Fig. 33.1
Electron micrographs of molecular layers (a, b) or Purkinje cell layers (c) in mouse cerebellar cortex prepared by the “in vivo cryotechnique ” without ischemia (IVCT; a, c) or quick-freezing of fresh tissues (FQF; b) show that a number of open extracellular space s (a, c; arrowheads) are seen among the parallel fibers (a; inset, asterisks) and dendrites (a, De) or cell bodies (c, Pu) of Purkinje cells . In the cerebellar specimens prepared with FQF (b), few open extracellular spaces are observed among the parallel fibers (b, asterisks). Bars, 500 nm in (a) and (b); 1 μm in (c). (The figure is adapted from Ohno et al. [6])
Electron microscopic images of cerebellar cortex obtained by quick-freezing of fresh resected tissues followed by freeze-substitution (FQF) reflect the effect of anoxia for a few minutes, because the tissue specimens of the cerebellum had to be resected from the anesthetized mice before the cryofixation . Few open ECS were detected in the cerebellar tissues prepared with FQF, although in mouse cerebellum prepared with the IVCT, widely open ECS could be observed among cellular profiles in the molecular layers (Fig. 33.1) [6]. The open ECS were also maintained around processes and soma of Purkinje cell s in Purkinje cell layers (Fig. 33.1). Ischemia for 30 s still remains the open ECS, whereas ischemia for 8 min eliminated the open ECS [6]. IVCT without ischemia also suggested that the size of ECS could vary depending on the area in the molecular layers, since the ECS sizes among cellular profiles ranged from as large as a few hundred to <10 nm2 and the percent area occupied by ECS ranged from 8 to 28 % [6]. Previous studies indicated that ECS occupies about 10–20 % of CNS tissues, although direct observation by electron microscopy has been difficult. The difficulty is attributable to technical artifact s caused during conventional chemical fixation or asphyxia of tissue resection prior to resected tissues followed by quick-freezing [2, 8]. The asphyxia before completion of chemical fixation can cause the morphological changes through movement of extracellular fluid and electrolytes into intracellular compartments [9], although even larger molecules may easily enter the intracellular compartment via gap junction hemichannels during ischemia/anoxia [10]. When the cellular processes in molecular layers of mouse cerebellum were examined in tissue specimens prepared with IVCT or FQF, not only several minutes of anoxia but also even 30 s of ischemia can increase the size of parallel fibers [6]. The rapid changes in the size of Bergmann glia l fibers as well as neuropils support the rapid translocation of various extracellular components. IVCT would provide novel information about ultrastructural variance within molecular layers of the cerebellar cortex which was indicated in previous studies using conventional chemical fixation and dehydration methods [11–13].
ECS occupied considerable areas in molecular and Purkinje cell layers of the mouse cerebellar cortex , but the size of ECS appeared variable [6]. The ECS and their heterogenous distribution were also proposed in other ultrastructural studies using conventional quick-freezing and freeze-substitution or diffusion analyses [1, 7, 14]. The findings are also compatible with the simulation analyses of in vivo molecular diffusion, indicating that abundant extracellular matrices affect the probe’s diffusion, and ECS of rat cerebral cortex was similar to fluid-filled pores sized about 38–64 nm [15, 16]. Indeed, abundant meshwork structures in the remaining ECS could be observed in mouse cerebellar tissues with the quick-freezing and deep-etching method after perfusion fixation [6]. Combination of IVCT with the diffusion analysis, by which ECS in living animals can be examined under anesthesia [14], will be one feasible approach for further analyses of ECS. Roles of widely open ECS in living mice may include dynamic remodeling of the cerebellar cortex morphology, such as enlargement of dendritic spine or extension of cellular processes [17, 18].
33.3 Synaptic Clefts, Open ECS, and Surrounding Glial Processes
In specimens prepared with IVCT, many synapses in molecular layers of cerebellar cortex were detected between parallel fibers and Purkinje cell dendrites (Fig. 33.1). About 45 % of the synaptic clefts appeared directly exposed to the open ECS (Fig. 33.2) [6]. The other synaptic clefts were completely or partially surrounded with closely apposed glial sheaths (Fig. 33.2). The open ECS around this type of synapses was observed outside of the glial sheath (Fig. 33.2). When (1) the length between the presynaptic active zones and surrounding cellular profiles in synapses open to ECS and (2) the shortest length between synaptic clefts and nearest cellular profiles other than surrounding glial sheaths in the synapses enclosed by glial processes were measured, the lengths obtained in opened or enclosed synapses were not significantly different [6].
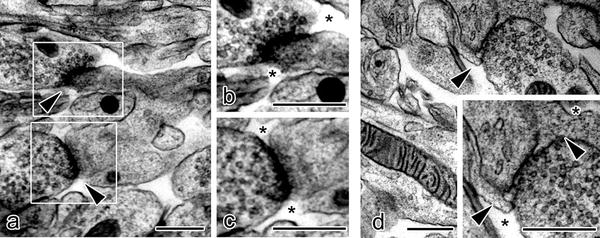
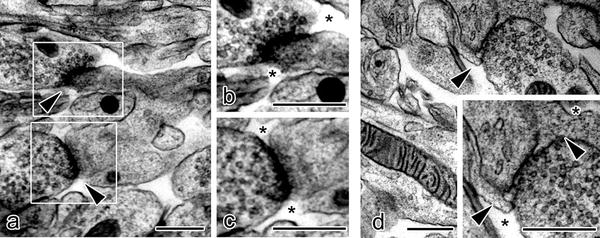
Fig. 33.2
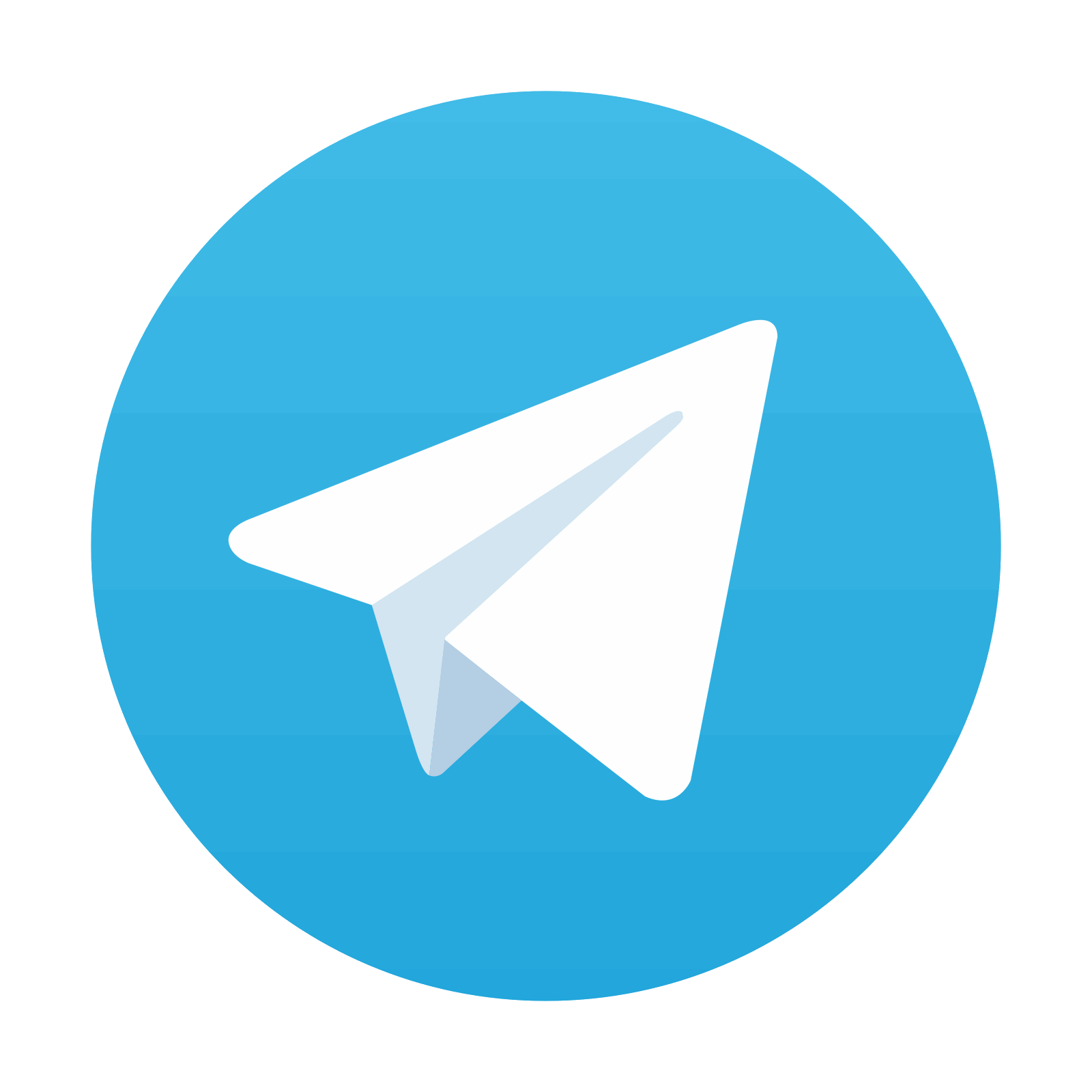
Electron micrographs of synaptic clefts in molecular layers of mouse cerebellar cortex prepared with the “in vivo cryotechnique ” show that some synaptic clefts (a, arrowheads) are exposed to the open ECS (b, c; asterisks), whereas the other synaptic clefts are not exposed and totally or partially enclosed with glial sheaths (d, arrowheads). Open extracellular space s (ECS) are located outside of the glial sheaths (d, asterisks). Bars, 500 nm. (The figure was adapted from Ohno et al. [6])
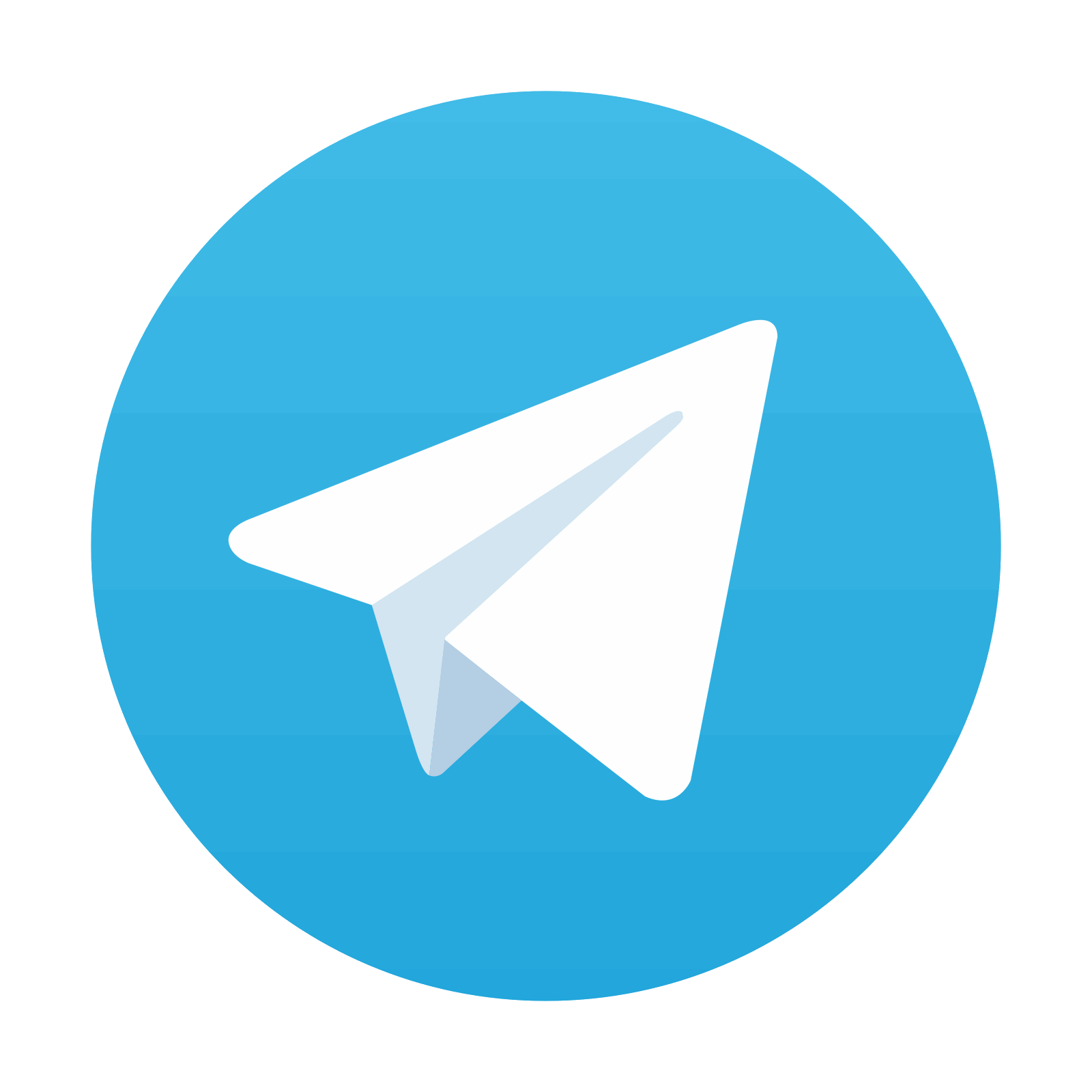
Stay updated, free articles. Join our Telegram channel

Full access? Get Clinical Tree
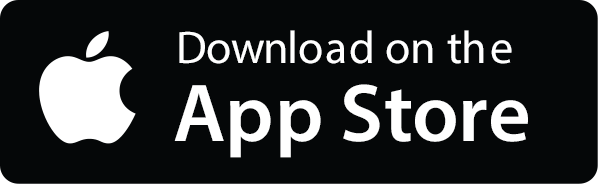
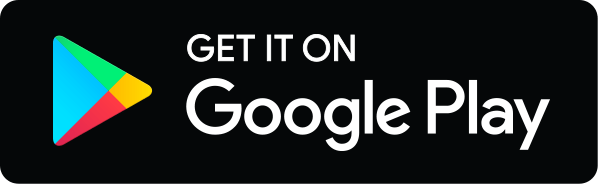
