CHAPTER 28 Evaluation of performance potential requires an understanding of the physiologic mechanisms involved in the energetics of exercise. Muscular work requires that the physiologic systems of the horse are integrated to minimize the stress imposed on the component mechanisms supporting the energetics. Muscular respiration depends on complex interactive systems that allow gas exchange between muscle cells and the atmosphere. Optimal gas exchange between muscle cells and the atmosphere requires (1) efficient lung function, (2) effective pulmonary circulation, which is able to match the requirements of ventilation, (3) blood with an adequate hemoglobin concentration, (4) a cardiovascular system that can deliver an appropriate quantity of oxygenated blood to the periphery to match tissue respiratory requirements, and (5) control mechanisms capable of regulating arterial blood gas tensions and pH. For the oxidative metabolic pathways to be able to meet the energy demands imposed by exercise, delivery of oxygen to the working muscle via the pulmonary and cardiovascular systems must be adequate. Working muscle consumes the O2, and in response to the increased extraction of O2 and addition of CO2 to capillary blood by muscle, an almost immediate increase occurs in muscle blood flow. The initial vasodilation is thought to be centrally induced, with subsequent dilation occurring under the influence of local humoral control. This process is selective, allowing vasodilation in the muscle units with the highest metabolic rates.
Evaluation of performance potential
Athletic performance
Musculoskeletal system
< div class='tao-gold-member'>
Stay updated, free articles. Join our Telegram channel

Full access? Get Clinical Tree
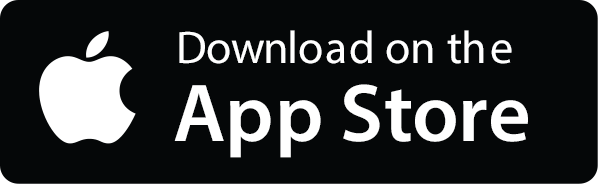
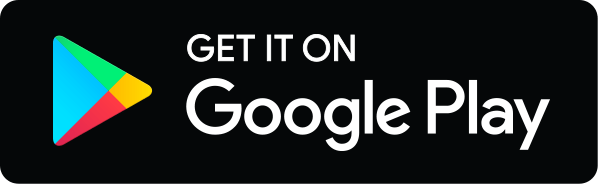