43 Ectoparasiticides
Ronald E. Baynes
Introduction
The focus of this chapter is to describe the pharmacology of veterinary drugs and pesticides currently approved to control important ectoparasites that adversely affect domestic animal health and production. These ectoparasites are primarily insects (fleas, flies, lice) and ascarines (ticks and mites). Effective control of these ectoparasites is not only critically important for the veterinary patients, but also from a public health perspective as several of the animal ectoparasites not only transmit disease between animals but they can also transmit major diseases to humans. Fleas are known vectors for tapeworms, Diplidium caninum, and humans can develop cat scratch disease when Bartonella henselae is transmitted from cats to humans via cat fleas. Flea allergy dermatitis is major concern for companion animals. These are amongst the most important reasons why flea control in companion animals is critical, and explains why annual expenditures to control fleas have totaled in excess of $2.0 Billion in the USA and Western Europe (Kramer and Mencke, 2001). Ticks can transmit Lyme disease, Rocky Mountain spotted fever, babesia in dogs and cattle, and heartwater in ruminants, to mention only a few. Mosquitoes can transmit West Nile virus to humans and heartworm diseases to pets, malaria to birds, rodents, and primates, and they also serve as biological vectors of viral encephalitides in horses. Flies not only transmit disease and cause damage to hides and coat of pets, their nuisance can result in decreased feed intake in livestock and thus reduced timely weight gain, resulting in economic loss to livestock farmers. Face flies are known to transmit the causative agent (Moraxella bovis) of pink eye or infectious bovine keratoconjunctivitis in cattle. Control of myiasis-causing flies can be significantly improved with effective ectoparasiticides.
The feeding behavior of ectoparasites differ and this may be critical if the drug of choice is strictly a repellent or adulticide. Adult fleas usually engorge themselves with a blood meal from the host with 5 minutes to 1 hour of infestation of the animal, with female fleas feeding for about 25 minute and males feeding for 11 minutes (Cadiergues et al., 2000). In order to prevent flea allergy dermatitis, an effective ectoparasiticide should be able to prevent this feeding behavior. Comparative efficacy studies suggest that organophosphates (OPs) and/or pyrethroids are more effective than the newer ectoparasiticides in preventing the flea from taking the first blood meal (Franc and Cadiergues, 1998). Ticks feed on a blood meal for a longer period (days) before it is completed. Under these conditions pathogens such as Borrelia burgdorferi, which causes Lyme disease, become well adapted to this long feeding period. However, with other tick-borne pathogens, such as Ehrlica and Rickettsia, transmission can occur within the first hours. For these reasons, the use of combination drugs (insecticide and repellent) will prevent ticks from attaching and feeding (Young et al., 2003). A good example of this desired combined efficacy is a drug formulation containing the repellent permethrin and the acaricide imidacloprid.
The ideal ectoparasiticide should not only be an effective repellent and adulticide, it must also be persistent in the blood or skin surface at therapeutic concentrations for a significantly long time, such as 1–3 months, so that there is adequate client compliance. Another challenge is that the formulation of an insecticide must be stable to sunlight and also water/shampooing. An excellent example of this is imidacloprid, which is photostable and persistent after shampooing compared to the early generation pyrethroids that were not very stable in sunlight. Some formulations, such as Frontline Plus®, contain the adulticide (fipronil) as well as an insect growth inhibitor (methoprene), because by the time pets are observed to be infested with fleas, the fleas would already have laid eggs. A final consideration in choice of therapy is whether to treat the environment as well as the animal. There appears to be a new paradigm within the last 10 years of treating the pet and not the environment (Rust, 2005).
Pharmaceutics of Topical Formulations
Many of the commercial topical ectoparasiticides used to treat domestic animals are formulated to be released from (i) liquid organic/aqueous formulations and/or (ii) synthetic polymers such as collars and ear tags. The rate of penetration through the skin or translocation across the skin surface is dependent on several critical pharmaceutical properties. Liquid formulations include pour-ons, topical sprays, and dips, which consist of the active ingredient and other (formerly called inert) ingredients that can modulate dermal pharmacokinetics. These other ingredients include mostly alcohols, oils, and spreading agents (Magnusson et al., 2001). The ear tags are composed of a solid organic polymeric macromolecule (e.g., polyvinyl chloride) and an active ingredient that are mixed with the tag and formed by injection molding. These devices are designed to slowly release the insecticide over a defined time period (e.g., Cutter Gold® last 5 months) as the animal moves its head, but it should be removed at the end of the fly season or before slaughter.
Transdermal Delivery
Topical application is the more common for administration of ectoparasiticides. An understanding of the dermal pharmacokinetics of these drug and pesticide formulations will further the understanding of drug efficacy and safety. The fundamentals of this topic are covered in Chapter 47. Many of these drugs and pesticides are very lipophilic with large molecular weights, and therefore it is not surprising that there is slow dermal absorption and low systemic bioavailability, as well as a large volume of distribution and long plasma and tissue half-lives. All of these are important factors when considering repeat applications and strategies to minimize the emergence of pest resistance, whether it is in a house, kennel, or pasture, and also to minimize drug residues in food-producing animals.
The advantages of transdermal delivery include ease of application to the animal’s coat and it avoids the problems of first-pass metabolism and also the gastrointestinal degradation often associated with oral administration. Some of the major disadvantages associated with this route of administration are pet owners sometimes overdose the animal with topical sprays or pour-on formulations, which often result in toxicoses in sensitive populations such as feline species and juveniles. Licking is part of the natural grooming and social behavior of many animal species, which can result in significant oral absorption and subsequently increased systemic exposure in the treated and also untreated animals that are part of a large livestock herd (Laffont et al., 2003). Application of pour-on products to food animals can result in significantly longer withdrawal times when compared to administration by oral or injection routes. This is most evident with ivermectin pour-on products, which cause significant retention of ivermectin in the skin layers and thus prolong release of the drug. For example, this results in a 45-day withdrawal time for the pour-on product versus 35 days for the subcutaneous route (KuKanich et al., 2005).
Routes of Dermal Absorption
There are five possible routes (Figure 43.1) for a drug or pesticide to diffuse as it partitions from the formulation on the skin surface through the stratum corneum and viable epidermis before entering the microcapillaries at the epidermal–dermal juncture. Many, if not all, of the ectoparasiticides diffuse through the intercellular matrix (intercellular route) in the stratum corneum and viable epidermis. This matrix may vary biochemically between animal species, but in all species it consist of varying proportions of fatty acids, ceramides, and cholesterol. The reader should also be aware that apparent epidermal thickness (Monteiro-Riviere et al., 1990) varies across animal species in the following order, thereby influencing the intercellular path length for the diffusing pesticide:


Figure 43.1 Pathways for drug and insecticide transport in mammalian skin. A, transcellular; B, intercellular; C, transfollicular; D, sweat pore; E, lateral diffusion.
The intracellular route and sweat pores are less likely routes for these lipophilic chemicals as they have more hydrophilic properties than the intercellular matrix.
Even though light microscopy examination of skin suggest that the hair follicle extends into the dermis and hypodermis, this is not really true. The hair follicle is really a deep invagination of the epidermis, and therefore if a drug travels down the hair follicle, it still has to cross the epidermis in order to gain access to the circulatory system. However, the presence of many hair follicles significantly increases the effective epidermal surface area, and thus increased flux, although the apparent surface area may be the same. This in part explains why care must be taken when administering pour-on ectoparasiticides in an extralabel manner across species. In essence, hair follicles and are arranged and distributed differently across animal species, and these differences can dictate transdermal delivery into the systemic circulation for pour-on products as well as translocation across the skin surface for topicals. The coat may contain primary and or secondary hair follicles. The former are large diameter and deep rooted into the dermis and associated with sebaceous and sweat glands. The secondary follicles are smaller in diameter, they are near the surface, and have sebaceous glands, but no sweat gland erector pili muscle. Horses and cattle have single hair follicles distributed evenly while pigs have single follicles grouped in clusters of four follicles. Dogs have primary and secondary follicles with as many as 15 hairs emerge from a single opening in the skin. Goats have primary follicles in groups of three, with three to six secondary follicles associated with each group. Sheep are bit more complex. They have hair-growing regions with mostly single follicles on face, distal legs region, and ears, and they have wool growing regions over most of their body, which can be defined as mostly compound follicles. A cluster will have three primary follicles and several secondary follicles.
Mechanisms of Action
Structures of many of the compounds to be discussed in this chapter are depicted in Table 43.1. Ectoparasiticides can have the following broad mechanisms of action that can affect the biology of the ectoparasite. More specific examples are summarized in Table 43.2 .
- Ectoparasite nervous system
- acetylcholinesterase (AChE) inhibitors
- Na+ channel blockers
- nicotinic acetylcholine receptor (nAChR) inhibitors
- γ amino butyric acid (GABA)/glutamate receptor Cl− channel inhibitors
- Ectoparasite repellant
- Insect growth and development.
Table 43.1 Chemical structures and physicochemical properties of common ectoparasiticides
Name | Chemical name (empirical formula) [molecular weight] Log P | Chemical structure |
Permethrin |
3-(2,2-dichloroethenyl)-2,2-dimethyl-cyclopropane-carboxylic acid (3-phenoxyphenylmethyl) ester (C21H20Cl2O3) [391.29] Log P = 6.10 |
![]() |
Fenthion |
Phosphorothioic acid O,O-dimethyl O-[3-methyl-4-(methylthio)phenyl] ester (C10H15O3PS2) [278.34] Log P = 4.84 |
![]() |
Carbaryl |
1-naphthalenol methylcarbamate (C12H11NO2) [201.22] Log P = 1.85 |
![]() |
Ivermectin |
22,23-dihydroavermectin B1 (C48H74O14) [875.10] |
![]() |
Milbemycin Oxime |
5-didehydromilbemycin (oxime derivative) 80% A4, 20% A3 (C31H43NO7) A3 [541.68] (C32H45NO7) A4 [555.71] |
![]() |
Fipronil |
5-amino-1-[2,6-dichloro-4-(trifluoro-methyl)phenyl]-4-[(trifluoromethyl)sulfinyl]-1H-pyrazole-3-carbonitrile (C12H4Cl2F6N4OS) [437.15] Log P = 4.0 |
![]() |
Imidacloprid |
1-[(6-chloro-3-pyridinyl)methyl]-4,5-dihydro-N-nitro-1H-imidazol-2-amine (C9H10ClN5O2) [255.66] Log P = 0.57 |
![]() |
Table 43.2 Mechanisms of action of ectoparasiticides against the parasite and signs of mammalian toxicity
Drug | Mechanism of action | Signs of toxicity |
Fipronil | Noncompetitively block passage of Cl ions through GABA- and glutamate-gated channels | Hyperactivity and convulsions related to GABA antagonism |
Imidacloprid | Competitive inhibition at postsynaptic nicotinic acetylcholine receptors (nAChR) leads to influx of Na ions | Nicotinic effects (tremors) |
Avermectins | Target glutamate-gated chloride channels |
CNS depression and ataxia Target GABA- and glycine-gated chloride ion channels |
Pyrethrins and pyrethroids | Target voltage-gated Na ion channels in axonal membrane |
Type 1 syndrome (tremors, hyperexcitability) Type 2 syndrome (salivation, weakness) |
Organophosphates and carbamates | Inhibition of acetylcholinesterase (AChE) | Muscarinic and nicotinic effects (miosis, tremors, depression) |
Amitraz | Inhibits monamine oxidase | Activates α2-adrenergic receptors (CNS depression) |
Insect growth regulators |
Falsely signals insect to remain immature stage Inhibition of chitin synthesis |
No known toxic signs |
Synergists | Inhibit cytochrome P450 | Can potentiate drugs and pesticides |
A good ectoparasiticide has a high efficacy against the ectoparasite and is not toxic to the host. Many of the OP and organochlorine pesticides are very effective and have a broad spectrum activity. However, because of their toxicity to mammals (Table 43.2 ), there are safer pesticides available for veterinary use, and fewer chemicals from these two classes are being used in veterinary medicine. For this reason, this chapter will not discuss the organochlorines especially as they are no longer approved for veterinary use. The newer ectoparasiticides such as the neonicotinoids (e.g., imidacloprid) are more selective for certain insects such as fleas, and very few other insects. However, the term broad spectrum may be misleading. Recall that ivermectin, which is often regarded as a broad-spectrum antiparasitic drug, is indeed highly effective against many nematodes and ectoparasites, but is without effect on cestodes and trematodes.
Resistance to Ectoparasiticides
Resistance has been widely reported for many popular ectoparasiticides such the avermectins, pyrethroids, and OPs. Resistance also tends to occur where there is intensive use of antiparasitic drugs, even on “well-managed” farms. There is some concern that as the newer insecticides such as the neonicotinoids become more frequently and inappropriately used, resistance can potentially emerge. However, there is little or no evidence of fleas developing resistance to the increasing and popular use of imidacloprid (Schroeder et al., 2003). Mechanisms of resistance may be related to decreased penetration, increased detoxification enzyme activity, and decreased sensitivity of the target site. These mechanisms have been more recently elucidated from a molecular basis using annotated insect genomes and recognizing that insect resistance is mediated by complex multigene enzyme systems. Pyrethroid resistance amongst insects has been associated with mutations in the sodium channel genes reducing the ability of the insecticide to bind to the sodium channel. Although resistance to imidacloprid has not been demonstrated in cat fleas, mutations in nicotinic acetylcholine receptors have been associated with some agronomic insects being resistant to this new class of insecticides (Liu et al., 2005).
Cross resistance for a specific target site is indeed very common within individual insecticide classes. However, cross-resistance across different target sites is a more severe consequence and limits insect management. Detoxification mechanisms are often the source of cross-resistance between insecticides that differ in their mechanisms of action. These detoxification enzymes include the carboxylesterases, cytochrome P450s, and glutathione-S-transferases, which are often implicated in pyrethroid and OP resistance. In a more recent observation, point mutations in the Rdl gene in a field population of cat fleas conferred cyclodiene resistance and cross-resistance to fipronil, which is one of the few new chemicals on the commercial market (Daborn et al., 2004).
Strategies that can prevent or delay resistance include: (i) appropriate selection of insecticides, (ii) insecticide rotations and mixtures, (iii) limited interactions with agricultural pesticides, and (iv) resistance monitoring. The latter is a major issue when it comes to treating fleas as there are no universally susceptible strains of cat flea known (Rust, 2005). However, the use of various genetic tools such as TaqMan-allele-specific amplification provide a promising method for assaying large populations of insects for insecticide resistance to the newer chemicals on the commercial market. With this in mind, the reader is encouraged to consult reviews (ffrench-Constant et al., 2004) on how the new genetic tools are addressing insecticide resistance.
Fipronil
Fipronil was first approved in 1996 for use in dogs and cats to treat for fleas and ticks. This chemical has been marketed as Frontline® Top Spot and Frontline® Plus for dogs, puppies, cats, and kittens, and Frontline® Spray Treatments for cats and dogs. The Plus product indicates that fipronil was formulated with methoprene, which is an insect growth regulator (IGR) (methoprene is discussed in more detail in Section Insect Growth Regulators). The TopSpot product contains 9.7% fipronil and the Spray contains 0.29%. A more recent fipronil formulation is Frontline® Tritak for dogs (9.8% fipronil, 8.8% methoprene, and 5.2% cyphenothrin) and Frontline® Tritak for cats (9.8% fipronil, 11% methoprene, and 15% etofenprox). Cyphenothrin and etofenprox are pyrethroid or pyrethroid like and are discussed in Section Pyrethrins and Synthetic Pyrethroids.
Chemistry
This insecticide is classified as a phenylpyrazole with the molecular formula: C12H4Cl2F6N4OS. Fipronil has a molecular weight of 437.15 and log P (n-octanol/water) of 4.0, and solubility of 2 mg/l in water and >10,000 mg/l in corn oil (Budavari, 1996). This high affinity for oils in the skin partly explains why dermal absorption is limited.
Pharmacokinetics
Topical application of the fipronil formulation between the shoulder blades is believed to result in surface translocation of the chemical over the entire body with significant deposition in sebum, sebaceous glands, and hair follicles. In effect, the sebaceous glands act as a reservoir, which continuously secretes firponil out of the hair follicle forming a layer on and in the stratum corneum and fur of the animal. Recognizing that 95% effective flea control requires 0.7 µg fipronil/g fur, as much as 4.5 µg/g and 1.6 µg/g have been detected in cats at 42 days and dogs at 56 days, respectively. Dermal absorption of 14C-fipronil was less than 1% of the applied dose for all doses tested (0.88–48 mg/rat) and up to 24 hours exposure (Cheng, 1995).
Mechanism of Action
Fipronil acts on the central nervous system where it appears to be primarily effective in noncompetitively blocking the passage of chloride ions through GABA-gated and glutamate-gated chloride channels in ectoparasites. The latter mechanism exits in ectoparasites, but not mammals, suggesting that there are multiple insect target sites for this drug. A study has demonstrated that fipronil and its metabolite, fipronil sulfone, are potent inhibitors at these sites (Zhao et al., 2005). In mammals, fipronil binding to the GABA receptor is also less effective, which also accounts for its wide margin of safety. Fipronil sulfone has a higher potency than fipronil in mammalian GABA receptors and simultaneous exposure to other drugs that potentiate formation of the metabolite could potentially be toxic to mammals in these scenarios. However, data from the literature is conflicting as to whether metabolic conversion to the sulfone derivative represents detoxification. For example, pretreatment of mice with a cytochrome P450 oxidase inhibitor, piperonyl butoxide, increased the toxicity of fipronil by as much as sevenfold (Hainzl and Casida, 1996). Prior to its veterinary approval, fipronil was and is still approved for use in plant agriculture; however, photochemical conversion can result in a desulfinylated photoproduct that is 10-fold more potent than firponil at the mammalian chloride channel. Whether this will be concern for livestock that forage on exposed pastures is still to be determined.
Efficacy
Fipronil as a topical spray is effective against adult fleas, all stages of brown dog ticks, American dog tick, lone star ticks, and deer ticks. This product is approved for dogs, cats, and puppies or kittens (>8 weeks), and can provide protection for up to 30 days. The manufacturers claim that although the Topical Spot On product can be effective against fleas for as long as 3 months they still recommend that it be applied every month. Fipronil (9.8%) formulated with 8.8% S-methoprene (dogs) or 11% S-methoprene (cats) (Frontline® Plus), an insect growth regulator, is not only an effective adulticide, it also kills flea eggs and flea larvae. While excellent ectoparasiticidal efficacy against fleas, ticks, and chewing lice has been proven, there has been some concern about its repellent effects against ticks and also ticks already on the animal (Denny, 2001; Young et al., 2003). Compared to some pyrethroids and some OPs, fipronil is also not effective in preventing fleas from biting and feeding within the first hour of infestation and prior to being killed (Franc and Cadiergues, 1998). Firponil is effective for the treatment and control of biting lice (Trichodectes canis) in canine infestations (Pollmeier et al., 2002, 2004). The label also claims that this drug can aid in the control of sarcoptic mange infestations. Note that Frontline® Tritak™ for dogs is different from that for cats in that different pyrethroids are used in the formulations and applicators should be aware of this difference (see discussion on pyrethroid esters and pyrethroid ethers later in Section Pyrethrins and Synthetic Pyrethroids).
Safety/Toxicity
Fipronil appears to have a wide margin of safety (rat dermal LD50 = 2000 mg/kg), and thus is regarded as a lower order of toxicity when used in veterinary applications. No adverse effects have been observed at five times the maximum dose rate in dogs and cats. However, slight skin or eye irritation have been observed after skin and eye exposure, respectively (Rhone Merieux, 1997). The oral and dermal LD50 in rats is 100 mg/kg and >2000 mg/kg, respectively (Budavari, 1996). Like the avermectins and organochlorines, fipronil’s mechanism of action involves GABA receptor antagonism. At toxic doses, it can cause hyperactivity, hyperexcitability, and convulsions, which are correlated with increased spontaneous nerve activity and generation of prolonged high-frequency discharges with nerve stimulation. These symptoms are associated with blockage of the neuronal inhibition or membrane hyperpolarization because these antagonist are also blocking chloride uptake. This in effect results in stabilization of the GABA-bound form of the chloride channel (Bloomquist, 2003).
There appears to be no reported interactions between firponil and approved companion animal drugs and ectoparasiticides. Animals exposed to large doses of firponil via accidental oral absorption are expected to display seizures, and neurological and hepatic function should be monitored (Hovda and Hooser, 2002). Extralabel use in young or small rabbits can cause seizures and death. Multigenerational rat studies have concluded that this drug is not mutagenic or teratogenic.
Neonicotinoids
Imidacloprid
Imidacloprid has been approved for use in crop agriculture as a systemic insecticide as well as for treating adult fleas on dogs and cats. Amongst the many imidacloprid products, it is available as Advantage® (9.1% imidacloprid) for adult fleas and Advantage® II (9.1% imidacloprid and 0.46% pyriproxyfen) to target adult and early life stages of fleas. It is also formulated at 8.8% w/v with permethrin (44% w/v) in K9 Advantix® II to treat for fleas as well as ticks. The latter is approved for use in cats and not dogs because cats do not tolerate permethrin as well as dogs. Advantage Multi® (10% imidacloprid and 2.5% moxidectin for dogs/1.0% for cats) is a once a month application approved for dogs and cats to treat for adult fleas, intestinal worms, and prevent heartworm disease as well as treat sarcoptic mange in dogs and control ear mites in cats.
Chemistry
Imidacloprid belongs to a relatively new class of synthetic insecticides known as the neonicotinoids. Imidacloprid (chloronicotinyls nitroguanidine) more specifically belongs to the chloronicotinyls that were first synthesized in 1985. This chemical was found to have 62 to more then 3000-fold greater insecticidal activity than the nicotinoids (e.g., nicotine), and was introduced into the commercial market in 1991. These chemicals are photostable, which ensures prolong residual efficacy, and they are moderately soluble in water (0.51 g/l). The log octanol water partition coefficient of imidacloprid is 0.57 and it has a molecular weight of 255.7. Unlike the nicotinoids, the neonicotinoids are not ionized at physiological pH. Neonicotnoids therefore more readily penetrate the CNS of insects than nicotinoids because the former has a greater hydrophobicity. This physicochemical difference in physiological conditions partly explains why the neonicotinoids have greater insecticidal activity than the nicotine-based insecticides.
Pharmacokinetics
Topical application of imidacloprid to the skin does not result in significant dermal absorption into the blood stream, but rather surface translocation aided by body movement results in whole-body coverage. Its efficacy is dependent on contact with the flea at the surface of the animal’s skin. Results from metabolism and toxicokinetics studies in other vertebrates suggest that if this chemical becomes systemic, then it is widely distributed to organs, but not to fatty tissues or the central nervous system (Sheets, 2001).
Mechanism of Action
At the nicotinic cholinergic synapse, acetylcholine (ACh) is normally released from the presynaptic membrane and by binding to the postsynaptic membrane this results in a conformational change in the receptor molecule that leads to activation and opening of the ion channel and subsequent influx of extracellular Na+ and efflux of K+. This results in change in membrane potential, and propagation of nerve impulse. Imidacloprid essentially mimics the effects of ACh by competitive inhibition at the postsynaptic nicotinic acetylcholine receptors (nAChR) and little or no effect on muscarinic acetylcholine receptors (Buckingham et al., 1997; Tomizawa and Casida, 2003). The former are widely and predominantly distributed in the neuropil regions of the CNS in insects, and imidacloprid is highly specific for nicotinic receptor subtypes unique to insects. However, instead of a depolarization of the postsynaptic neuron and transmission of the nerve impulse as normally seen with acetylcholine, imidacloprid causes a biphasic response. This consists of increased frequency of spontaneous discharge followed by a block in nerve pulse propagation along the neurons and then insect death (Schroeder and Flattum, 1984). Muscarinic receptors and other types of nicotinic receptors in mammals do not bind imidacloprid effectively, which accounts for its selectivity for insects (Kagabu, 1997). There is, therefore, no surprise that adverse effects are not observed when dogs were topically exposed to greater than the recommended dosage. Readers should be aware that although the neonicotinoids are selective, the nicotinoids are less selective and therefore more toxic to vertebrates than the neonicotinoids.
Efficacy
Compared to fipronil and selamectin, imidacloprid appears to be more readily taken up by the flea via body contact (Mehlhorn et al., 2001). This study demonstrated that imidacloprid killed larvae and adult fleas within 1 hour of exposure, while similar efficacy was achieved with the other two chemicals within 24 hours. It should be noted that none of these three chemicals had any repellent activity against fleas. Imidacloprid can kill 98–100% of adult fleas for at least 4 weeks on dogs and cats. This chemical has limited activity against ticks. Imidacloprid also stops fleas from biting within 3–5 minutes after application although the fleas do not die within this short time frame. Among the product claims for Advantage II® is the ability to kill fleas within 12 hours of application. This clearly is useful for preventing flea allergy dermatitis. Earlier studies demonstrated that shampooing or water exposure does not significantly reduce the efficacy of imidacloprid for up to 28 days (Cunningham et al., 1997).
In fall 2002, Bayer Corp. received registration from EPA for a topical ectoparasite K9 AdvantixTM, which is a topical insecticide formulated with imidacloprid and permethrin to repel and kill mosquitoes, ticks, and fleas on adult dogs and puppies 7 weeks of age and older for up to 4 weeks. The product’s efficacy is not affected by swimming or bathing activities. Imidacloprid acts on the postsynaptic nicotinic receptor by “locking” it in the open position thus hyperstimulating the neuron, and permethrin keeps open the Na+ ion exchange channels causing constant nerve impulses. Recall, that these would normally close after transmitting an impulse. There is, therefore, a synergistic interaction on the nervous system that is associated with an overwhelmed parasite nervous system, followed by rapid paralysis and death of the parasite. Due to their unique physiology and inability to metabolize certain compounds such as the pyrethroid (reduced glucuronidation activity in cats), this product must not be given to cats.
Safety/Toxicity
Clinical signs of toxicity are usually associated with nicotinic effects (e.g., tremors) and hepatic effects when dogs were exposed to a high dose of 41 mg/kg in the diet (Hovda and Hooser, 2002). However, except for one single reported case in cats (Godfrey, 1999),there are no published reports of imidocloprid causing systemic toxicity in domestic animal species following dermal exposure. The chemical does not appear to cause dermal irritation nor does it appear to cause dermal sensitization, although there are very rare anecdotal reports of adverse skin reactions. Imidacloprid does not appear to be a teratogen, reproductive toxicant, mutagen, or carcinogen (Sheets, 2001). The available products should not be given to puppies or kittens younger than 4 months.
Nitenpyram
Nitenpyram (CapstarTM) is in the same class as imidacloprid (i.e., chloronicotinyl), and therefore has the same mechanism of action. This is, however, not a topical application, but an oral adulticide that is used against fleas in dogs and cats.
Chemistry
Nitenpyram, like imidacloprid, belongs to the new class of synthetic insecticides known as the neonicotinoids. Nitenpyram has a molecular weight of 270.72, a log octanol water partition coefficient of −0.64, and water solubility of 840 g/l.
Pharmacokinetics
Nitenpyram is readily absorbed (bioavailability = 100%) in the gastrointestinal tract with the time to maximum blood concentration being 1.21 hours in dogs and 0.63 hours in cats. The Cmax is about 4.3 to 4.8 ppm in both dogs and cats, which is significantly greater than the blood concentration of 0.5–1.0 ppm nitenpyram required to kill 100% of the fleas. The half-life in dogs and cats is 2.8 hours and 7.7 hours, respectively, and it is primarily excreted in the urine.
Efficacy
The recommended minimum oral daily dosage is 1 mg/kg in tablet form with or without food and as often as once per day when fleas are seen on the animal. One dosage form (11 mg) is for cats and dogs up to 11 kg, and the other dosage form (57 mg) is for dogs between 11 and 57 kg. This chemical is also very effective against fleas that have become resistant to fipronil (Schenker et al., 2001). Based on the above pharmacokinetics, it is no surprise that fleas begin falling off of the coat of dogs and cats within 0.5 hours of administration of this drug (Rust et al., 2003; Rust, 2005). Efficacy reaches 98.6% in dogs and 98.4% in cats within 6 hours.
Safety/Toxicity
The acute oral LD50 in male rats is 1680 mg/kg, which suggest that this chemical is very safe. Ten times the recommended dose did not appear to cause adverse health effects in 7 to 8-month-old cats and 7-month-old dogs (Witte and Luempert, 2001). In the same study, administration of one, three, or five times the recommended daily dose for 6 months resulted in no adverse health effects in dogs and cats that were initially 8 weeks old. The drug is well tolerated for daily doses in puppies and kittens that are >4 weeks. No adverse reactions were observed when used with most other drugs or ectoparasiticides such as topical, carbaryl, fipronil, pyrethin, or even imidacloprid, which has a similar mechanism of action (Witte and Luempert, 2001). A transient itching period observed in dogs and cats within 1-2 hours after treatment with this product appears not to be associated with the chemical irritation, but attributed to fleas displaying increased trembling activity before dying and falling off the animal (Mahoney et al., 2001).
Spinosad
This neonicotinoid, which is a mixture of spinosyn A and spinosyn B (17 : 3 ratio), was approved in 2007 for once a month use in dogs, and in 2012 for cats (e.g., COMFORTIS® Chewable Tablets). Spinosad at 30 mg/kg dose is also formulated with milbemycin oxime (0.5 mg/kg) as TRIFEXIS®
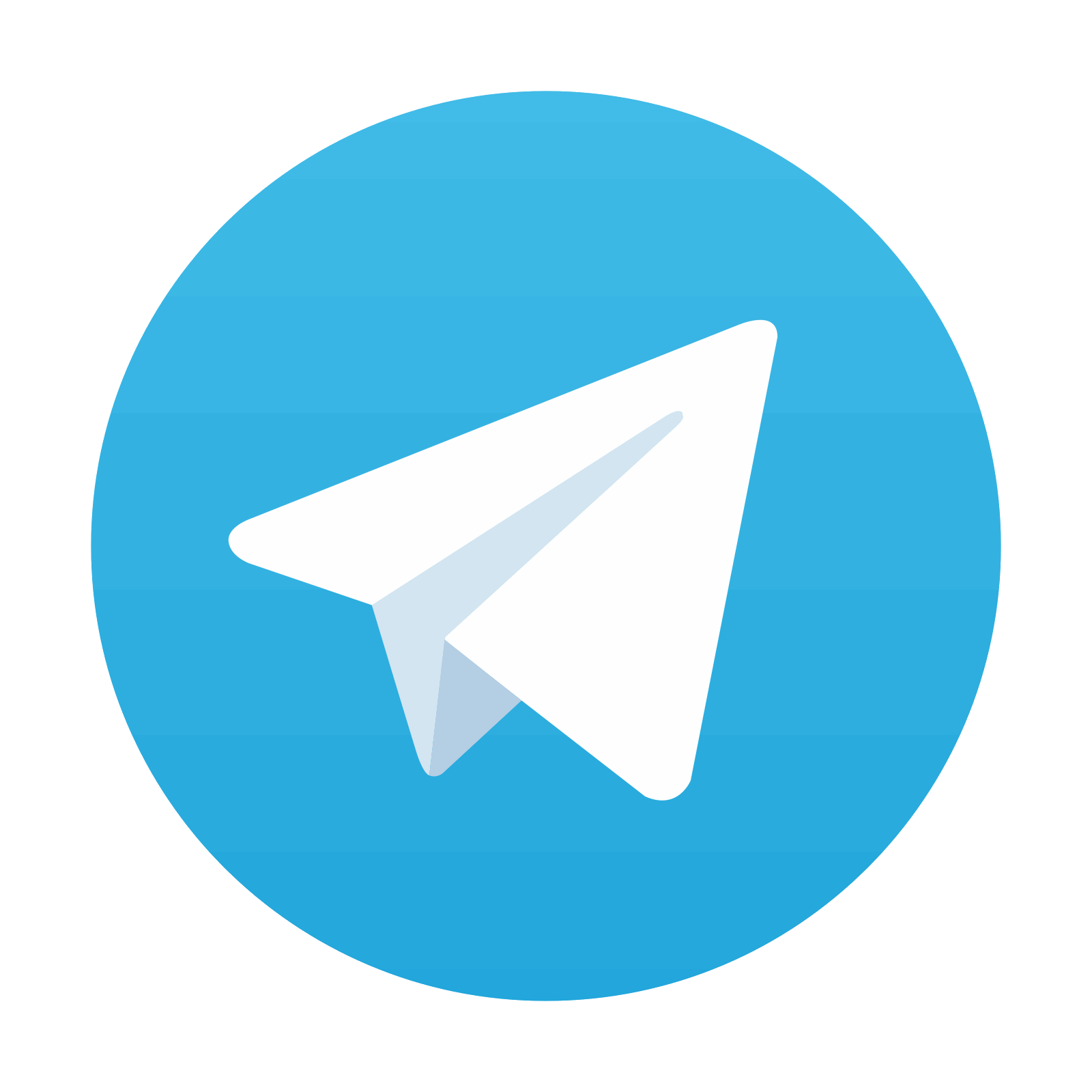
Stay updated, free articles. Join our Telegram channel

Full access? Get Clinical Tree
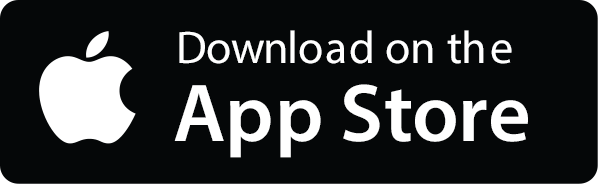
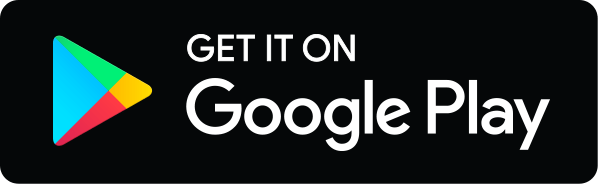