Disorders of the Neurologic System and Special Senses
The Neurologic Examination
After static observation, and with consideration for contagious and zoonotic conditions, the hands-on neurologic examination usually begins with assessment of the cranial nerves. In head-shy animals or those suspected to have rabies, it may be wiser to leave this until last. Olfaction (cranial nerve I [CNI]) is difficult to evaluate; abnormalities of this nerve often affect appetite. Vision (CNII + brain) is assessed by observing spontaneous obstacle avoidance by the animal or by leading the animal through obstacles, by stimulating the menace reflex (which also tests CNVII), and by observing the animal’s ability to track moving objects. Vision (CNII) and oculomotor nerve (CNIII) functions are further assessed by stimulating the direct and consensual pupillary light reflex. Camelids tend to have a sluggish response, similar to what is seen in ruminants. Eye position (CNIII, IV, and VI) is observed with the head in neutral position and in a few abnormal positions. Eye position often appears abnormal in animals in lateral recumbency or with the head retroflexed over the back but is found to be normal when the animal is restored to a normal, sternal position. Facial sensation (CNV) and motor function (CNVII) are assessed by stimulating various parts of the face with a probe and observing a twitch response, particularly by assessing the palpebral reflex. Facial motor function is further assessed by looking for lip, ear, or eyelid droop in a neutral position (Figure 38-1). CNVIII, the vestibular system, and, to a lesser degree, the cerebellum are assessed by identifying head tilting, circling or tendency to fall to one side, or nystagmus. Nerves innervating the tongue, larynx, and pharynx (CNIX, X, XI, and XII) are assessed for evidence of dysphagia or dyspnea. Tongue tone (CNXII) is difficult, but not impossible, to assess and is accomplished by gently grabbing the tip of the tongue and determining if the animal is able to pull it back. Unilateral loss of tongue tone is much harder to detect in camelids than in horses because of the relative immobility of the camelid tongue. Further evaluation of hearing and vision are discussed later in this chapter.
Figure 38-1 Ear drop and head tilt caused by dysfunction of cranial nerves VII and VIII, the most common cranial nerve dysfunctions in New World camelids.
Many camelids with neurologic disorders are recumbent or stand for only short periods, making evaluation of limb function difficult. For those that are ambulatory, it is possible to observe muscle strength, gait, and posture and to evaluate placement and conscious proprioception by obstacle avoidance and response to abnormal placements of the limbs. Ambulatory animals may be led in a straight line, over curbs, and subjected to gentle weaving or circling. Overly aggressive manipulators may easily make camelids appear neurologic. As with other species, the limbs are crossed, spread apart, and placed in a knuckling position to evaluate for corrective responses (Figure 38-2). This may be exceedingly frustrating in foot-shy camelids. Evidence of cerebellar disease, including hypermetria and intention tremors, is rare in camelids but easier to detect in the ambulatory camelid than in the recumbent one.
Spinal responses may likewise be difficult to evaluate. The panniculus response, which is stimulated by poking various parts of the trunk with a semisharp probe, and the limb withdraw reflex, which is simulated by applying pressure on or above the foot, have a voluntary component. Debilitated camelids or those demonstrating stoicism or a prey response may not react, in spite of intact neuromuscular pathways. The patellar reflex is involuntary and is best assessed with the camelid in lateral recumbency. The limb is elevated from the medial aspect of the distal femur with the stifle in relaxed flexion, and the patellar tendon is struck on its dorsal aspect. A sluggish response usually reflects lumbar spinal cord or cord root disease or a generalized neuromuscular disorder, and a hyperactive response suggests higher spinal disease.
Cerebrospinal Fluid Collection
Tapping at the lumbosacral site may be accomplished with light sedation and local anesthesia (Figure 38-3). In debilitated or recumbent camelids, butorphanol (0.022 milligrams per kilogram [mg/kg], intravenously or intramuscularly [IV or IM]) plus a few milliliters of subcutaneous local anesthetic at the site may be sufficient. In standing or struggling camelids, use of a restraint chute or stronger sedatives may be necessary.
It is common in healthy camelids and more common in sick ones for CSF to flow out of the needles under pressure (Figure 38-4). This may relate to a variety of factors but should not specifically be considered indicative of abnormal CSF pressure.
Normal CSF should be clear and colorless. Turbidity usually reflects an abnormally high cell count or protein concentration, and red discoloration reflects hemorrhage, which may be the result of the procedure or part of the pathologic process. A number of different sources have reported values from healthy camelids.1–4 The general consensus is that nucleated cell counts should not exceed 3 to 5 per microliter (µL) and microprotein concentration should not exceed 50 milligrams per milliliter (mg/mL). Unless blood contamination is present, the major nucleated cell types (up to 90% combined) should be small or large mononuclear cells. Creatine kinase (CK) activity should be less than 10 units per milliliter (units/mL), and the glucose concentration should be 60% to 80% of the blood value. The glucose difference increases with acute hyperglycemia because it takes approximately 4 hours for glucose to equilibrate across the blood–brain barrier. CSF sodium concentration should be similar to or slightly higher than plasma concentration. Acute increases or decreases in CSF sodium concentration usually reflect water shifts out of or into CSF.
Diagnostic Imaging
The possibilities of ultrasonographic examination are limited by the bone surrounding most of the CNS. Ultrasound probes may be placed on or around the eye, directed toward the foramen magnum, or be used to assess peripheral and cranial nerves where they course through soft tissue.
Cerebral Disorders
Cerebral disorders are relatively common in camelids. They may be primary or may be secondary to other disorders such as hepatic diseases or anything that disturbs electrolyte or fluid balance. Hypoglycemia and hyperosmolar disorder are covered in Chapter 41. Some cerebral diseases are focal and cause less pronounced signs, but most are diffuse and cause marked abnormalities. Milder signs include lethargy, inappetence, and eventually weight loss, which are signs that may also be caused by many other diseases. Thus, the pursuit of these general signs may eventually lead to CNS diagnoses. More specific signs include head or muscle tremor, ataxia, hyporeflexia, central blindness, behavior changes, head pressing, opisthotonus, ataxia, seizures, and coma, along with worsening weakness, obtundation, and possibly fever. Central blindness, an important indicator for diffuse cerebral disease, may be absent with focal disease and may be difficult to discern in animals that have existing retinal or oculomotor disease. It is characterized by evidence of blindness (bumping into obstacles, unawareness of approaching people, and an absent menace response), with retention of an adequate papillary light response.
Hydrocephalus and Hydranencephaly
Developmental brain abnormalities are present in a small percentage of crias and account for less than 2% of all congenital malformations.5 Some of these also have overt cranial malformation, but many have a grossly normal appearance from the outside. The severity of the signs depends on the degree of compromise of brain tissue. Signs are usually present at birth, although in rare cases of hydrocephalus, they may develop and worsen over the first 2 weeks of life or so. Affected crias are generally hypoactive and hyporeflexive. They appear weak and do not suckle readily. Thus, most suffer some degree of failure of passive transfer. Breathing may be normal or weak. Hydrocephalus has also been identified in an adult, and a cria with meningitis, but appears to be extremely rare.4,6
Polioencephalomalacia and Other Causes of Cerebral Necrosis
Polioencephalomalacia (cerebrocortical necrosis) in camelids is similar in some ways to the condition in ruminants but also appears to have some unique features.7 In all species, polioencephalomalacia is a histologic abnormality, which may be the common end point of a diverse set of conditions. In all cases, increased intracranial pressure (ICP) leading to pressure necrosis of the deep cerebral laminae, particularly those of the occipital lobe and parietal cortex, appears to be the final mechanism. The individual causes for that increase in pressure may vary, and they may occur at different frequencies in camelids and ruminants.
ICP usually increases with water accumulation. In ruminants, this is often the result of failure of the adenosine triphosphate (ATP)–dependent sodium–potassium pump. This pump exports three intracellular sodium molecules in exchange for two extracellular potassium molecules, thus promoting the outflow of intracellular water. Free water eventually passes from the cranial vault. Although some compounds may interfere with this pump directly, most cases of polioencephalomalacia in ruminants affect the pump indirectly by impairing ATP production. This has rapid consequences. The grey matter of the brain is metabolically very active and has a high energy demand (2% of body weight, but 20% to 30% of body glucose use). It is able to take up glucose in an insulin-independent fashion but must metabolize it aerobically (Krebs cycle) to obtain sufficient ATP.8 The brain stores very little glycogen and no oxygen and therefore relies on constant delivery of both compounds.
Much has been made of the role of the glycolytic pentose–phosphate shunt, which may augment other glycolytic pathways. Thiamine diphosphate is a rate-limiting co-factor in this shunt. Thiamine is made by microbes in the forestomach, and thus its production may be disturbed by illness, feed changes, or oral medications. Very little is stored, so constant production is required. In young crias or ruminants, ingestion of preformed thiamine is required and may be insufficient with some feeds. In juvenile and mature ruminants, outbreaks of polioencephalomalacia are often linked to changes to high-carbohydrate feeds or administration of oral antiparasitic agents. High-energy feeds lead to acidification of the rumen fluid, die-off of thiamine producers, and propagation of type II thiaminase-producing bacteria. Some deworming agents act as co-factors to other bacterial (type I) thiaminases, and some plants such as bracken fern, horsetail, and Nardoo fern contain thiaminases as well. Amprolium is an inactive thiamine analog, which blocks thiamine’s activity by competitive inhibition. Amprolium-induced polioencephalomalacia has been experimentally produced in camels, but only when the animals were fed a barley diet.9 A number of other minor and unlikely conditions may also decrease thiamine production or affect its function. Although some camelids with polioencephalomalacia, particularly those in scientific reports, have been exposed to some of these risk factors, namely, high-energy feeds, feed changes within 3 to 5 days, dewormers, or amprolium, many have not.9–13 Most reported cases involve adults, but preruminant crias account for half or more of our cases; whether this suggests dietary thiamine inadequacy or another mechanism is unknown.
Clinical signs are also more varied in camelids than is typical in ruminants. Central blindness is common but not universal. Obtundation, lethargy, anorexia, weakness, salivary loss, and recumbency are common but nonspecific. Head and body tremors, head pressing, opisthotonus, aimless wandering, hyperreflexia or hyporeflexia to external stimuli, ataxia, seizures, and coma are more specific to the neurologic system. Clinical pathology abnormalities are normally mild or reflect the primary disease such as hypoxemia or enteritis in neonates and forestomach acidosis in adults. Spinal fluid may contain a high number of vacuolated mononuclear cells and a mild increase in protein concentration. Diagnosis is mainly presumptive, based on signs, history, and CSF analysis. Postmortem identification of flattening and widening of gyri, necrosis of grey matter, and edema is confirmatory. In severe cases, the occipital lobes may have herniated under the tentorum cerebelli, and the hindbrain may have herniated into the foramen magnum. Fluorescence of brain sections under ultraviolet (UV) light was detected in one case but not another.11,13 Histologic lesions resemble those seen in ruminants.11
Cerebral Edema
Cerebral edema is a relatively common complication of IV fluid therapy in camelids because they are generally prone to hypoproteinemia and also hyperosmolar disorder. In addition, fluid requirements of camelids have not been worked out clearly, and aggressive regimens used successfully in other species may be too rapid for camelids. Signs are similar to those of hyperosmolar disorder and include lethargy, weakness, obtundation, fine head tremor that worsens to a coarse, whole-body tremor, base-wide stance, ataxia, seizures, coma, and death. Eyes and mucous membranes may also appear excessively moist. Because the signs are so similar to hyperosmolar disorder, history and clinical pathology data may be necessary to differentiate the disorder; camelids with cerebral edema would not specifically be expected to have high blood or CSF sodium concentrations. Increased CSF pressure may be indicative, but reference values have not been determined, and fluid from normal camelids often appears under pressure at the lumbosacral tap site. Cerebral edema is rare in camelids not being administered IV fluids. Pulmonary edema and respiratory signs may be present concurrently.
Toxic Neuropathies and Neurohepatopathies
The most widely suspected toxic neuropathy was the outbreak of suspected salinomycin-related feed contamination.14 Affected camelids eating a specific feed developed signs that included dyspnea, ataxia, recumbency, and seizures before dying. Blood evaluation demonstrated increases in muscle enzymes. On postmortem evaluation, heart and brain tissues appeared to be affected.
Overdosing lidocaine hydrochloride may lead to depression, behavior changes, ataxia, muscle tremors, opisthotonus, blindness, apnea, hypotensive shock, and seizures. The toxic threshold for camelids is not known but is likely to be similar to what is seen in ruminants (<5 mg/kg or <4 mL/kg of 2% solution). Tolazoline reversal of xylazine has also been associated with the development of neurologic signs, including evidence of anxiousness, hyperesthesia, and convulsions. Other possible signs include profuse salivation, diarrhea, gastrointestinal (GI) hypermotility, tachypnea, and hypotension.15 This toxicosis was associated with giving too much tolazoline too quickly and potentially after much of the xylazine had been metabolized. Giving smaller doses gradually is generally considered safe. Intracarotid injection of a variety of agents has also been associated with the acute development of central neurologic signs, including seizures, obtundation, and central blindness.16 For all these acute reactions to pharmaceuticals, general care is the rule: antiseizure medications as needed, cardiopulmonary support, and a low stress environment where self-trauma is unlikely. Some toxicoses are rapidly fatal, but if the animal maintains or regains cognizance within around 4 hours, the prognosis for general recovery is good; some neurologic deficits may remain and be considered permanent.
Cranial Trauma and Intracranial Hemorrhage
Head trauma is an unusual condition in camelids. Intracranial hemorrhage (ICH) is slightly more common and may occur independent of evidence of trauma (Figure 38-5). No real risk factors have been identified, but trauma from conflicts, encounters with other animals, projectiles, or motorized vehicles, or any other process leading to violent activity could potentially lead to concussive injury. Hemorrhage without trauma could be associated with a vascular accident or coagulopathy. Severe trauma may focally damage the brain directly through penetrating injuries or depression fractures. More commonly, it leads to ICH without fracturing the skull. Hemorrhage acts as a space-occupying lesion, and as it usually disseminates throughout the subdural space, it may lead to diffuse pressure on the cerebral cortex or the brainstem. Cerebral signs include anorexia, weakness, or obtundation in mildly affected animals, and ataxia, central blindness, head pressing, opisthotonus, or coma in more seriously affected animals.
Diagnosis may be based on history, especially if the event is witnessed, evidence of external trauma, CSF analysis, or imaging studies. CSF may contain numerous erythrocytes and often have high protein and neutrophil counts. Evidence of erythrophagocytosis helps differentiate pathologic hemorrhage from iatrogenic bleeding during the procedure but may not develop for the first 24 hours or so. Atlanto-occipital fluid may be revealing when lumbosacral fluid is not. Conventional radiography is helpful if the skull is fractured, if penetrating foreign bodies are present, or if gas has entered the cranial cavity. Cross-sectional studies, particularly MRI, may be used to identify abnormalities of brain tissue or the presence of subdural hemorrhage.
Bacterial Meningitis, Encephalitis, and Myelitis
Bacterial infections of the CNS are relatively common but are usually the result of systemic infection or of infection with opportunistic organisms, as opposed to infection with any particular neurotropic organism. The most commonly reported infection, and the most common cause of sepsis in general, is Escherichia coli. Salmonella, Enterococcus, Pasteurella, Streptococcus, Listeria, and a variety of other organisms have been recovered from the CNS.17–23 Most may also be recovered simultaneously from other sites, including blood, the liver, or the lungs. While neonatal sepsis accounts for many of these cases, adult sepsis is also an important disorder. Neonatal sepsis is often a complication of failure of passive transfer, an otherwise compromised neonate, or housing in a dirty environment. Adult sepsis is often the result of chemical, infectious, or parasitic GI damage. Immunodeficiencies may also play a role. Most of the organisms are opportunists. Salmonella and Streptococcus equi ssp. zooepidemicus are known primary pathogens. Listeria monocytogenes is another primary pathogen, and does appear to show some degree of neurotropism. It is also a cause of sepsis.
Treatment consists of supportive care, treatment of underlying disorders, preventing or controlling seizures, decreasing inflammation, and eliminating the infection. Treatment of underlying disorders may include GI protectants, antiparasitics, and plasma transfusion. Seizure control may involve diazepam (0.02–0.5 mg/kg, IV) or phenobarbital (15–20 mg/kg diluted, IV over 30 minutes for loading dose, followed by approximately 1 to 4 mg/kg, IV or PO, q12h). Flunixin meglumine (0.5–1.0 mg/kg. IV, q12-24h) is the most common antiinflammatory agent. Glucocorticoids, dimethyl sulfoxide (DMSO), and a variety of antimicrobials have been used. Given the variety of organisms isolated from camelids with meningoencephalitis, empirical choices should be broad-spectrum agents. With early or less inflammatory disease, selection of a lipid-soluble agent that penetrates the blood–brain barrier may be beneficial. With later or more inflammatory disease, blood–brain permeability increases, and most antimicrobials will reach the brain and spinal cord. Because of the general lack of efficient antimicrobial defense mechanisms in the brain, high concentrations of bactericidal antibiotics are preferred. Agents reported to be useful in other species when the blood–brain barrier is reasonably intact include penicillin, erythromycin, trimethoprim sulfas, and florfenicol.24,25 The choices broaden with meningeal inflammation and include ceftiofur.26
Viral Encephalitis
Herpesviral encephalitis has been described after natural infection and experimentally reproduced using a virus originally described as neurotropic equine herpesvirus 1 (EHV-1).27–29 The largest outbreak of natural disease occurred in a group of llamas and alpacas imported together from South America and housed for 6 months in proximity with zebra, gnus, and antelope. Signs developed in a number of the camelids in the herd over a 30-day period, and a separate alpaca developed signs simultaneously 31 days after leaving the herd and less than 2 months after the arrival of a new group of zebras. This suggests that an incubation period of at least 1 month is possible before signs develop. In experimentally infected llamas, signs developed 5 to 8 days after intranasal inoculation.28
The major clinical sign is blindness with dilated pupils and absent papillary light reflexes. This is associated with chorioretinitis, vitritis, and optic neuritis. In some cases, only the retrobulbar optic nerve is affected, resulting in a normal funduscopic examination. Fever occurs during the acute phase, up to 4 weeks before the onset of blindness. The minority of affected camelids also develop tremors, dysphagia, ataxia, head pressing, obtundation, head tilting, paralysis, or vestibular signs, including nystagmus and head tilting. Blood changes are mild. CSF was judged to be normal in the one outbreak.27 If present, abnormalities would presumably consist of mononuclear pleocytosis and an increase in protein.
Diagnosis in live camelids may be supported by identification of a high or rising serum or CSF antibody titer. Clinically normal herdmates may also have increasing titers. In dead camelids, eosinophilic intranuclear inclusion bodies, cerebral edema, nonsuppurative meningoencephalitis, and lymphocytic perivascular cuffing may be found in the brain. Virus isolation, electron microscopy, immunohistochemistry, and fluorescent antibody testing may all be used to confirm the role of herpesvirus.
Although the connection between EHV-1 infection and clinical signs is strong in this case, the incidence of clinical infection in camelids appears to be very low in spite of frequent contact between camelids and horses. We have seen this in a single llama with a previously diagnosed immunodeficiency. Considering that the virus in the experimental trial was derived from a dead alpaca from the original outbreak, it is likely that that particular strain of herpesvirus, potentially originally from a zebra, was particularly pathogenic in camelids. Recent evidence suggests that zebras may be the reservoir host for the newly described EHV-9, which is closely related to EHV-1 and has also been linked to fatal encephalitis in artiodactylids and other species.29 Because herpesvirus infection was one of the first specific diseases described in New World camelids in the United States, many early references recommend vaccination with killed equine vaccine. The infrequency of this disease should be considered when making vaccine recommendations.
West Nile virus infection in New World camelids was first noted in 2002 in the United States, soon after its introduction into the Western hemisphere and has since been reported in several areas.30–32 More recently, the disease has also been identified in camels in the Middle East. Currently, the disease may be found in most of the world except in South America and eastern Asia. It is chiefly a disease of birds and spread by mosquitoes. Waterfowl and corvid birds appear to be some of the most sensitive hosts, and mass deaths among birds during mosquito season are harbingers of an outbreak. When West Nile virus, a flavivirus, first moves into an area or when large populations of naïve birds migrate into an infected area during mosquito season, the amount of virus in birds and mosquitoes increases. Mosquitoes then carry the virus to other hosts, including camelids, horses, and humans. The majority of mammals seroconvert over a very short period. They are considered dead-end hosts, though blood transfusion, organ transplantation, and breast feeding have been linked to spread in humans. The likelihood of disease in mammalian hosts relates to their degree of immunity, genetic susceptibility, and the amount of challenge.
Treatment is mainly supportive. In addition to fluids, NSAIDs, steroids, DMSO, and mannitol have all been used to decrease brain inflammation. Plasma from camelids hyperimmunized against West Nile virus may provide specific immunotherapy, but experiences in other species suggest this must be administered very early in the course of disease for the highest efficacy.32
Vaccination may lower susceptibility. Trials using killed vaccines produced for horses have produced minimal side effects.33 However, up to 10% of inoculated camelids still have inadequate virus-neutralizing titers after the second shot, leading some to suggest a third dose during the initial series. Annual boosters at least 2 weeks before mosquito season are recommended. Fly control and housing camelids from before dusk until after dark may also decrease exposure.
Louping ill is a tick-borne flaviviral encephalitis.34,35 It mainly affects lambs but occasionally also affects grouse, cattle, and goats. Less commonly, pigs, deer, rodents, camelids, and humans may also be infected. Currently, it is thought to occur only in and near Scotland and in Dartmoor, although a third focus in Eastern Europe is surmised. Transmission is most common during tick season, and Ixodes ricinus is thought to be the most important infective host. In sheep, most infected animals clear the infection after a few days of fever and viremia, but others develop severe, fatal viral encephalitis. In camelids, most reports are from younger adults and adolescents, and immunologic naïveté to the virus may contribute to more severe signs. In other species, the virus is shed in many body secretions, including milk, and may spread through contact, but this has not been reported in camelids.
Clinical signs include anorexia, lethargy, head and neck tremors, ataxia, and posterior weakness progressing over a few days to recumbency or death. A hind-limb “goose-stepping” gait may be seen. Progression is fairly rapid over a few days. Difficulty swallowing was reported in one case.35 Ixodes ticks may be found on affected camelids or herdmates. Herd outbreaks, or sporadic occurrence on farms have been reported. Severity of disease may depend on immunity: in sheep, previous exposure gives long-lasting immunity and colostrum is protective. Naïve flocks may have fatality rates as high as 60%.
Treatment is entirely supportive. Antiinflammatory agents, as well as treating affected animals and herdmates for ticks, may be beneficial. Two out of five animals in two reports survived.34,35 For prevention, effective tick control and limiting animal exposure to tick habitats during active times of the year are recommended. Additionally, vaccines are available in endemic areas.
Eastern equine encephalitis virus is an alphavirus with a similar natural cycle to West Nile virus. Birds are the reservoir population, and spread occurs through mosquito bites. Aberrant infection has been reported in a variety of mammals, including horses and humans, and involves different species of mosquitoes. Mammals are considered dead-end hosts. Eastern equine encephalitis virus is found along the coasts of the Atlantic, the Great Lakes, and the Gulf of North America; the Caribbean; and the Atlantic coasts and adjacent riparian regions of Central and South America. Infection is sporadic in mammalian hosts and tends to occur in the middle of the optimal season for mosquitoes and bird infection (August through October on the East Coast of the United States). Camelids less than 1 year of age appear to be more susceptible than adults, potentially because of lower immunity but also potentially before their immature fleece leaves them more open to mosquito bites.36
Antemortem diagnosis is usually presumptive and is based on clinical signs, the presence of the disease in the area, and the appropriate season for mosquitoes. The presence of fever in the acute stages is helpful in distinguishing herpesviral or flaviviral encephalitis from many other causes of CNS signs. Serologic evidence is supportive but requires survival of the patient. Postmortem diagnosis may be accomplished by immunohistochemistry or PCR of brain tissue or isolation of virus from this tissue. Histopathologic changes include lymphocytic perivascular cuffing, gliosis, and sporadic neuronal necrosis. Encephalitis may be mild and multifocal and also tends to be more neutrophilic compared with other viral encephalitides. The white matter is largely spared. Some camelids also have spinal cord inflammation, but viral antigen has not been identified in the cord. Immunohistochemistry staining localizes the virus to the cytoplasm of neurons, astrocytes, oligodendrocytes, and occasionally neutrophils.36
Rabies in camelids is much like this disorder in other mammalian species.37–43 It occurs worldwide except for a number of pockets of exclusion, namely, the British Isles, Australia, New Zealand, Scandinavia, the Iberian peninsula, Italy, Greece and the southern Balkans, and Hawaii. Virus is present in the saliva of infected animals, and transmission most commonly occurs through animal bites causing skin breaks. Most of the cases in the United States have been reported in the southeastern states, where raccoons are the major wildlife reservoir. Raccoons act as the major wildlife reservoir along the Atlantic coast of North America, foxes are the major reservoir in Asia, Europe, coastal Alaska, southern Arizona, central Texas, and parts of northern New England. Skunks are the major reservoir throughout the central United States, and coyotes are important in southern Texas. Rabid bats are found in many areas but are a rare source of exposure for domestic animals except where vampire bats are found. In areas where domestic animal vaccination is common, camelid exposure usually occurs through a bite from an infected wild animal. In areas where vaccination is sporadic, domestic dogs represent an important source of exposure for camelids.38–40 Dog attacks are also commonly associated with multiple infected camelids, whereas other wild animal encounters rarely result in more than one case. Infected alpacas biting other herd members, contaminating the fleece with saliva, and causing skin breaks on the ear has been reported, and alpaca-to-alpaca transmission has been suspected.39 Attempts to experimentally infect alpacas through conjunctival inoculation did not result in rabies.
Clinical signs have been documented 15 to 90 days after a known exposure in alpacas and up to 6 months later in camels.38,39,41 In other species, incubation periods of over a year have been postulated. Hindlimb bites may first lead to signs of rear-end weakness and ataxia, frequently interpreted as lameness, and progress to ascending paralysis and central signs. Forelimb bites may also lead to lameness signs, but these are rapidly overshadowed by central signs. Bites on the face lead to the most rapid development of cerebral and brainstem signs, potentially within a few days. Central signs include behavior changes and functional changes. Behavior changes may be toward aggressiveness (furious form) or, more rarely, toward lethargy (dumb form). Aggressive signs include aimless charges, head shaking, head, neck, and body tremors, pruritus, attacks on penmates, handlers, and inanimate objects, self-mutilation, obsessive biting and chewing, rejection of offspring, falling, aggressive breeding behavior in males, repetitive alarm vocalization, and intolerance of the environment. Dumb signs include generalized weakness, lethargy, flaccid paresis of various muscles, and hyporeactivity. With either form, pharyngeal and GI function may be impaired, leading to ptyalism and pseudoptyalism, cud loss, inappropriate regurgitation, dysphagia, tenesmus, colic, and bloating. In camelids, incessant yawning has been described, which may represent compromised attempts to vocalize.41 Progression from first signs to coma and death appears to take approximately 6 to 8 days.38,44
Vaccination with killed vaccines devised for livestock produces titers and possibly protects camelids against clinical rabies.45 Although these products are not approved for camelids, their apparent efficacy and safety support their use in rabies-endemic areas. In camels, boosters every 6 to 8 months may be necessary.41 Some modified vaccines may be problematic; postvaccination complications were reported in over 10% of the vaccinates in one herd.39 These complications included unilateral or bilateral hindlimb paralysis 14 to 30 days after vaccination in adults, with possible quadriparesis and difficulty urinating and defecating. In 6- to 10-month-old crias, eating, urination, and defecation ceased, but paralytic signs were not observed. This incident occurred over 50 years ago, and the vaccine used was a Flury low-egg passage strain. These have been shown to retain residual virulence in some species, and alpacas may have been affected for that reason.46 Modified live vaccines generated by other methods may present less risk, but this outbreak emphasizes the need for safety testing before using products in large numbers of animals.
Rabies is zoonotic. Secondary potential human exposure has been documented, so prophylactic immunization is required, although no clinical disease has been reported.38,42 Concerns about transmission through spitting appear to be overblown, considering pharyngeal function is usually impaired in animals with salivary infection.
Bornavirus was originally detected in Germany and was long thought to be confined to central Europe. Recent evidence suggests that it may be found worldwide. It was also originally thought to cause disease mainly in sheep and horses, but it is now thought to infect a wide variety of mammals, including humans and birds. The possibility of natural infection throughout this broader host range and the importance of infection in individual species have yet to be defined. Some infected individuals develop clinical T-cell–mediated, nonsuppurative meningoencephalomyelitis, but others develop subtle to no signs. Clinical infections in camelids have been reported only in Germany: an outbreak in zoo llamas and alpacas, a single adult alpaca in a private herd of four, and another single adult alpaca that moved between regions for breeding purposes.47–49 Asymptomatic horses were long thought to be the reservoir hosts, but recent data implicate shrews, and other mammals or birds may prove to be important as well.
Clinical signs include lethargy, anorexia, weight loss, progressive hindlimb lameness, weakness, ataxia, recumbency, and seizures. Specific neurologic signs may be obscured by the general weakness and malaise. In one male, chronic lack of libido was noted. Progression occurs over several weeks, although the disease may progress quickly once overt neurologic signs are noted. Survival appears to be rare. A furious form has also been seen in other species, confounding differentiation from rabies, but this has not yet been identified in camelids. Possible premortem tests include blood or cerebrospinal serology and PCR for virus within peripheral blood monocytes. Postmortem examination of the brain reveals lymphocytic inflammation and perivascular cuffing. Intranuclear inclusion bodies (Joest-Degen bodies) may be seen in neurons, especially of the hippocampus, but often throughout the brain. Occasionally, diffuse, homogenous intracytoplasmic staining exists. The presence of Bornavirus antigen may be confirmed by immunohistochemistry of tissue sections or cell cultures inoculated with homogenates of the brain or CSF, or the virus may be detected by PCR or isolated from infected cell cultures.48,50 Testing for Bornavirus is not available everywhere, especially in nontraditional areas, so samples from suspect animals may have to be sent to laboratories that offer testing. Because Bornavirus may infect humans and because rabies is the most important differential diagnosis, appropriate precautions should be taken when handling suspect animals or samples. Bornavirus infection is reportable in many areas. Vaccination is practiced as a preventive measure in endemic regions.
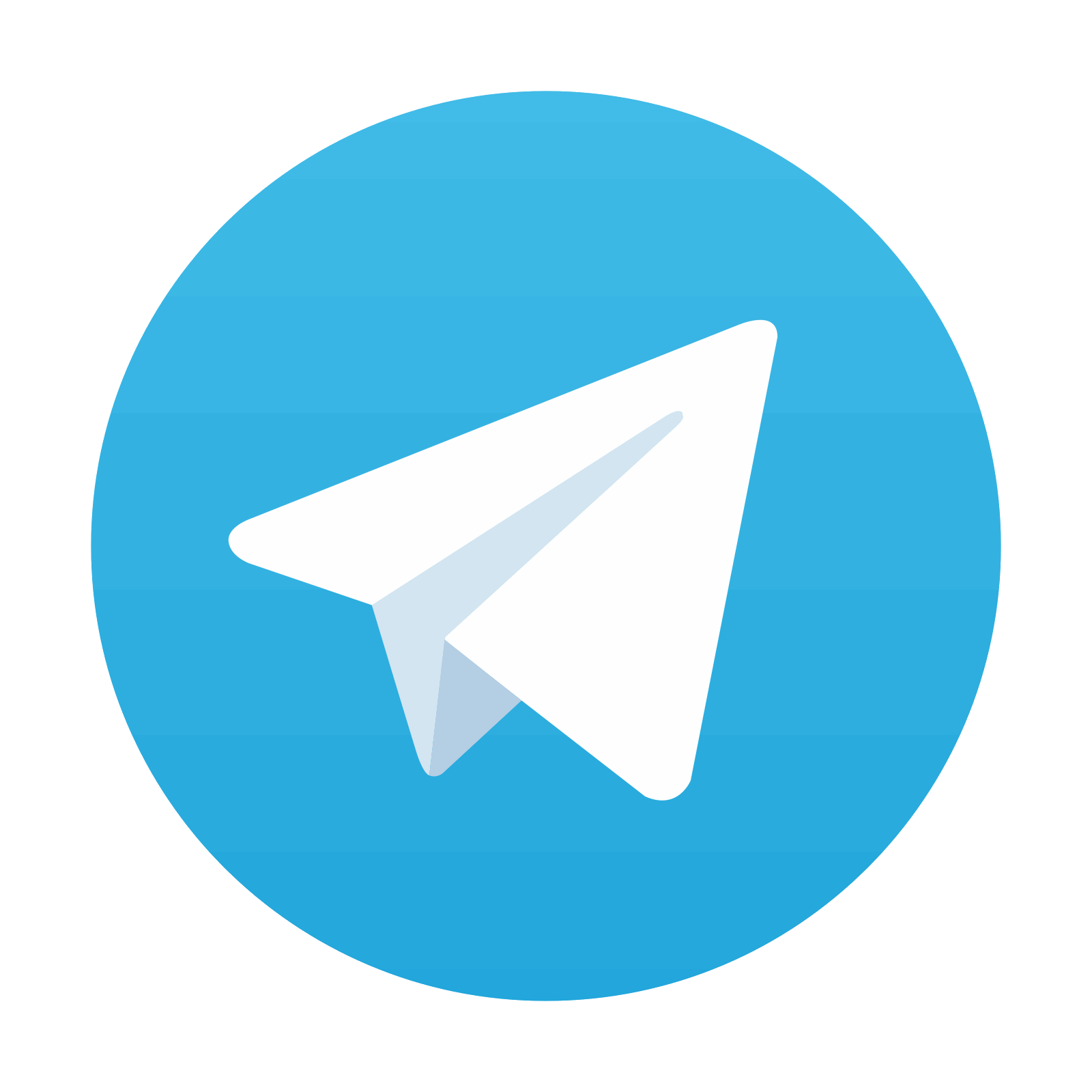
Stay updated, free articles. Join our Telegram channel

Full access? Get Clinical Tree
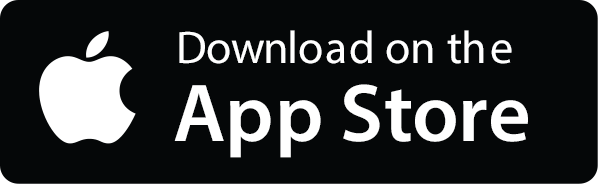
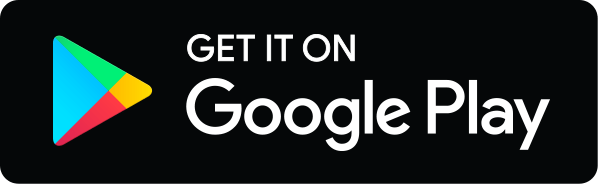