year |
Muscina stabulans | 6–8 | Eggs, larvae on chicken feces | Life cycle in summer about 2–3 weeks | Life cycle in summer about 2–3 weeks | Life cycle in summer about 2–3 weeks | 4–5 generations per year |
Stomoxys calcitrans | 6–7 | 800 in groups of 25–50 in silage, in stables with urine and feces | 1–2 days, temperature-dependent: 14 days up to months | 6–8 days, temperature-dependent: 14 days up to months | 6–8 days, temperature-dependent: 14 days up to months | Females live about 70–90 days |
Haematobia irritans | 4.5–5 | In fresh cattle dung | Temperature-dependent: 24 days up to months, optimum 27–30°C | Temperature-dependent: 24 days up to months, optimum 27–30°C | Temperature-dependent: 24 days up to months, optimum 27–30°C | 3–4 generations per year |
Calliphora species | 9–11 | Eggs on feces with cadavers | Temperature-dependent | Temperature-dependent | 10–40 days | 1–2 months |
10–14 | ||||||
Sarcophaga carnaria | 10–19 | Eggs on cadavers, agent of myiasis | Temperature-dependent | Temperature-dependent | 10–40 days | 1–2 months |
Lucilia sericata, L. caesar | 5–11 | Eggs on feces, wounds, meat | 24 h | 4–7 days | 1–2 weeks on the ground | 1–2 months |
Pollenia rudis | 6–12 | Eggs on feces, soil | Larvae parasitize in earthworms | 4–8 days | 1–2 weeks on carcasses | 1–2 months |
Phaonia errans | 9–12 | Eggs are placed on wood powder or dry feces | Temperature-dependent | 4–5 generations per year | 10–40 days | 1–2 months |
Hydrotaea dentipes | 6–7 | Eggs on human and animal feces | Temperature dependent | 4–6 generations per year | 10–40 days | 2–3 months |
Oestrus ovis | 8–15 | Larvae are deposited at the nose or eyes | Immediately after laying | Larvae hibernate in the nose | 2–4 weeks on the ground | 4 weeks |
Hypoderma bovis | 13–15 | 600–800 glued on hair of cattle | 4–7 days, then invading the skin | Inside the body until March | 14–65 days on the ground | 3–5 days (up to 28 days in the cold) |
Hypoderma lineatum | 11–13 | 5–20 eggs per hair, in a row | 3–6 days, then invading the skin | Inside the body until March | 23–28 days on the ground | 3–5 days |
Table 9.6
Periods of activity of selected flies that attack cattle and sheep in central Europe
Species | Breeding site | Jan | Feb | Mar | Apr | May | Jun | Jul | Aug | Sep | Oct | Nov | Dec |
---|---|---|---|---|---|---|---|---|---|---|---|---|---|
Musca domestica (housefly, typhoid fly) | Eggs and larva in and on animal feces, even in stables | +1 | +1 | +1 | +1 | + | + | + | + | + | + | +1 | +1 |
Musca autumnalis (eye fly) | Eggs and larvae on animal feces outdoors, adults hibernate in buildings | 02 | 02 | 02 | + | + | + | + | + | + | + | 02 | 02 |
Fannia canicularis (little housefly) | Eggs on feces of chicken and other animals, kitchen slops | 02 | 02 | 02 | 02 | + | + | + | + | + | + | 02 | 02 |
Muscina stabulans (false stable fly) | Eggs, larvae often on chicken feces, predacious as well | +1 | +1 | +1 | +1 | + | + | + | + | + | + | +1 | +1 |
Stomoxys calcitrans (stable fly; sucks blood every 3 days) | Oviposition on plant material in stables | + | + | + | + | + | + | + | + | + | + | + | + |
Haematobia irritans (horn fly) | Eggs and larvae on fresh cow dung | − | − | − | − | + | + | + | + | + | − | − | − |
Calliphora vicina, C. vomitoria (blowflies) | Eggs on feces and cadavers | − | − | + | + | + | + | + | + | + | + | + | − |
Sarcophaga carnaria (flesh fly) | Eggs and larvae on meat, cadavers, earthworms | − | − | − | + | + | + | + | + | + | + | − | − |
Lucilia sericata (gold fly) | Eggs in wounds, cadavers, feces | − | − | − | − | + | + | + | + | + | + | − | − |
Lucilia caesar (carrion fly) | Eggs and larvae in feces, cadavers | − | − | − | − | + | + | + | + | + | + | − | − |
Oestrus ovis (nose botfly) | Larvae are extruded into the nose of sheep and goats | Larvae in nose | Larvae in nose | Larvae in nose | Pupae on ground | + | + | + | + | + | + | + | Larvae in nose |
Hypoderma bovis (large skin botfly) | 600–800 eggs adhere to hair of cattle | Larvae in body | Larvae in body | Larvae in body | Pupae on ground | + | + | + | + | + | Larvae in body | Larvae in body | Larvae in body |
Hypoderma lineatum (small botfly) | 5–20 eggs per hair of cattle | Larvae in body | Larvae in body | Larvae in body | Larvae in body | + | + | + | Larvae in body | Larvae in body | Larvae in body | Larvae in body | Larvae in body |
Tables 9.5 and 9.6 in addition show the periods when the flies are most active at the in general moderate temperatures of central Europe. In warmer regions they are active for practically the whole year, so the potential transmission of agents of diseases may occur throughout the year. This makes it clear that only strict prophylaxis measures will protect animals and as a consequence also humans who live in the close neighborhood of animals.
All flies caught in the surroundings of human dwellings and/or different animal houses or recreation sites are so-called synanthropic species (Sukopp and Wittig 1998). The definition of the term “synanthropy” characterizes plant or animal species which have adapted their life cycle to human housing areas. There they may reproduce in such high numbers that they do not need additions from outside to maintain their population at a constant level. In cases of an increase in food, many other individuals of this species might be attracted and this may lead to real plagues. Eusynanthropy and hemisynanthropy are subgroups of synanthropy. In the first case, the occurrence and reproduction of a certain species is obligatory because of the co-occurrence with human houses. Members of the genus Drosophila are examples of flies in Europe. Examples of other insects are the bedbug (Cimex lectularius), the bread beetle (Stegobium paniceum), and imported cockroaches (Blatta orientalis, Blattella germanica, Supella longipalpa, Periplaneta americana), which can only exist in human houses, whereas forest cockroaches (e.g., Ectobius silvestris) enter human dwellings only occasionally. “Hemisynanthropy” (the facultative form) covers species which find an optimum amount of food and facilities for survival inside or around human dwellings, but these species also survive easily outside human settlements. For example, Lucilia caesar is such an species as is the Eurasian swift (Apus apus), which likes to use human buildings as a starting point for its magnificent flights, although it may also start from natural rocks, etc.
With respect to these definitions of synanthropy, many flies are hemisynanthropic animals, which use the facilities of human settlements with all their garbage, superfluous food, and protected places for their—often immense—reproduction. They may, however, survive easily far away from human settlements, since dead animals, their carcasses, and their degrading feces offer practically unlimited food and propagation facilities. Therefore, even the best fly control measures cannot prevent the constant influx of flies from outside into human houses and into the stables of farmed animals. However, eusynanthropy and hemisynanthropy of most flies make it necessary for humans to control fly propagation by minimizing the breeding sites in and around human buildings of any kind. This can be done simply by reduction of garbage, removing remnant food, removing feces, changing straw, etc. In addition—especially in monocultures of many animals—chemical treatment of animals, of the stable walls, and/or of the straw might become necessary to prevent feeding farm animals from being disturbed or even to avoid infections with agents of diseases as listed in Tables 9.1 and 9.2.
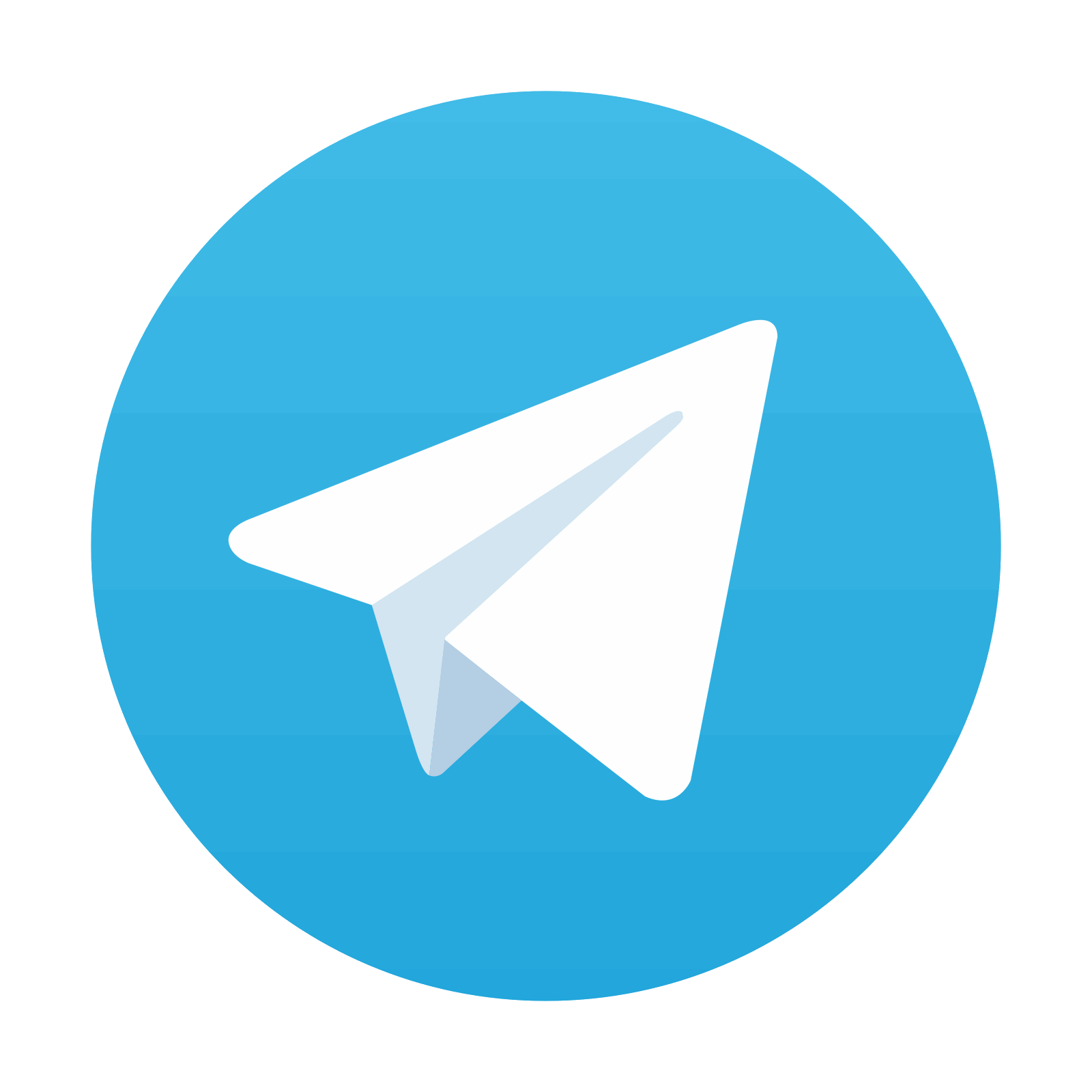
Stay updated, free articles. Join our Telegram channel

Full access? Get Clinical Tree
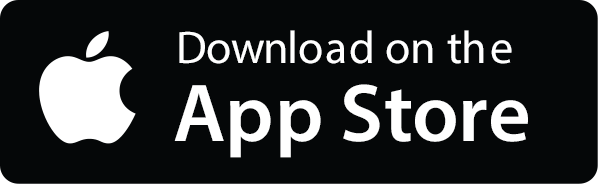
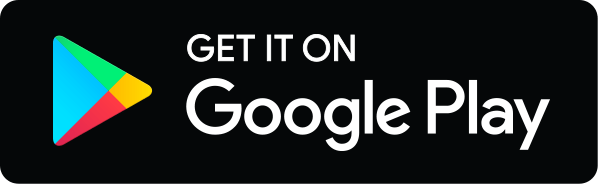