Evelien Dierick, Evy Goossens, John F. Prescott, Richard Ducatelle, and Filip Van Immerseel Clostridia can cause a large spectrum of enteric, neurotoxic or histotoxic diseases in humans, as well as in pets, livestock and wildlife. These opportunistic pathogens affect a diverse array of host species, often resulting in species‐specific diseases. They produce far more toxins than any other bacterial genus, explaining the diversity in disease manifestations. This chapter focusses on enteric clostridial infections in animals, presenting as enteritis, enterocolitis, or enterotoxemia. Although many enteric clostridia have been described, this chapter focuses on Clostridium perfringens and Clostridioides difficile. The role of other clostridia involved in enteric disease is summarized only briefly, since details of pathogenesis are scanty. A wide range of enteric diseases in both animals and humans can be attributed to C. perfringens. This Gram‐positive, rod‐shaped bacterium is widely distributed in nature. In addition to its presence in soil, sewage, and contaminated foods, C. perfringens is a normal intestinal inhabitant of many warm‐blooded animals. As an aerotolerant anaerobe, it can survive in a growth‐arrested state in the presence of oxygen or low concentrations of superoxide‐ or hydroxyl‐radical‐generating compounds. Its aerotolerant character facilitates interhost transmission since the bacterium can survive on aerobic surfaces and in oxygen‐exposed tissues. Its aerotolerance, with its ability to form heat‐resistant endospores, allows the bacterium to be highly persistent in the environment. This spore‐forming pathogen can germinate in and persistently colonize the gastrointestinal tract. Germination is triggered by a wide variety of small molecules but, perhaps most importantly, primary bile acids (glycocholate, cholate, taurocholate) in the gastrointestinal tract of mammals also induce germination. By contrast, secondary bile acids, such as deoxycholate, produced by resident commensal bacteria inhibit germination, proliferation and bacterial colonization (Kiu and Hall 2018). C. perfringens is mesophilic, able to grow at temperatures of 12–50°C, with an optimum of 37–45°C. It is one of the fastest growing microorganisms known, with a generation time of 7 minutes when grown at 45°C and 12–17 minutes at 37°C. Under ideal circumstances, it can grow at least twice as fast as other intestinal commensals, giving it a competitive advantage in niche colonization, sometimes enhanced by bacteriocin production. Although for a long time described as non‐motile, a type IV pili‐dependent gliding motility has been reported in C. perfringens (Juneja et al. 2010); however, their role in pathogenesis has not been investigated. C. perfringens is highly specialized as an “anaerobic flesh‐eater” (Shimizu et al. 2002) capable of rapidly breaking down both dead and living animal tissue. It can also adapt to cause disease in specific animal species. Its specialization in nature may originally have been the decomposition of dead animals. The genomes of numerous C. perfringens strains have been completely sequenced, leading to insights in energy metabolism, biosynthesis, sporulation, virulence, and other aspects of its pathogenic lifestyle. In addition to the chromosome, C. perfringens importantly can harbor large related conjugative virulence plasmids, which can be transferred between C. perfringens. In addition to the conserved core, over 300 variable genomic islands can be distinguished, all contributing to the genetic diversity within this species. Whereas all C. perfringens have a complete set of genes for glycolysis and glycogen metabolism, no genes encoding for tricarboxylic acid cycle or respiratory chain‐related proteins have been identified, both of which are mostly oxygen‐requiring processes. A wide variety of genes encoding glycolytic enzymes are present within the C. perfringens genome, explaining its ability to degrade a large array of complex sugars. After the breakdown of complex sugars, many simple sugar compounds can then be used in the main glycolytic pathway. Fermentation products such as alcohol, lactate, butyrate, and acetate are produced. In the anaerobic fermentation pathway, when pyruvate is converted into acetyl‐coenzyme A by pyruvate‐ferrodoxin oxidoreductase, both CO2 and H2 gases are produced, which aids the growth and survival of the anaerobic bacteria inside the host (Shimizu et al. 2002; Myers et al. 2006). C. perfringens lacks crucial enzymes required for the biosynthesis of arginine, aromatic amino acids, branched amino acids, glutamate, histidine, lysine, methionine, serine, and threonine, and cannot grow in an environment with limited supply of such essential amino acids. To obtain these and other essential nutrients from its host, this non‐invasive bacterium secretes a vast array of degradative enzymes and toxins. Many virulence factors have been identified in C. perfringens, including toxins such as alpha‐ (CPA), beta‐ (CPB), beta2‐ (CPB2), epsilon‐ (ETX) and iota toxin (ITX), perfringolysin O (PFO), enterotoxin (CPE), NetB and NetF, and numerous other non‐toxin tissue‐degrading enzymes such as sialidases (NanI, NanJ, NanH), collagenase(s), and hyaluronidases. However, the strain‐to‐strain difference in toxin production is high (Kiu and Hall 2018; Dierick et al. 2021), reflecting in some cases host adaptation of strains. Based on the presence or absence of specific toxin genes, C. perfringens strains can be divided into different toxinotypes. The classical system (type A–E), based on the CPA‐, CPB‐, ETX‐, and ITX‐encoding repertoire of the strains, was extended in 2018 by addition of two additional toxinotypes, F and G, based on the presence of genes encoding C. perfringens enterotoxin (CPE) and NetB toxin (Rood et al. 2018). Table 28.1 gives the updated current toxinotyping system. C. perfringens is omnipresent throughout nature. Type A is the most common toxinotype, being widespread in the environment and in the intestine of both healthy and diseased animals. Other toxinotypes are less frequent in the intestinal tract of healthy animals and humans. For instance, C. perfringens type G is present in the gastrointestinal tract of healthy broiler chickens, although in lower numbers as compared to type A. These bacteria can only thrive in a favorable gastrointestinal environment, resulting in proliferation and overgrowth of commensal gut microbiota. Fecal shedding by adult animals results in early intestinal colonization of neonates, partly explaining the association of some toxinotypes with neonatal necrotizing enteritis in farm animals. Fecal shedding is the main source of clostridial infection. Widespread contamination has also been demonstrated through the isolation of C. perfringens from bedding, transport cages, drinking water, boots, fans, fly strips, and flies. Since C. perfringens is aerotolerant and forms heat‐resistant spores, the bacterium can survive in the environment for years. Table 28.1 Classification of Clostridium perfringens strains into toxinotypes A to G based on their toxin production and presence of toxin genes. Two lead candidates for possible future additional toxinotypes are NetF strains associated with canine haemorrhagic enteritis syndrome and foal necrotizing enteritis, discussed in this chapter, and BEC toxin‐producing strains associated with acute foodborne gastroenteritis in humans. Source: Rood et al. (2018), with the permission of Elsevier. Whole genome sequence analysis of C. perfringens strains originating from different sources has revealed a highly divergent open pangenome with indications of significant horizontal gene transfer. Horizontal gene transfer between C. perfringens strains is crucial in understanding both evolution and epidemiological aspects of this bacterium (Wisniewski and Rood 2017; Mehdizadeh Gohari et al. 2021). In addition to the chromosome, genetic information can be located on plasmids which, if they are conjugative, can be transferred from one strain to another. These conjugative plasmids may contain antibiotic‐resistance genes or genes encoding toxins or other virulence factors, thereby playing an critical role in C. perfringens pathogenesis. The most well‐known conjugative plasmid family is the tcp family first recognized in the tetracycline resistance plasmid pCW3 (Rood et al. 1978). Sequencing analysis revealed that these tcp conjugative plasmids contain a 35 kb highly conserved core region, encoding critical genetic elements for replication, maintenance, stability, partitioning, regulation, and transfer of the plasmid. A tcp conjugation locus is found in this conserved region, enabling efficient conjugative transfer. These plasmids harbor a unique replication gene rep and partitioning locus parMRC. The highly variable accessory region can contain the genes encoding toxins, bacteriocins, antibiotic resistance determinants, and other likely virulence factors. Almost all major toxins of C. perfringens have been related to these tcp plasmids, including CPB, ETX, ITX, TpeL, NetF, NetB, and sporadically CPB2. CPE is located on this type of plasmid in type C, D, 30% of the type F strains and in all NetF‐positive strains. These variable regions are often associated with mobile genomic elements such as transposases, insertion sequences and prophages, explaining the large variability within plasmids, and playing a crucial role in the continued evolution of the tcp conjugative plasmid family. Figure 28.1 shows the conserved and accessory regions of this family for all of the major toxinotypes (Parreira et al. 2012). The high similarity between the C. perfringens toxin plasmids suggests that they are derived from a common progenitor plasmid that has acquired different virulence genes throughout evolution, likely as part of a process of host adaptation. The tcp virulence plasmids can only be found in C. perfringens. Interestingly, up to five of these closely‐related tcp plasmids are able to coexist within an individual strain (Wisniewski and Rood 2017). Plasmid compatibility can be explained by the existence of multiple partitioning systems, of which over 10 have been identified (Parreira et al. 2012; Adams et al. 2015). C. perfringens likely maintains its major toxin genes (other than cpa) on different conjugative plasmids because this confers virulence plasticity and adaptability. Figure 28.1 Comparative analysis of Clostridium perfringens tcp conjugative plasmids encoding tetracycline or toxin genes. From the top: tetracycline gene (pCW3); netB toxin gene (pNetB‐NE10); netB toxin gene (pJIR3535): cpe enterotoxin and cpb2 toxin genes (pCPF5603) cpe enterotoxin gene (pCPF4969); itx iota toxin genes (pCPPB1); etx epsilon toxin gene (p8533etx); cpb2 toxin gene (pCPB2‐CP1); cpb2 toxin gene (pJIR3844). Conserved regions within the analyzed plasmids are highlighted by gray boxes, toxin genes in purple, and accessory genes in other colors. The netF and netG toxin genes are also present on tcp plasmids. Source: Based on Parreira et al. (2012) and Gohari et al. (2015). Recently, a second conjugative plasmid family has been described. The pCP13‐like family is characterized by a pcp conjugative transfer locus and has been associated with the CPB2 and BEC toxin. Unlike tcp, this locus is evolutionarily related to the conserved conjugation system of other pathogenic clostridial species. Plasmid acquisition has been crucial in evolution, leading to the differentiation of C. perfringens pathotypes. This has been shown in vitro with several tcp conjugative virulence plasmids. However, other genes on the virulence plasmids have been shown to be, or are very likely to be, involved in pathogenesis (Parreira et al. 2012; Wade et al. 2020). Genes found on the chromosome of different strains are also likely or have been shown to be involved in host specific or possibly environmental adaptation (Lepp et al. 2013; Wade et al. 2020). The extent and the basis of host adaptation of C. perfringens is still a largely unexplored topic. Although not fully proven, possession of a TpeL‐encoding virulence plasmid is generally accepted as enhancing the virulence of toxinotype G avian necrotic enteritis strains encoding the netB gene. The entire genome of a toxinotype A C. perfringens was first sequenced in 2002. Since then, a wide range of strains, covering all toxinotypes from different hosts and environments have been sequenced. Large‐scale comparative genomic analysis shows substantial variability between strains. The genome of C. perfringens is 2.9–4.1 Mb in size, with a low G+C% content. The core genome comprises 1192 genes, covering one third of the entire bacterial genome. A wide variety of accessory genes have been identified, resulting in a highly versatile, open, and plastic pangenome (Feng et al. 2020; Abdel‐Glil et al. 2021). This is expected to expand rapidly with the addition of sequenced C. perfringens genomes. Such a remarkably extensive pangenome makes C. perfringens the most highly diverse of Gram‐positive bacterial species. Most variable and unique regions in the accessory genome of C. perfringens are associated with mobile genomic elements, including insertion sequences, transposases, and prophages. Five lineages can be distinguished, reflecting the strong influence of the environment/host on bacterial phylogeny and their involvement in different diseases (Feng et al. 2020; Abdel‐Glil et al. 2021). Studying the large variability of accessory genes potentially has given insights in the disease‐inducing capacity of C. perfringens strains but linking these regions in specific genomes to diseases is complex. Additional research is required to verify whether the identified gene has indeed a role during pathogenesis, is non‐functional, or is the result of other host or environmental adaptation throughout evolution. Study of multiple clostridial knockout mutants will aid this research (Keyburn et al. 2008; Wade et al. 2020). Further genome study using carefully selected strains will undoubtedly lead to improved understanding of host adaptation and of disease associations. The genomes of poultry isolates of toxinotype A and G have been studied in the greatest depth, and illustrate important aspects of evolution of this pathogen. For a long time, NetB was considered the sole crucial toxin in avian necrotic enteritis development in broilers. Loss of the entire netB‐encoding plasmid results in the loss of virulence of C. perfringens type G strains. Interestingly, complementation of this mutant strain with only netB toxin gene restores pathogenicity, although not to its full extent, showing that other netB‐associated genes present on the plasmid are required during pathogenesis (Zhou et al. 2017). Lepp et al. (2010) identified three highly conserved pathogenicity loci specific for type G strains (Figure 28.2). These genomic regions include NEloc‐1 (42 kb, plasmid, contains the netB and 36 additional genes), NEloc‐2 (11.2 kb, chromosome, contains 11 additional genes), and NEloc‐3 (5.6 kb, plasmid, contains five associated genes). In addition to these pathogenicity loci, over 400 variable genes (VR regions) were identified, including several chromosomal genes associated with bacterial host “fitness” such as iron uptake and nutrient acquisition as well as a pilus‐adhesive gene locus VR‐10B (Lepp et al. 2013). The involvement of some of the putative proteins during avian necrotic enteritis have been proven, for instance the plasmid‐encoded glycoconjugate binding zinc metalloprotease ZmpA (NEloc‐1, mucus degradation), and the related chromosomally encoded ZmpB, as well as the chromosomally encoded collagen binding adhesin CnaA, which is part of a chicken‐specific pilus VR‐10B genetic locus (Wade et al. 2016, 2020). Avian necrotic enteritis strains belong to at least two distinct clades, with clade 1 containing a necrotic enteritis‐specific pilus‐adhesion locus VR‐10B and largely falling into two subclades (Lacey et al. 2018). The second clade represents a broader range of strains that have acquired the NetB virulence plasmid but appear less well adapted to chickens (Lacey et al. 2018). Additional research is required to identify the importance of the other putative virulence factors during necrotic enteritis pathogenesis specifically, or disease pathogenesis for other C. perfringens associated diseases in general. Toxinotypes have been associated with specific human and animal diseases, indicating that toxin production profoundly influences the virulence of C. perfringens strains, although other host‐specific adaptations are also present. A list of both the well characterized and more speculative C. perfringens‐associated enteric diseases is given in Table 28.2. The common presence of C. perfringens in the intestine of animals, especially in young animals, means that the organism has likely erroneously been attributed as the cause of some intestinal diseases. Considerable further work is required to carefully describe both the disease processes and the genome sequences of the strains so that its role can be confirmed. Disease manifestations of the most prominent enteric C. perfringens‐associated animal diseases for each toxinotype are briefly discussed below. Figure 28.2 Genetic organization of necrotic enteritis‐specific loci in Clostridium perfringens type G strains. The genetic organization of (a) NELoc‐1, (b) NELoc‐2, and (c) NELoc‐3 is shown, each arrow representing a predicted gene and the total size given below each locus. Predicted functional annotations and locus tags are shown above and below each gene, respectively. Genes are color‐coded by their putative role based upon sequence analyses. The presence of NELoc‐2 and NELoc‐3 is characteristic of clade 1 strains of type G strains; clade 2 only possess NELoc‐1 in the pNetB virulence plasmid. Source: Based on Lepp et al. (2010) and Lacey et al. (2018). C. perfringens type A is the possible cause of several bovine gastrointestinal disorders, but is most convincingly recognized as a cause of necrohemorrhagic enteritis (formerly known as enterotoxemia) and to a lesser extent of abomasitis. Necrohemorrhagic enteritis affects calves held under intensive rearing conditions, resulting in sudden death despite overall good health. The disease occurs in both suckling calves and in veal calves (predominantly beef cattle breeds) around the ages of four and eight months, respectively. The susceptibility of breeds is higher in beef cattle breeds compared with dairy and mixed‐breed calves. The disease progresses rapidly, with sudden death only five hours after the first premonitory signs are observed. The enteritis is characterized by diffuse or localized small intestinal hemorrhage mostly situated in the jejunum, but occasionally the ileum, and less frequently the caecum, colon, and abomasum can be affected. The intestinal content is mostly liquid and bloody and limited to the diseased segment, suggesting a paralytic ileus. On a microscopic level, intestinal hemorrhages and cell necrosis can be observed from the tip of the villi to the base of the crypts. In the most advanced stage, necrosis is present in which the villus tips detach from the underlying viable tissues. The essential role of CPA has been confirmed (Goossens et al. 2017). Table 28.2 Enteric diseases associated with different recognized toxinotypes of Clostridium perfringens. C. perfringens type B infection causes dysentery in young lambs less than two weeks of age. The disease occurs uncommonly in Europe, South Africa, and the Middle East, but has not been described in America and Australia. Disease has been associated with nutrient spillover into the small intestine in lambs suckling heavily lactating ewes. Clinical signs associated to lamb dysentery are acute abdominal pain, a distended abdomen, and hemorrhagic diarrhea, followed by recumbency, coma, and eventually death, with a fatality rate of 100%. Disease is characterized by hemorrhages and ulcerations in the small intestine, congested and edematous lungs, a pale, congested, or friable liver, an enlarged and pulpy spleen, and pale, enlarged kidneys. Microscopically, there is multifocal to diffuse transmural hemorrhage of the small intestine with mucosal coagulation necrosis that extends into the muscularis and serosa. In addition to its effect on the gastrointestinal tract, neurological signs are common. The two main virulence factors associated to lamb dysentery are CPB and ETX. However, Falkow’s molecular Koch’s postulates have not been attempted to confirm this. The latter toxin could explain the observed neurotoxic effects (Uzal and Songer 2016). C. perfringens type C causes necrotizing enteritis and enterocolitis in an array of species, including humans. In lambs, the disease is referred to as “hemorrhagic enteritis,” in adult sheep as “struck,” and in young goats, calves, piglets, and foals as “necrotic enteritis.” In humans, “enteritis necroticans” is also sometimes called “pigbel” or “Darmbrand.” There is limited information on the prevalence of the disease, although it is uncommon. In all host species, the fatality rate is high, at 50–100%. Clinical signs are similar in young animals of most species, although interspecies and individual variations may occur. In peracute or acute cases, animals suffer from severe colic, lethargy, severe depression, and often bloody diarrhea. If the disease progresses more slowly, a more chronic form is observed, which is characterized by persistent diarrhea, weight loss, and dehydration. C. perfringens type C infection induces lesions in the small intestine, either jejunum or ileum in sheep and calves, or just the jejunum in piglets. The comprehensive hemorrhagic necrosis affects the mucosa, submucosa, and muscularis mucosa. As a result of serosal congestion and hemorrhage, the affected intestinal segment becomes dark. In acute cases, a brown, bloody fluid has been reported, containing fibrin clots and necrotic debris. In chronic cases in piglets, the intestinal fluid is mucoid and yellow. Molecular Koch’s postulates have been fulfilled for the CPB toxin in piglets (Diab 2016). C. perfringens type D causes enterotoxemia in small ruminants (sheep, goats, occasionally calves). The disease is sometimes also referred to as “overeating disease,” since sudden access to large amounts of feed in unaccustomed animals predisposes to disease development. In the past, the term “pulpy kidney” has been used, but is not recommended because of the subjectiveness of the description. This highly prevalent disease is notorious for its short clinical course and fatal outcome. Affected animals may develop neurologic disease since ETX absorbed from the intestine may be distributed throughout the body, causing generalized vascular tight junction degeneration and marked increased capillary permeability, including in the brain. Intracranial pressure rises, resulting in encephalomalacia. Type D‐induced enterotoxemia mainly affects young sheep and goat kids at the age of two weeks but also occurs in adult animals, notably in milking animals being pushed for production. In young animals, the disease mainly presents in an acute form, where animals die either shortly after showing neurological and respiratory clinical signs or suddenly without premonitory signs. In older sheep, both subacute and chronic enterotoxemia have been reported, characterized by neurological clinical signs such as blindness, ataxia, head pressing, and paraparesis. In adult goats, subacute enterotoxemia is characterized by diarrhea and colic, either with or without respiratory or neurological clinical signs. Animals die or recover within two to four days. Chronic enteritis may occur in older vaccinated goats, characterized by persistent, watery, and sometimes hemorrhagic diarrhea. Gross lesions in the gastrointestinal tract of animals with enterotoxemia are often limited to multifocal distention of the small intestine. In acutely affected sheep, the disease is characterized by pulmonary oedema with large amounts of froth in the trachea and lower airways, hydropericardium, hydrothorax, splenic and hepatic congestion, ascites and subendocranial, subepicardial, and serosal hemorrhages. In older sheep, gross brain lesions have been described, including cerebellar coning and focal symmetrical encephalomalacia. No such lesions are found in goats. In subacute and chronic cases of the disease in goats, colitis has been reported with mild to severe mesocolonic edema, colonic distention, hyperemia, hemorrhages, and necrosis of the colonic mucosa. In addition, hydropericardium, ascites, and pulmonary edema have been reported in the subacute form (Fernandez Miyakawa and Uzal 2003). The differing disease pathology in different hosts could be the result of host adaptation of the strains, although this hypothesis has not been confirmed to date. Type E C. perfringens is associated with often fatal but hemorrhagic enteritis in neonatal calves. Sporadic reports have described clinical cases in ostrich chicks, reindeer, goats, and lambs. Most aspects of the pathogenesis of type E enteric infections remain poorly characterized (Songer 2016). Type F strains contain the cpe gene and produce the CPE enterotoxin upon sporulation (Table 28.1; Rood et al. 2018). These strains cause food poisoning and non‐foodborne diarrheal disease in humans. The cpe gene is chromosomally encoded in the majority of type A food poisoning isolates but is plasmid borne in most human non‐foodborne and animal enteric disease isolates. The role of CPE‐producing strains in enteric disease in animals is poorly characterized and controversial (Busch et al. 2015; Uzal et al. 2018). Further work is required to identify whether, with the exception of NetF‐producing strains described below, type F strains are causally involved in enteric disease of animals. A subset of toxinotype F strains, which all encode the cpe gene on a separate tcp plasmid, are strains that encode two pore‐forming toxins related to CPB and NetB, designated NetE and NetF. Toxic activity is caused by NetF. These strains are associated with both necrotizing enterocolitis in foals and with canine acute hemorrhagic diarrhea syndrome in dogs. The strains all possess a tcp plasmid similar to those in Figure 28.1 but distinct from the cpe‐encoding plasmid. A small proportion possess a separate tcp plasmid with an additional related toxin gene named NetG (Mehdizadeh Gohari et al. 2021). NetF‐producing C. perfringens are associated with necrotizing enterocolitis in neonatal foals. The disease is very similar to type C infection and is usually a necrotic enteritis. Mortality is high with diseased foals dying within 12–24 hours after onset of the disease. Clinical signs are diarrhea, depression, dullness, colic, dehydration, and abdominal pain. Hematologically, either neutrophilia or neutropenia can be present, with increased fibrinogen. The disease is characterized by severe fibrinonecrotic enteritis, in some cases with intestinal mural emphysema. Focal mucosal ulceration can be present in both the small and large intestine. The disease is commonly acute but may develop into a severe, chronic, localized region of necrosis. Microscopically, the epithelia of the small intestine, large intestine, and gastric glands shows acute coagulative necrosis and are separated from underlying layers by a band of mostly neutrophilic infiltration. Typically, large numbers of Gram‐positive rods adhere to the necrotic intestinal mucosa (Gohari et al. 2015). NetF‐producing toxinotype F C. perfringens are also commonly associated with acute hemorrhagic diarrhea syndrome (AHDS), also known as canine hemorrhagic gastroenteritis or canine intestinal hemorrhage syndrome. This is a common cause of acute hemorrhagic diarrhea in dogs, although no single etiological agent of AHDS has been identified. AHDS may occur in dogs of any age or breed but typically occurs in small breed dogs. The disease is characterized by an acute onset of bloody foul‐smelling diarrhea with frequent vomiting. AHDS mostly has a self‐limiting, mild, though dramatic, course with only sporadically a fatal outcome. Clinical signs include depression, painful abdomen, and ileus. Hemoconcentration with increased packed‐cell volume, hemoglobin concentration, and red blood cell count may reflect the rapid fluid loss into the intestine. The mucosal and serosal surfaces of both small and large intestine are often diffusely hemorrhagic and necrotic Lesions can be present throughout the entire gastrointestinal tract, although the gastric mucosa is only sporadically involved. Microscopically, the villus epithelium shows coagulative necrosis and is separated from its underlying layers by a band of mostly neutrophilic infiltration. The recognition in recent years of the important role of NetF‐producing type F C. perfringens in many cases of AHDS has been a critical advance in understanding the basis of this disease, although more work is needed to understand its epidemiology and factors affecting host predisposition (Mehdizadeh Gohari et al. 2021). The causative agents of non‐haemorrhagic necrotic enteritis in broilers are C. perfringens type G strains producing the NetB toxin (Table 28.1
28
Enteric Clostridia
Introduction
Clostridium perfringens
Characteristics of the Organism
Pathogenic Types
Sources of Infections: Ecology, Evolution and Epidemiology
Toxinotype
Presence of toxin genes encoding
CPA
CPB
ETX
ITX
CPE
NetB
A
+
−
−
−
−
−
B
+
+
+
−
−
−
C
+
+
−
−
±
−
D
+
−
+
−
±
−
E
+
−
−
+
±
−
F
+
−
−
−
+
−
G
+
−
−
−
−
+
Plasmids in Evolution of Different Toxinotypes
Pathogenomics
Types of Disease and Pathologic Changes
Enteric Diseases Caused by Clostridium perfringens Type A
Bovine Necrohemorrhagic Enteritis
Toxinotype
Major toxin
Confirmed disease association
Possible but unproven association
A
CPA, alpha toxin
Necrohemorrhagic enteritis (“enterotoxemia”) in young cattle
Enteric diseases farmed ruminants (including abomasitis), poultry, horses, dogs, neonatal swine; necrohemorrhagic enteritis broilers; focal duodenal necrosis broilers; necrotic enteritis layer pullets
B
CPB, beta toxin
Lamb dysentery, sheep
Similar disease other ruminants, possibly foals; chronic enteritis sheep
C
CPB, ETX
Neonatal necrotic enteritis farmed ruminants, foals, swine; acute enterotoxemia adult sheep; enteritis necroticans in humans
Acute enterotoxemia sheep
D
ETX, epsilon toxin
Enterotoxemia farmed ruminants; enterocolitis adult goats
Yellow lamb disease
E
ITX, iota toxin
Severe gastroenteritis calves
Enteric disease rabbits, sheep
F
CPE, enterotoxin
Human food poisoning; human antibiotic‐associated and sporadic diarrhea. NetF‐positive, cpe‐positive strains necrotizing enterocolitis foals, canine acute hemorrhagic diarrheal syndrome
Food poisoning in animals?
G
NetB, necrotic enteritis B‐like toxin
Necrotic enteritis in poultry, other birds
Diseases Caused by Clostridium perfringens Type B
Lamb Dysentery
Diseases Caused by Clostridium perfringens Type C
Diseases Caused by Clostridium perfringens Type D
Diseases Caused by Clostridium perfringens Type E
Diseases Caused by Clostridium perfringens Type F
Diseases Caused by Toxinotype F Strains Producing NetF (With or Without NetG)
Diseases Caused by Clostridium perfringens Type G
Non‐Hemorrhagic Necrotic Enteritis in Broilers
Stay updated, free articles. Join our Telegram channel

Full access? Get Clinical Tree
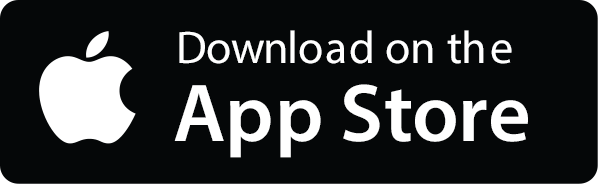
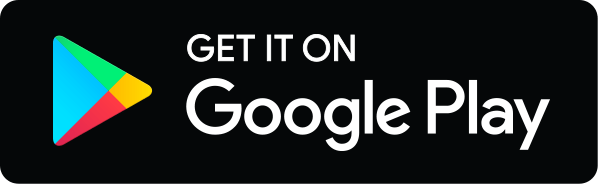