John M. Fairbrother and Carlton L. Gyles Escherichia coli is a Gram‐negative, fermentative, rod‐shaped bacterium, which grows readily on simple bacteriologic media, including MacConkey agar, on which it forms large red colonies. Other features are a positive indole reaction, negative tests for urease and hydrogen sulfide, and failure to use citrate as the sole carbon source. Although vastly outnumbered by anaerobic bacteria, E. coli is the major facultatively anaerobic bacterium in the intestine of most animal species, and is typically present at 107–109 organisms per gram in feces. E. coli is usually the dominant organism recovered on aerobic culture of feces, but pet birds are an exception as E. coli are recovered from only a low percentage of healthy pet birds. The sterile intestinal tract of the newborn animal quickly becomes contaminated with E. coli from the microbiota of the dam and from the environment. E. coli rapidly becomes established in the intestine and remains as a part of the normal microbiota throughout the life of the animal. The concentration of E. coli is low in the upper small intestine but increases progressively, with the maximum concentration in the large intestine. Most E. coli are commensals, but a small proportion of strains are pathogenic, being classified into pathotypes based on the production of broad classes of virulence factors and on the mechanisms by which they cause disease. In animals, the most important pathotypes are intestinal pathogenic E. coli (Table 7.1): enterotoxigenic E. coli (ETEC), enteropathogenic E. coli (EPEC), Shiga toxin‐producing E. coli (STEC); and extraintestinal pathogenic E. coli (ExPEC). Intestinal pathogenic strains are defined as pathotypes by their distinctive virulence profiles, whereas extraintestinal pathogenic strains, although they were traditionally separated into subgroups based on disease association, are now recognized as being more broadly pathogenic at multiple sites in the host, and are grouped together as one pathotype, ExPEC (Dale and Woodford 2015; Vila et al. 2016). More recently, hybrid‐pathogenic E. coli have been described, demonstrating new combinations of virulence factors among these pathotypes (de Mello Santos et al. 2020). Such hybrid pathotypes may lead to the appearance of new clinical signs associated with E. coli disease. In addition, STEC in the normal microbiota of cattle and other ruminants may be highly pathogenic for humans and are designated as enterohemorrhagic E. coli (EHEC). ExPEC and EPEC typically are part of the normal intestinal microbiota of their host and may be considered as opportunistic pathogens. In most E. coli diseases, pathogenicity is associated with virulence genes encoded by plasmids, bacteriophages, or pathogenicity islands (PAI; Desvaux et al. 2020). These genes include plasmid‐encoded genes for enterotoxins and fimbriae or pili, phage‐encoded genes for Shiga toxins (Stx), and PAI‐encoded genes for the attaching and effacing lesion in EPEC and EHEC as well as the pap, hly, and cnf1 genes in ExPEC. Table 7.1 Overview of categories of Escherichia coli found in animals. Differentiation of E. coli into subtypes is important for distinguishing pathogenic from nonpathogenic types and for epidemiological investigations. Serotyping is the most well‐established method and is based on the O and H antigens determined by the polysaccharide portion of lipopolysaccharide (LPS) and flagellar proteins, respectively. Presently, there are 181 O‐antigens (O1 to O187, with O groups 31, 47, 67, 72, 94, and 122 removed); (Liu et al. 2020a) and 53 H antigens (H1 to H56, with 13, 22, and 50 unassigned) in the international typing scheme. For fimbrial antigens are additional surface antigens, whose identification provides valuable information in the characterization of strains. Serotyping remains the foundation of subspecies differentiation. Molecular O and H serotyping using polymerase chain reaction (PCR) or whole‐genome sequencing is now replacing traditional phenotypic methods (Fratamico et al. 2016). Other procedures that are used to characterize isolates include pulsed‐field gel electrophoresis, random amplified polymorphic DNA (RAPD) analysis, amplified fragment length polymorphism, ribotyping, 16S rDNA sequencing, traditional multilocus sequence typing (MLST) based on seven house‐keeping genes, multiple‐locus variable‐number tandem‐repeat analysis (MLVA) and virulence factor analysis (Dale and Woodford 2015; Fratamico et al. 2016). Whole‐genome sequencing typing methods, including core genome MLST and single‐nucleotide polymorphism analysis, are being increasingly used and have a very high discriminatory power. Detection of virulence factors that are unique to or associated with particular types of pathogenic E. coli is important for identification and characterization of pathogenic E. coli into pathotypes (Vila et al. 2016). The availability of more complete virulence gene databases is now allowing a more comprehensive characterization of E. coli isolates based on whole‐genome sequencing data (Tetzschner et al. 2020). E. coli cause a variety of enteric and extraintestinal diseases in animals (Tables 7.2, 7.3). ETEC is the most common cause of E. coli diarrhea in farm animals. This pathotype is characterized by the production of enterotoxins and of adhesins that adhere to the intestinal epithelium and promote colonization (Dubreuil et al. 2016). Enterotoxins produced by ETEC may be heat stable (STa, STb, or enteroaggregative E. coli heat‐stable enterotoxin 1, EAST1), or heat labile (LT). In pigs, the most frequently encountered fimbrial adhesins of ETEC are F4(K88), F5(K99), F6(987P), F41, and F18. Isolates producing the F4 or F18 adhesin and certain isolates producing F6 are hemolytic. In calves and lambs, the most important fimbrial adhesins of ETEC are F5 and F41. Colonies of ETEC that possess these adhesins are usually mucoid and nonhemolytic. F17 fimbriae are associated with bovine or ovine strains that cause diarrhea or septicemia. The virulence genes and O serogroups of ETEC most commonly associated with diarrhea in pigs, cattle, and sheep are shown in Tables 7.4 and 7.5. ETECs cause severe watery diarrhea, which may lead to dehydration, listlessness, metabolic acidosis, and death. In some cases, especially in pigs, the infection may progress so rapidly that death occurs before the development of diarrhea and is referred to as enteric colibacillosis complicated by shock. Diarrhea is mostly observed in the first few days after birth and affects one or more animals in a group. A less watery diarrhea may be observed in pigs during the first one to two weeks of age, or as early as two days post‐weaning, with low mortality and often with decreased weight gain. In affected piglets of this age group, co‐infection with other pathogens such as transmissible gastroenteritis virus, rotavirus, or coccidia, is often observed. F4‐producing isolates occasionally proliferate rapidly in the small intestine of young pigs and induce symptoms of shock and rapid death. Enteric colibacillosis complicated by shock occurs in unweaned and recently weaned pigs, and manifests as rapid death with cutaneous cyanosis of the extremities, or less acutely with hyperthermia, diarrhea, and anorexia. Table 7.2 Major types of Escherichia coli implicated in enteric diseases of animals. Table 7.3 Extraintestinal diseases of animals caused by Escherichia coli. Post‐weaning diarrhea is seen as yellowish or gray fluid and most commonly starts three to five days after weaning, lasting up to a week and causing emaciation (Fairbrother and Nadeau 2019). Over several days, most of the pigs in a group may be affected and mortality of up to 25% may be observed. In farms where husbandry measures at weaning include addition of higher levels of protein of animal source, plasma, acidifying agents, or zinc oxide to the feed, peaks of diarrhea and enteric colibacillosis complicated by shock may be delayed, occurring up to eight weeks after weaning. Postmortem findings of post‐weaning diarrhea may be dehydration, dilation of the stomach, gastric infarcts in the mucosa of the stomach, intestinal dilation (by fluid) and congestion, and hyperemia of the small intestine. Intestinal contents vary from yellow to green, watery to mucoid with blood sometimes, and a characteristic odor. On histopathology, layers of E. coli are observed adhering to the mucosa of the jejunum and ileum in newborn and post‐weaning pigs with ETEC infection. Bacteria are usually located at a distance of approximately half to one bacterial width away from the microvilli. Typically, there is no microscopic lesion. ETEC colonize the crypts of Lieberkühn and cover the apex of the villi. In cases of enteric colibacillosis complicated by shock, typical microscopic lesions of hemorrhagic gastroenteritis, congestion, and microvascular fibrinous thrombi and villus necrosis may be observed in the mucosa of the stomach, small intestine, and colon. The O serogroups and virulence gene profiles (virotypes) of ETEC most commonly associated with diarrhea in post‐weaning pigs are shown in Tables 7.4 and 7.6. These ETECs are typically hemolytic and are predominantly O149, O8, O141, or O138. Table 7.4 Important virulence categories and factors, O serogroups and pathotypes of Escherichia coli causing disease in pigs. In calves and lambs, diarrhea is mostly observed in the first few days after birth. Animals produce large amounts of foul‐smelling pasty to watery feces varying from pale yellow to white and occasionally containing flecks of blood. In acute cases, extensive loss of fluid leads to a marked decrease in body weight within six to eight hours of the onset of diarrhea. The intestinal mucosa usually appears normal on histopathology. In dogs, ETEC have been associated with cases of diarrhea, especially in young animals, and rarely from healthy control groups of dogs. Stx is the critical virulence factor in diseases caused by STEC. Edema disease in pigs is the only animal disease for which the role of Stx is clearly established. This subgroup of STEC is referred to as EDEC. However, there is evidence to implicate STEC in diarrhea and dysentery in calves and lambs. Affected calves show attaching and effacing lesions in the terminal ileum, colon and rectum, with edema and neutrophil infiltration of the lamina propria and an exudate of neutrophils, mucus, and exfoliated epithelial cells in the lumen. The lesions are more severe in colostrum‐deprived than in colostrum‐fed calves and may be induced with Stx1‐ or Stx2‐positive STEC. Table 7.5 Important virulence categories and factors, O serogroups and pathotypes of Escherichia coli causing disease in cattle and sheep. Hemolytic uremic syndrome (HUS) occurs occasionally in young dogs of several breeds, in which there is a prodrome of bloody diarrhea followed by thrombocytopenia, microangiopathic hemolytic anemia, and anuric acute renal failure. The kidneys of affected dogs show renal proximal tubular necrosis and hemorrhage, and glomerular lesions consisting of hypertrophy, necrosis and loss of capillary endothelial cells, adherence of aggregated platelets to the basement membrane, fibrin thrombi, and fibrinoid necrosis of blood vessels. Edema disease may be sporadic or may affect many litters or an entire herd and may be first recognized as sudden death without signs of illness. Some affected pigs become inappetent, develop swelling of the eyelids and forehead, emit a peculiar squeal, and show incoordination and respiratory distress. There is no diarrhea or fever. Pigs that die of edema disease typically show gross lesions of edema and hemorrhage in some or all of the following sites: the subcutaneous tissues of the eyelids and forehead, the greater curvature of the stomach; the mesenteric lymph nodes, the colonic mesentery, and the brain, especially the cerebellum. Light and electron microscopy identify degenerative changes in vascular endothelial cells, thrombosis in blood vessels, perivascular edema and necrosis of vascular smooth muscle. EDEC recovered from affected pigs most commonly belong to O group 139, and less frequently to O group 138, or 141 (Table 7.4). EDEC may or may not be enterotoxigenic. Those hybrid strains which are enterotoxigenic are known as ETEC/STEC and are most commonly associated with diarrhea. EPECs are a cause of diarrhea in several animal species, most importantly, in rabbits, pigs, and dogs. These strains induce AE lesions on the intestinal mucosa and are grouped in a category of E. coli called attaching and effacing E. coli (AEEC), which also includes EHEC strains. Healthy cattle, sheep and pigs, as well as dogs and cats, appear to be important reservoirs of AEEC, most of which are classified as EPEC producing the outer membrane protein adhesin intimin (Eae), encoded by the eae gene. Table 7.6 Important pathotypes, hybrid pathotypes, virotypes, and sequence types of Escherichia coli in pigs. EPEC may be associated with post‐weaning diarrhea in pigs (Fairbrother and Nadeau 2019). Histopathological lesions range from mild and scattered through the large and small intestine, to severe and involving mostly the cecum and colon. They include light to moderate inflammation of the lamina propria, enterocyte desquamation and some mild ulceration, and light to moderate villus atrophy in the small intestine. Extensive multifocal bacterial colonization of the surface epithelium by a thin layer of coccobacilli, often oriented in a palisade pattern, is observed. Typical intimate attachment of bacteria to intestinal epithelial cells and effacement of microvilli are observed on electron microscopy. EPEC are the pathogenic E. coli most commonly associated with diarrhea in dogs (Beutin 1999). There is at least one report of identical EPEC strains having been isolated from a diarrheic puppy and a young child in the same household. EPEC have also been isolated from cats. Cases in dogs have a history of gastrointestinal disease associated with histological and bacteriological evidence of AEEC. Typical attaching and effacing lesions are observed in the jejunum and ileum, to a lesser extent in the large intestine, and have been reported in the stomach of affected dogs. Many of these dogs originate from kennels and pet shops and are aged between 1.5 and 3 months. Co‐infection with other enteric pathogens, such as canine distemper virus, canine parvovirus, or coccidia, is often observed. EPECs are the only important class of pathogenic E. coli in rabbits, being one of the principal infectious agents in diarrheic rabbits and causing 25–40% losses. Certain serotypes of EPEC, such as O109:H2 and O8:H?, are mainly pathogenic for suckling rabbits whereas other serotypes, such as O26:H11, O20:H7, O109:H7, O153:H7, O128:H2, and O132:H2, are associated predominantly with disease in weaned rabbits. EPEC of serotypes O2:H6, O15:H‐, O103:H2 are associated with disease in both suckling and weaned rabbits (Milon et al. 1999). In general, O109:H2 strains tend to cause severe and lethal diarrhea in suckling but not weaned rabbits, whereas O15:H‐, O103:H2, and some O26:H11 strains tend to induce severe diarrhea with a high mortality rate in weaned rabbits. EPEC strains of other serotypes tend to cause a mild diarrhea with possible weight loss. In suckling rabbits, typical attaching and effacing lesions are observed over the entire length of the small and large intestines. In weanling rabbits, typical lesions are found mostly in the cecum, and to a lesser extent in the colon and ileum. In suckling rabbits, diarrhea due to EPEC occurs at 3–12 days of age, with mortality of up to 100% within a litter. In weanling rabbits, diarrhea is observed at 5–14 days after weaning, with mortality of 5–50% and decreased weight. ExPEC includes E. coli implicated in a wide range of infections including septicemia (septicemic E. coli, SEPEC), and infections of the urinary and genital tracts (uropathogenic E. coli, UPEC), the respiratory tract (avian pathogenic E. coli; APEC), and the mammary gland. The importance of ExPEC is increasing rapidly as they are constantly present in the intestinal microbiota of the various animal species and are increasingly becoming more resistant to antimicrobials (Vila et al. 2016; Biran and Ron 2018). Some ExPEC recovered from disease in humans and animals share several virulence factors and animals may be reservoirs of some of these pathogens that cause disease in humans. ExPEC are found in the normal intestinal microbiota. In contrast to ETEC, EPEC, and STEC, they are not characterized by the presence of a particular virulence factor or group of factors but usually possess a large number of virulence factors, which may vary greatly among strains. These are now commonly grouped into functional categories (see Section Virulence factors). There are several gene clusters for similar activities within each functional category and an ExPEC strain may carry one or more of them (Tables 7.4,7.5, and 7.7; Biran and Ron 2018). ExPEC strains usually carry at least one gene cluster for adherence, iron‐binding, and serum survival. ExPEC mostly occur in phylogroups B2, F, or G, although certain strains of other phylogroups have acquired sufficient virulence factors, probably by horizontal gene transfer, to become ExPEC (Vila et al. 2016). Conversely, some B2 strains that are ExPEC‐like also show characteristics defining diarrheagenic pathotypes such as adherent and invasive E. coli (Dogan et al. 2020). Many of the specialized traits distinguishing ExPEC from other E. coli are now considered as “fitness” rather than “virulence” factors, as they mediate host colonization and persistence without resulting in disease (Vila et al. 2016). In pigs, E. coli septicemia occurs in neonates and less frequently in suckling animals. It is characterized by an acute generalized infection, sometimes with diarrhea at the terminal stage, with signs of shock, often followed by death in three to eight hours, with fatality of up to 100%. The infection may become localized causing polyarthritis, pneumonia, metritis, abortion, or meningitis. Table 7.7 Important virulence categories and factors, O serogroups and pathotypes of Escherichia coli causing disease in poultry. In acute primary septicemia, there may be no gross lesions but congestion of the intestine, the mesenteric lymph nodes and the extraintestinal organs may be observed. In subacute cases, subserosal or submucosal hemorrhages and fibrinous polyserositis with gross lesions of pneumonia are usually observed, and may be accompanied by fibrinopurulent arthritis and meningitis. In pigs manifesting symptoms of shock and rapid death, typical histopathological lesions of septicemia such as hemorrhagic gastroenteritis, congestion, renal hemorrhage, and thrombi in the mucosa of the stomach and small intestine are observed. These lesions probably result from the rapid release of bacterial LPS from the intestine into the circulation. In ruminants, E. coli septicemia occurs in calves in the first few days of life and in lambs at two to three weeks of age. Bacteria enter the host across the intestinal epithelium, or through the umbilicus. The clinical signs and localizations are as described for pigs. Bacteria are excreted in nasal secretions, urine, and feces in affected animals. On postmortem examination of calves with peracute disease, there are usually petechial hemorrhages on the epicardium and serosal surfaces and there may be pulmonary edema and hemorrhage and enlargement of the spleen. Fibrinous polyarthritis and meningitis may be observed in chronic cases. In poultry, clinical signs vary with age. Young birds, often following yolk sac infection and/or omphalitis, may die rapidly of septicemia at a high prevalence with enlargement of the liver and spleen and increased fluid in body cavities. This first week mortality results from colonization of day‐old chickens by APEC originating from the parents (Christensen et al. 2021). This vertical transmission may occur following surface contamination of eggs from the environment of the parents and may lead to infection of the embryo if the bacteria penetrate the eggshell. The bacteria then spread horizontally during hatch and subsequent handling and transport of chicks. The risk of this transmission has been associated with broiler parents older than 43 weeks of age and following the use of floor eggs. Respiratory tract infection with APEC results in depression and fever in birds of four to nine weeks of age and may result in extensive economic losses, with up to 20% mortality. Air sacs of infected birds are thickened and often have a caseous exudate on the respiratory surface. On histopathology, edema is the earliest change and initial infections are characterized by airsacculitis with a serous to fibrinous exudate, an initial infiltration with heterophils and a subsequent predominance of macrophages, which is frequently followed by a general infection commonly resulting in perihepatitis and/or pericarditis. In laying birds, APEC may infect the oviduct via the left abdominal air sacs leading to salpingitis and loss of egg laying ability. APEC may invade the peritoneal cavity via the oviduct, leading to peritonitis and death in caged layer hens. APEC may also cause a syndrome called swollen head syndrome characterized by gelatinous edema of the facial skin and periorbital tissues, and caseous exudate in the conjunctival sac, facial subcutaneous tissues and lacrimal gland. APEC are also associated with asymptomatic cellulitis of the lower abdomen and thighs. The skin is discolored and yellowish fibrinocaseous plaques are found in the subcutaneous tissues underlying the skin lesions. E. coli is the pathogen that is most frequently implicated in urinary tract infections (UTI) in dogs, cats, and humans. Infection, which is more common in dogs than in cats, is most frequently manifested as cystitis but urethritis, pyelonephritis and prostatitis are also seen. E. coli mastitis can develop in any host species but is most common in dairy cattle, particularly in high‐producing animals, in the first two weeks after calving, and in animals with low somatic cell counts. Infection of the mammary gland by E. coli results in mild to severe inflammation and a clinical course of mastitis, which may be peracute, acute, or chronic. Granulomatous colitis is a rare type of inflammatory bowel disease occurring in Boxer dogs and French bulldogs (Dogan et al. 2020). Clinical signs include colitis, hematochezia, and weight loss, progressing to cachexia. Invasive E. coli may be observed in colonic macrophages. Isolated E. coli resemble ExPEC, possessing several of the ExPEC‐associated virulence genes. Virulence factors of pathogenic E. coli are now grouped by functional categories, which include adhesins, toxins, autotransporters, secretion systems, invasins, metal transport/uptake systems, protectins, regulation, and fitness factors (Johnson and Russo 2018; Sarowska et al. 2019). This facilitates the interpretation of the extensive virulence gene profiles that may be obtained on analysis of strains by whole‐genome sequencing, owing to the large numbers of potential virulence genes now found in referenced databases. Fimbriae (or pili) are rod‐like or fibrillar surface appendages on bacteria which mediate attachment to host tissues. Each fimbrial unit consists of hundreds of copies of a major subunit, which provides the structure and confers the antigenic specificity of the fimbriae. Fimbriae may also contain several copies of minor subunits, one of which may be an adhesin with specific binding properties. A system of F numbers was devised to designate fimbrial adhesins. The fimbriae originally named K88, K99, 987P, Fy, and F107, are now named F4, F5, F6, F17, and F18, respectively. Uncharacterized adhesins may play a role in infections with ETEC lacking genes for the recognized fimbriae. The F4 fimbria is a fine fibrillar structure. The major subunit of the F4 fimbriae constitutes the adhesin. F4 fimbriae are encoded by the fae locus on a plasmid, which often also carries genes for raffinose fermentation. The heat‐stable toxins and virotypes most commonly associated with F4 fimbriae are listed in Table 7.4. F4 fimbriae mediate bacterial adherence to the intestinal epithelium throughout most of the small intestine of pigs of all ages. Hence, colonization of the intestinal mucosa by F4‐positive ETEC occurs in both neonatal and post‐weaning pigs and may be observed in finisher pigs. Adherence due to F4 fimbriae is species specific, occurring almost exclusively in pigs. There are three variants of F4, ab, ac and ad, with the ac variant being most common. Some pigs are resistant to colonization by F4‐positive ETEC because they lack the receptors for the F4 adhesin that are encoded on chromosome 13. The allele (S) for the F4 receptor is dominant and genetic resistance to adherence by F4‐positive ETEC is inherited in a Mendelian fashion. Hence, there are three genotypes: ss (resistant), SS and Ss (susceptible). A PCR test for resistance/susceptibility of pigs to intestinal adherence of F4‐positive ETEC has been developed. If the sow is resistant, there is no anti‐F4 antibody in the colostrum in the absence of parenteral immunization, resulting in highly susceptible piglets. Susceptibility of pigs to F4ab/ac ETEC diarrhea has been linked to a polymorphism on MUC4 gene, although other receptors for F4ac are probably present (Dubreuil et al. 2016; Fairbrother and Nadeau 2019). The peptide that includes amino acids 125–163 of FaeG appears to be essential for F4 variant‐specific binding. F5 fimbriae are also fibrillar appendages. They are encoded by the fan locus, which is found on a plasmid. As with F4 fimbriae, the major fimbrial subunit (FanC) is the adhesin, allowing the fimbriae to bind laterally rather than by the tip. F5 fimbriae are produced by ETEC from pigs, cattle and sheep (Tables 7.4 and 7.5). F5 fimbriae bind to the specific receptor N‐glycolylneuraminic acid‐GM3 and mediate attachment of ETEC mostly to the posterior small‐intestinal mucosa in younger and occasionally in older animals. Hence, diarrhea due to F5‐positive ETEC is observed mostly in neonatal pigs, calves, and lambs. F6 are large rod‐shaped fimbriae, encoded by the fas locus on the chromosome and on plasmids and encodes three structural proteins, the major subunit FasA and minor subunits FasF and FasG. FasG is the adhesin. F6 mediates bacterial colonization mostly of the posterior small intestine in neonatal pigs. F6‐mediated intestinal colonization in older pigs is rarely observed and is thought to be inhibited by preferential binding of bacteria to F6 receptors in the intestinal mucus of older pigs rather than to receptors on the intestinal epithelium. Hence, diarrhea due to F6‐positive ETEC is observed almost exclusively in neonatal pigs. ETEC with this fimbria have been reported at low frequency in recent years. Three specific receptors have been reported for F6 fimbriae. The adhesin, FasG, binds both hydroxylated sulfatide and histone H1 on intestinal epithelial cells and the major subunit, FasA, binds hydroxylated ceramide monohexoside. F18 are long flexible filaments with a characteristic zig‐zag pattern that are encoded by the fed locus which is usually found on a plasmid. Binding is mediated by the adhesin FedF. There are two variants of F18, ab and ac, based on serological and nucleotide sequence differences. These variants are biologically distinct, F18ab being poorly expressed in vitro and in vivo and most often associated with Stx2e‐producing strains of E. coli implicated in edema disease, and F18ac being more efficiently expressed in vitro and in vivo and more commonly associated with ETEC strains. Nevertheless, both variants are involved in post‐weaning diarrhea or edema disease in pigs. It is probable that F18ab and F18ac bind to the same receptor. The expression of F18 receptor is age dependent, being lower in the first weeks of life and then maintained from three weeks onward. Genotypes susceptible and resistant to F18 adherence have been differentiated, and pigs with at least one copy of the dominant allele for the receptor are susceptible to epithelial cell adherence and hence to intestinal colonization. The receptor gene is localized on porcine chromosome 6, closely linked to the gene encoding halothane sensitivity, but there appears to be no co‐selection for F18 receptor and halothane sensitivity. Susceptibility to F18 E. coli infections was reported to be dependent on the activity of the FUT1 gene, encoding the alpha(1,2)‐fucosyltransferase. Pigs that lack this receptor can be identified by a PCR assay that detects a specific mutation in the gene. F41 are fibrillar appendages encoded by genes on the chromosome of both bovine and porcine ETEC strains. They are mostly expressed together with F5 fimbriae on ETEC of O groups 9 and 101, although some strains, mostly from pigs, may produce F41 alone. F41‐mediated colonization is observed in the posterior small intestine in neonatal pigs, calves, and lambs, and may result in diarrhea, whether F5 fimbriae are present or not. F17 are flexible fimbriae found mostly on bovine necrotoxigenic E. coli (NTEC) strains producing cytotoxic necrotizing factor (CNF) 1 or 2, encoded by genes on the chromosome in the former and on a plasmid in the latter. Six gene variants, a–f, have been described, based on differences in the major subunit F17A (Bihannic et al. 2014). F17 has also been found on bovine ETEC strains, although its role in diarrhea due to these strains has not been elucidated. F17 fimbriae consist of a major structural pilin (F17A) with a flexible tip fibrillum that contains the F17G adhesin. Like the adhesins of type I and P fimbriae, the lectin domain of F17G has an immunoglobulin‐like fold. F17G has its binding site located laterally and has been proposed to insert between microvilli in order to bind. The carbohydrate specificity of the F17a fimbriae is a terminal or internal N‐acetylglucosamine on O‐linked oligosaccharides of bovine mucins or intestinal glycoproteins (Dubreuil et al. 2016). The adhesin involved in diffuse adherence 1 (AIDA‐1) is an autotransported 100‐kDa mature outer‐membrane protein, which mediates bacterial attachment to intestinal epithelial cells. AIDA‐1 is a self‐associating autotransporter, that can mediate bacterial autoaggregation through self‐associations. AIDA‐1 was originally detected in E. coli isolates from humans with diarrhea, and homologs have been detected subsequently in other E. coli, including strains from pigs with edema disease or post‐weaning diarrhea, particularly in strains of the virotypes Stx2e:F18 and F18 alone. A high percentage of the AIDA‐positive porcine strains carry the astA gene for EAST1. In these strains, AIDA is encoded by genes forming an operon including the aidA gene, on a plasmid. ETEC isolates of the STb or STb:EAST‐1 virotypes from neonatal or weaned pigs may also be AIDA‐positive and induce diarrhea in neonatal pigs. AIDA is also present in non‐ETEC. AIDA‐1 was shown to be involved in biofilm formation and in colonization of newborn experimentally infected pigs. Interaction between adhesins and host epithelial cells is believed to be an early development in formation of the attaching and effacing lesion. Long polar fimbriae (LPF; Zhou et al. 2019) have been suggested as an adhesin that might fill this role. LPF comprises two types, LPF1 and LPF2, both initially found in EHEC, and both playing essential roles in adherence to intestinal cells. LPF also appears to be required for active translocation of EHEC across human M cell monolayer. A type IV fimbria, called hemorrhagic coli pilus (HCP), has been shown to promote adherence of EHEC O157:H7 to bovine and human epithelial cells and to pig and cattle gut explants. Antibodies against HCP were detected in the sera of patients with HUS but not in the sera of healthy persons. A critical component of the locus of enterocyte effacement (LEE)‐encoded gene cluster is the eae (E. coli attaching and effacing) gene that encodes the outer membrane protein adhesin intimin (Eae; Mare et al. 2021). Intimin binds to the translocated intimin receptor (Tir), which has been injected into the host cell by the T3SS of AEEC, leading to a tight attachment of the bacterium to the host cell. Variation in the amino acid sequence in the C‐terminus of intimin has led to recognition of at least 30 intimin subtypes in STEC and EPEC. Although subtypes are associated with bacterial serotype, host specificity and tissue tropism, they are interchangeable between strains and can interact with any Tir‐binding domain. Many human EPECs possess plasmid‐encoded type IV bundle‐forming pili (Bfp), which are responsible for localized adhesion of these bacteria to HeLa cells in vitro and are proposed to be responsible for initial loose adherence of bacteria to each other and to the target enterocyte apical membrane (Pearson et al. 2016). bfpA encodes the major pilin subunit of Bfp. The presence of bfpA is used to subdivide EPEC into two distinct groups, typical (bfpA+) and atypical (bfpA−). Dog EPEC strains may possess a bfpA‐related gene. Rabbit EPEC strains also often possess one of the fimbrial adhesins AF/R1 (adhesive/rabbit 1), AF/R2, or Ral (rabbit EPEC adherence locus), which appear to be involved in initial bacterial adherence to the rabbit intestinal epithelium (Mainil and Fairbrother 2014). These fimbriae may be involved in initial attachment of rabbit EPEC to the enterocyte apical membrane, as proposed for BfpA of human EPEC. Adhesins on the surface of ExPEC bacteria may be classified as fimbrial, nonfimbrial, or atypical, the last contributing to adhesion but possessing other primary functions (Aleksandrowicz et al. 2021). For many of these adhesins, there is epidemiological or experimental evidence that they are involved in bacterial virulence (Johnson and Russo 2018). Type I Fimbriae Type I fimbriae are found in all ExPEC pathotypes (Sarowska et al. 2019). They are encoded by the fim operon found on the chromosome. The major fimbrial subunit is FimA and the FimH protein located at the tip is responsible for binding to glycoprotein receptors on host cells. Binding of type I fimbriae to receptors is inhibited by mannose. Expression of type I fimbriae is phase variable which may permit a role in colonization of certain organs such as the lower respiratory tract of chickens, but not in other sites. P Fimbriae P fimbriae are found in ExPEC of the UPEC, SEPEC, and APEC pathotypes. They are encoded by the pap operon in PAI on the chromosome. The major fimbrial subunit is PapA and the PapG protein is responsible for mannose‐resistant binding to glycolipid receptors containing Gal‐alpha‐(1,4)Gal galactoside residues on host cells. Three molecular variants, PapGI, PapGII, and PapGIII, have been identified, and are associated with different target organs and pathological changes. Other Fimbriae Other fimbrial adhesins found on ExPEC include S fimbriae (sfa gene), Stg (stg), AC/I (fac), F1C (foc), Dr. (dra), Escherichia common pili (ecp), Yad (yad), Yqi (yqi), curli (csg), type IV (pil; rci), GlcNac‐specific (G, F17c) (gafD), and M (bmaE; Dale and Woodford 2015; Johnson and Russo 2018; Sarowska et al. 2019; Aleksandrowicz et al. 2021). Afimbrial Adhesins Afimbrial adhesins are mostly found in UPEC and are encoded by a cluster of afa genes. The major structural subunit is AfaB. The receptor for the family of Dr. fimbriae and Afa afimbrial adhesins is the decay‐accelerating factor, expressed on the surface of erythrocytes and cells of other tissues (Sarowska et al. 2019). Other Non‐fimbrial Adhesins Other non‐fimbrial adhesins found on ExPEC include the autotransporters AatA (aatA gene), AatB (aatB), temperature‐sensitive hemagglutinin (Tsh) (tsh; see Section Autotransporters), antigen 43 (agn43), Iha (iha), CS31A (clpG), non‐fimbrial adhesin (nfa), and heat‐resistant hemagglutinin (hra). Atypical adhesins include flagella (fliC), LPS (waa/rfa), and type VI secretion system (vgrG/clpV). E. coli enterotoxins cause disturbances in intestinal fluid metabolism but do not produce pathological lesions or morphological changes in the intestinal mucosa. Two major classes of enterotoxin are produced by ETEC (Dubreuil et al. 2016): low molecular weight, poorly antigenic heat‐stable toxins, which are resistant to 100°C for 15 minutes, and high molecular weight, highly antigenic heat‐labile toxin (LT), which is inactivated at 60°C for 15 minutes. Both types of enterotoxin are plasmid encoded. This family of enterotoxins consists of STa (or STI), STb (or STII) and EAST1, differentiated on the basis of size, molecular structure, and biological activity. The LT family of enterotoxins consists of LTI and LTII, which differ in their antigenicity but have similar biological activity associated with similar A subunits. The secretion and delivery of LT and STa appear to be associated with bacterial contact with the intestinal epithelium (Zhu et al. 2018). STa is an 18‐ or 19‐amino acid peptide of about 2000 Da, which is produced as a 72‐amino acid pre‐pro‐peptide, transported across the inner membrane, folded in the periplasm in which three intramolecular disulfide bonds are formed by DsbA protein, then secreted through TolC. STa has been designated STaP (produced by bovine, porcine, and human ETEC) or STaH (produced by human ETEC), based on minor differences in composition. All forms of STa have a conserved C‐terminal 13 amino acids which include three disulfide bonds. STa binds to a guanyl cyclase C (GCC) glycoprotein receptor on the brush border of villous and crypt intestinal epithelial cells and activates guanyl cyclase, which stimulates production of cyclic guanosine monophosphate (cGMP; Figure 7.1a; Liu et al. 2020b). Elevated concentration of cGMP results in (i) phosphorylation of cGMP‐dependent protein kinase II (cGMPKII), which phosphorylates and thereby stimulates the cystic fibrosis transmembrane conductance regulator (CFTR) chloride channel; and (ii) inhibition of phosphodiesterase 3 (PDE3) leading to accumulation of cAMP which activates protein kinase A (PKA). PKA then turns on CFTR and also inhibits sodium/hydrogen exchanger 3 (NHE3) leading to release of Cl− and HCO3− through CFTR into the intestinal lumen and to inhibition of Na+ reabsorption from the intestinal lumen (Figure 7.1a). Binding of STa to GCC on villous enterochromaffin cells also appears to stimulate neural pathways. Here, the increased levels of cGMP trigger the release of paracrine mediators such as serotonin and neurotensin into the subepithelial space, leading to release of secretagogues, such as vasoactive intestinal peptide, which attach to receptors on the enterocytes and stimulate fluid secretion. Based on the concentration and affinity of STa receptors, the posterior jejunum appears to be the major site of hypersecretion in response to STa. STa also appears to induce intestinal secretion in mice by binding to receptors other than GCC (Sellers et al. 2008). Figure 7.1 Mechanisms of action of E. coli enterotoxins. (a) STa: Binding of STa to its guanyl cyclase C (GCC) receptor activates guanyl cyclase, which converts guanosine triphosphate (GTP) to cyclic guanosine monophosphate (cGMP). Elevated levels of cGMP: (i) activate cGMP‐dependent protein kinase II (cGMPKII), which turns on the cystic fibrosis transmembrane regulator (CFTR); and (ii) inhibit phosphodiesterase 3 (PDE3), leading to accumulation of cyclic adenosine monophosphate (cAMP) which activates PKA. PKA then turns on CFTR and also inhibits sodium/hydrogen exchanger 3 (NHE3) leading to release of Cl− and HCO3− through CFTR into the intestinal lumen and to inhibition of Na+ reabsorption from the intestinal lumen. (b) STb: STb binds to sulfatide and thereby activates GTP‐binding regulatory protein Gαi3, which leads to an influx of Ca2+. The high concentration of Ca2+ activates CaMKII and protein kinase C (PKC). CaMKII opens a CaCC and activates CFTR while PKC stimulates CFTR and inhibits NHE3. These actions lead to accumulation of Cl−, HCO3− and Na+ ions and water in the intestinal lumen. The high level of Ca2+ also causes increased production of secretagogues prostaglandin E2 (PGE2) and 5‐hydroxytryptamine (5‐HT) through its effect on phospholipases A2 and C. (c) LT: LT binds to GM1 ganglioside and the LT‐GM1 complex is taken up into endosomes (E) by receptor‐mediated endocytosis. The toxin‐GM1 complex is taken to the Golgi then the ER, where the LT A subunit is nicked by a protease and a disulfide bond is cleaved to release the A1 fragment. The A1 enzyme, activated by an adenosine diphosphate (ADP)‐ribosylation factor, transfers ADP‐ribose from nicotinamide adenine dinucleotide (NAD) to Gsα, a regulatory protein. The modified Gsα loses its intrinsic GTPase activity and turns on adenyl cyclase constitutively (conversion of GTP to guanosine diphosphate, GDP, turns off adenyl cyclase). The elevated levels of cAMP activate PKA which activates the CFTR resulting in hypersecretion of Cl− and HCO3− ions from the cell. PKA also inhibits absorption of Na+ from the intestinal lumen through NHE3. Water follows into the intestinal lumen by osmotic drag. Source: Courtesy of Aida Minguez Menendez, Faculty of Veterinary Medicine, University of Montreal. The effects of STa are reversible. STa is active in infant mice and young pigs but is less active in older pigs, consistent with decreasing affinity and density of STa receptors with increasing age. Not surprisingly, ETEC strains that produce STa as the only enterotoxin are associated with disease in neonatal pigs, calves, and lambs.
7
Escherichia coli
Introduction
Category of E. coli
Categories of virulence genes
Bacterial localization, site of infection
Site of pathological changes and clinical manifestation
Non‐pathogenic
Commensal
None
Microbiota (intestine, respiratory tract, urogenital tract etc.), environment
None
Pathogenic
Enterotoxigenic
Adhesins, toxins
Intestine
Intestine. No pathological changes
Shiga toxin‐producing (edema disease)
Adhesins, toxins
Intestine
Intestine, extraintestinal sites
Shiga toxin‐producing (enterohemorrhagic)
Adhesins, toxins, secretion systems
Intestine
Intestine, extraintestinal sites
Enteropathogenic
Adhesins, secretion systems
Intestine
Intestine
Extraintestinal pathogenic
Adhesins, toxins, invasins, auto‐transporters, metal transport/uptake systems, protectins
Intestine (microbiota)/extraintestinal sites
Extraintestinal sites
Mammary pathogenic
Metal transport/uptake systems, protectins
Intestine (microbiota)/mammary gland
Mammary gland
Pathotypes of E. coli and Associated Disease and Pathological Changes in Animals
Enterotoxigenic E. coli
Animal species
Disease
Pathotype (Sub‐pathotype)of E. coli
Cattle
Neonatal diarrhea
Enterotoxigenic
Diarrhea/dysentery
Shiga toxin‐producing
in 2‐ to 8‐week‐old calves
Possibly extraintestinal pathogenic
Pigs
Neonatal diarrhea
Enterotoxigenic
Post‐weaning
Enterotoxigenic, enteropathogenic
diarrhea
Enterotoxigenic/Shiga toxin‐producing
Edema disease
Shiga toxin‐producing (edema disease)
Dogs
Diarrhea
Enterotoxigenic
Enteropathogenic
Granulomatous colitis
Extraintestinal pathogenic
Rabbits
Diarrhea
Enteropathogenic
Animal species
Disease
Pathotype (Sub‐pathotype) of E. coli
Cattle
Septicemia
Extraintestinal pathogenic (septicemic)
Mastitis
Mammary pathogenic
Pigs
Septicemia
Extraintestinal pathogenic (septicemic)
Pneumonia
Extraintestinal pathogenic (septicemic)
Mastitis
Mammary pathogenic
Dogs
Urinary tract infection
Extraintestinal pathogenic (uropathogenic)
Pyometra
Extraintestinal pathogenic (uropathogenic)
Septicemia
Extraintestinal pathogenic (septicemic)
Pneumonia
Extraintestinal pathogenic (septicemic)
Cats
Urinary tract infection, pyometra
Extraintestinal pathogenic (uropathogenic)
Septicemia
Extraintestinal pathogenic (septicemic)
Poultry
Omphalitis/yolk sac infection
Extraintestinal pathogenic (avian pathogenic)
First week mortality
Air‐sacculitis/septicemia
Cellulitis
Salpingitis
Peritonitis
Swollen head syndrome
Pathotype
Virulence category and factors
O serogroup
Enterotoxigenic
Adhesins: F4, F5, F6, F18, F41, AIDA
8,9,20,45,64,101
Toxins: STa, STb, LT, EAST1, Hly
138,141,147,149,157
Enterotoxigenic/Shiga toxin‐producing
Adhesins: F4, F5, F6, F18, F41, AIDA
141,147
Toxins: STa, STb, LT, EAST1, Stx2e, Hly
Shiga toxin‐producing (edema disease)
Adhesins: F18, AIDA
2,9,101,107,120,121,125,138,139, 141,147,149,154,157
Toxins: Stx2e, Hly
Enteropathogenic
Adhesins: Intimin (Eae)
Secretion systems: Tir, EspA, EspB, EspD
Toxins: EspC
45,103,123
Extraintestinal pathogenic (septicemic)
Adhesins: P (PapC), S (Sfa), F1 (Fim), Curli (Csg)
6,8,9,11,15,17,18,20,45, 60,78,83,93,101,112,115,116
Toxins: Cnf1/2, Cdt, Clb, HlyA
Autotransporters: Vat
Invasin: IbeABC
Metal transport/uptake: IutA/iucD, Irp, sitABCD, IroN, FyuA, FepA,
Protectins: K1(KpsMII), Iss, OmpT
Extraintestinal pathogenic (uropathogenic)
Adhesins: P, S
1,4,6,18
Toxins: Cnf1/2, Cdt
Metal transport/uptake: IutA/iucD, Irp, sitABCD, IroN, FyuA, FepA
Protectins: K1(KpsMII), Iss, OmpT
Shigatoxigenic E. coli
Pathotype
Virulence category and factors
O serogroup
Enterotoxigenic
Adhesins: F5, F41
8,9,20,45,64,101
Toxins: STa, EAST1
Shiga toxin‐producing
Adhesins: Intimin (Eae)
5,8,20,26 103,111,118,145
Toxins: Stx1, Stx2
Enteropathogenic
Adhesins: Intimin (Eae)
Secretion Systems: Tir, EspA, EspB, EspD
Toxins: EspC
Extraintestinal pathogenic (septicemic)
Adhesins: P (PapC), S (Sfa), F1 (fim), Curli (Csg)
8,9,15,26,35,45,78,86,101,115,117,137
Toxins: Cnf1/2, Cdt, Clb, HlyA
Autotransporter: Vat
Invasin: ibeABC
Metal transport/uptake: IutA/iucD, Irp, SitABCD, IroN, FyuA, FepA,
Protectins: K1 (KpsMII), Iss, OmpT
Mammary pathogenic
Lipopolysaccharide
Enteropathogenic E. coli
Pathotype or hybrid pathotype
Pathovirotype
Most common virotypes
Most common sequence types when tested
Enterotoxigenic (ETEC)
ETEC: F4
LT:STb:F4
10, 90, 100
LT:STa:STb:F4
100
LT:STb:F4:F6
STa:STb:F4
772
ETEC: F18
STa:STb:F18
10, 5786,
LT:STa:STb:F18
42, 847
STa:F18
LT:STb:F18
1266
ETEC:F4:F18
LT:STa:STb:F4:F18
ETEC
STb
STa:STb
STb:AIDA
Enterotoxigenic/Shiga toxin‐producing (STEC)
ETEC/STEC:F18
STa:STb:Stx2e:F18
10
LT:STb:Stx2e:F18
LT:STa:Stx2e:F18
LT:STa:STb:Stx2e: F18
88
STEC
STEC:F18
Stx2e:F18
10
STEC
Stx2e
Enteropathogenic (EPEC)
EPEC
Eae
10, 29, 48,
ETEC/STEC/EPEC
ETEC/STEC/EPEC:F4
LT:Stx2e:Eae:F18
4214
ETEC/EPEC
ETEC/EPEC:F18
LT:STb:Eae:F18
Extraintestinal Pathogenic E. coli
Pathotype
Virulence category and factors
O serogroup
Enteropathogenic
Adhesins
Intimin (Eae)
Secretion systems: Tir, EspA, EspB, EspD
Toxins: EspC
Extraintestinal pathogenic (avian pathogenic)
Adhesins: P (F11), S (Sfa), F1 (Fim), Curli, Stg, Yqi, Tsh
1,2,5,6,8,15,18,27,35,53,63,64, 78,86,88,109,111,115,125,129,166,169
Toxins: Cnf1/2?, Cdt?, HlyF
Autotransporter: Tsh, Vat
Invasin: ibeABC
Metal transport/uptake: IutA/iucD, Irp, SitABCD, IroN, FyuA, FepA, ChuA
Protectins: Capsule K1 (KpsMII), O78 LPS, Iss, OmpT
Virulence Factors of Pathogenic E. coli
Adhesins
Enterotoxigenic E. coli Fimbrial and Afimbrial Adhesins
F4 Fimbriae
F5 Fimbriae
F6 Fimbriae
F18 Fimbriae
F41 Fimbriae
F17 Fimbriae
Adhesin Involved in Diffuse Adherence‐I, a Nonfimbrial Adhesin
Attaching and Effacing E. coli Adhesins
Long Polar Fimbriae
Hemorrhagic Coli Pilus
Intimin
Bundle‐forming Pili
Adhesins of Rabbit EPEC
Extraintestinal Pathogenic E. coli Adhesins
Fimbrial Adhesins
Nonfimbrial Adhesins
Atypical Adhesins
Toxins
Enterotoxins
STa
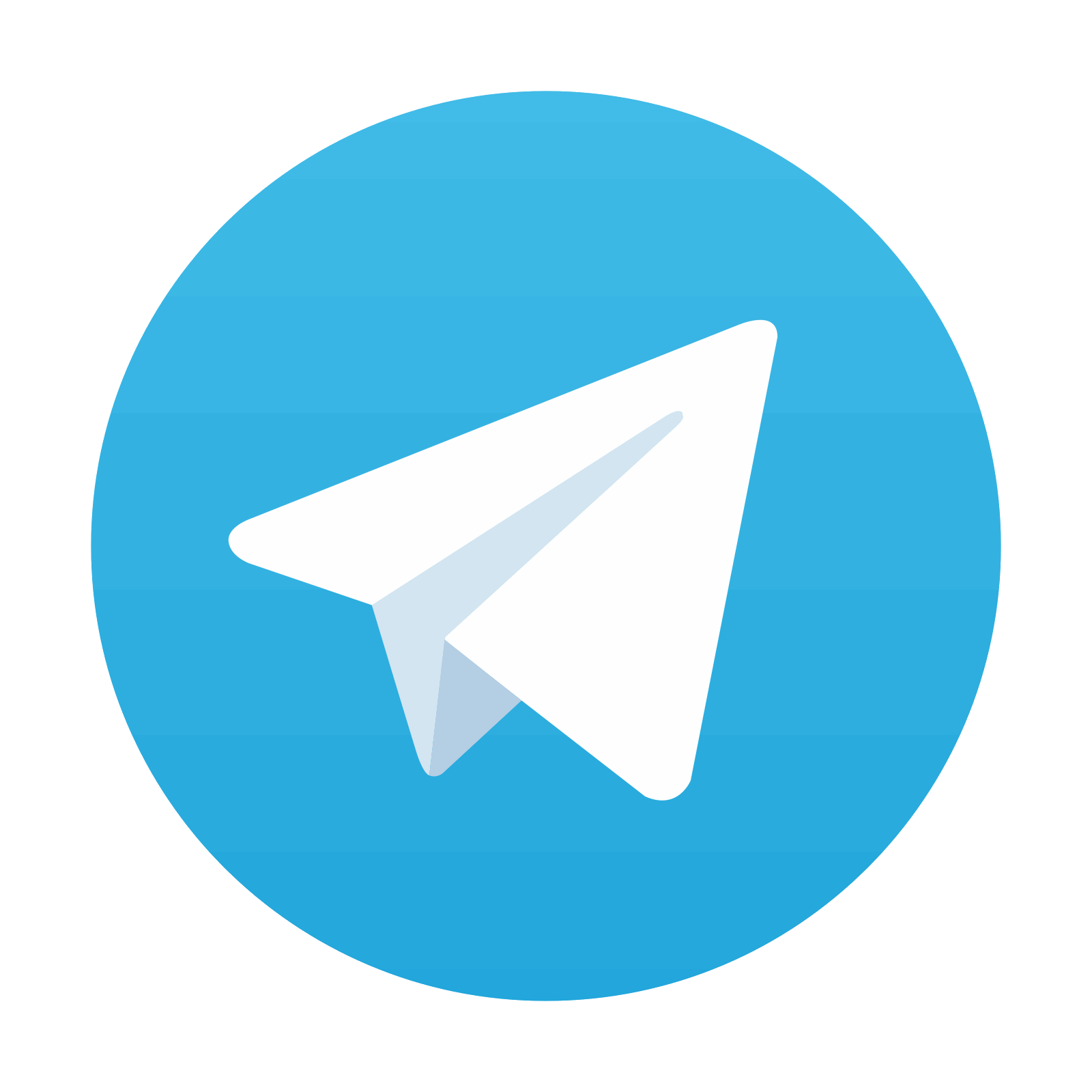
Stay updated, free articles. Join our Telegram channel

Full access? Get Clinical Tree
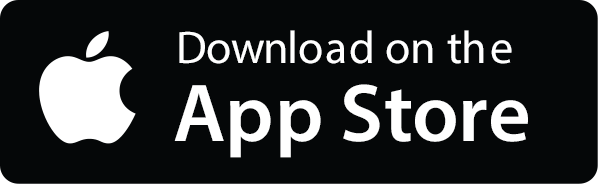
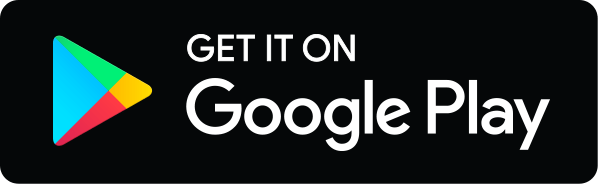