Chapter 9 Diseases of the Endocrine System
Hypothalamus
The hypothalamus is the portion of the rostral end of the diencephalon that lies below the thalamus at the base of the brain, around the third ventricle, extending from a plane immediately rostral to the optic chiasm to one immediately caudal to the mamillary bodies. Laterally its borders, somewhat ill-defined, roughly outline the optic tract.1 The hypothalamus is divided into a variety of nuclei that are critical to its major functions; this structure-function correlation should be kept in mind in considering diseases of the endocrine system.
The hypothalamus is the predominant center for the integration of many factors that influence control of key body functions. The proposal that the brain was somehow involved in controlling endocrine functions goes back to the 1930s.2,3 Neurohumoral control of the pituitary gland secretions and endocrine system was proposed in the 1950s, and the capillary system between the ventral hypothalamus and the pituitary gland was the suggested conduit for substances of hypothalamic origin that would act as releasers of each and every pituitary hormone.4,5 It was not until 1969 that the concept was finally validated by the isolation and structural characterization of one of these postulated messengers, thyrotropin-releasing factor (TRF), in extracts of ventral hypothalamic tissues.6,7 Ultimately, TRF and other hypothalamic messengers (Table 9-1) were demonstrated and quantified in blood obtained by catheterization of the hypothalamic-pituitary vessels.8
TABLE 9-1 Hypothalamic Messenger Hormones
Hormone | Abbreviation | Pituitary Target |
---|---|---|
Arginine vasopressin | AVP | Corticotropes |
Thyrotropin-releasing hormone | TRH | Thyrotropes |
Gonadotropin-releasing hormone | GnRH | Gonadotropes |
Somatostatin/growth hormone–inhibiting hormone | SS/GHIH | Somatotropes |
Growth hormone–releasing hormone | GHRH | Somatotropes |
Corticotropin-releasing hormone | CRH | Corticotropes |
Dopamine/prolactin-inhibiting hormone | PIH | Lactotropes |
Prolactin-releasing hormone | PrRP | Lactotropes |
Hypothalamic hormones regulate (i.e., stimulate or inhibit) the release of hormones from the anterior pituitary cells (Table 9-2) and are themselves released from the posterior pituitary gland (Table 9-3). Neuropeptides from the hypothalamus are transported to the anterior pituitary gland by the hypothalamic-hypophyseal portal circulation and to the posterior pituitary gland directly from hypothalamic nuclei by axoplasmic flow. Several different releasing and inhibiting factors in the hypothalamus are transmitted to the anterior pituitary gland by the portal vessels, each factor having more or less selective action on pituitary secretions. These hypothalamic releasing and inhibiting hormones are corticotropin-releasing hormone (CRH); thyrotropin-releasing hormone (TRH); growth hormone–releasing hormone (GRH); somatostatin (SS), also called growth hormone-inhibiting substance; gonadotropin-releasing hormone (GnRH); dopamine, also called prolactin-inhibiting hormone; and prolactin-releasing hormone (PrRH).9 The area from which hypothalamic releasing and inhibiting hormones are secreted is the median eminence of the hypothalamus. This region contains few nerve cell bodies, but many nerve endings are present in close proximity to the capillary loops from which the portal vessels originate. Cell bodies of the neurons that project to the external layer of the median eminence and secrete hypothalamic releasing and inhibiting hormones are organized into hypothalamic nuclei.
A wide range of physiologic signals, acting over diverse time frames, impinge on the hypothalamus. Regulatory systems in the hypothalamic-pituitary unit are established gradually throughout a sequence of morphologic and physiologic changes beginning in fetal life and ending in adulthood. The development of these axes is a complex process involving central as well as peripheral regulatory mechanisms.10 Some evidence suggests that the hypothalamic-pituitary axis in mammals is functional from early stages of fetal life.11 In the sheep fetus, maturation of the median eminence has been observed as early as days 48 to 67 of gestation.12 In small ruminants, the vascular connection between the hypothalamus and the pituitary is fully developed in the early stages of gestation (day 45), providing a functional link; presumably, hypothalamic factors may be transported directly by way of the portal vascular system to the pituitary gland to exert their stimulatory or inhibitory actions during gestation.12,13 However, the exact physiologic roles of some of the neuropeptides found in the embryo are not fully established.14 The individual components of the endocrine axes probably start their embryonic development independently of each other, and interactions between them are established only in the last stages of their maturation.15
Pituitary Gland
The pituitary gland is one of the most important endocrine glands in the vertebrate animal. The pituitary gland is an unpaired endocrine gland located at the base of the brain and is continuous with the ventral part of the hypothalamus. The reddish-gray round to ovoid gland lies on the inner surface of the base of the skull in the hypophyseal fossa of the sphenoid bone (sella turcica) between the optic chiasma and the mamillary bodies and consists of two major parts: the adenohypophysis and the neurohypophysis. Size and weight of the pituitary gland vary not only among species but also among breeds and age categories, and between genders. In sheep, the gland is approximately 13 to 15 mm in length and weighs 0.3 to 1.8 g on average.16 Its small size belies its importance and complexity, including its intricate embryology, structural heterogeneity, and functional diversity.
The anterior, intermediate, and posterior lobes of the pituitary gland act as three separate endocrine organs, each characterized by distinct cell populations, secretory products, and regulatory mechanisms. Originally described by Rathke in 1838,17 the pituitary originates from two distinct parts of the developing embryo. The adenohypophysis (pars distalis, pars intermedia, pars tuberalis) arises from the ectodermal saccule (Rathke’s pouch) of the roof of the primary oral cavity (stomodeum), and the neurohypophysis (pars nervosa or posterior pituitary gland) arises from the diencephalon.
Anterior Pituitary Gland
Structure, Function, and Hormones
The anterior pituitary gland is unique in the sense that the central nervous system, which controls this part of the hypophysis to a great extent, exerts its regulatory influence through a neurohumoral mechanism. Few if any nerve fibers pass to the anterior pituitary gland from the hypothalamus. Rather, the portal hypophyseal vessels form a direct vascular link between the hypothalamus and the anterior pituitary gland. Pituitary hormone–releasing substances are produced and released by the hypothalamic neurons into the special vascular system supplying the anterior pituitary gland. Arterial branches from the carotid arteries and circle of Willis form a network of fenestrated capillaries called the primary plexus on the ventral surface of the hypothalamus. Capillary loops also penetrate the median eminence. These capillaries drain into the sinusoidal portal hypophyseal vessels, which carry blood down the pituitary stalk to capillaries in the anterior pituitary gland. The portal system of blood vessels develops and is fully established by the end of the first trimester.18 The median eminence generally is defined as the portion of the ventral hypothalamus from which portal vessels arise. This region is outside of the blood-brain barrier. The anterior pituitary gland begins to function during the first trimester, and adrenocorticotropic hormone (ACTH), luteinizing hormone (LH), and follicle-stimulating hormone (FSH) are detected early in gestation. TRH-secreting cells develop early in the second trimester. Growth hormone (GH) and prolactin are synthesized in increasingly greater amounts during the second half of pregnancy.
The adenohypophysis constitutes approximately 80% of the pituitary gland19 and lies rostroventral to the neurohypophysis in ruminant species. The adenohypophysis can be divided into three subdivisions: the pars distalis (the major part of the adenohypophysis and referred to as the anterior pituitary gland for the remainder of this chapter), the pars intermedia (small division between the pars distalis and infundibular process), and the pars tuberalis (small dorsal extension of the pars distalis along the infundibular stem). It is a highly vascular structure that contains large numbers of different glandular cells capable of synthesizing and secreting various hormones—thyroid-stimulating hormone (TSH), ACTH, LH, FSH, prolactin, and GH. Some hormones from the anterior pituitary gland act on target endocrine glands, whereas others exert their influence without the intervention of other endocrine glands. Traditionally, cells of the adenohypophysis were divided on the basis of the affinity of the granules to various dyes with light microscopy (acidophils, basophils, and chromophobes). However, cells of the adenohypophysis are now distinguished according to their product(s) of synthesis and secretion.
The classical view of the anterior pituitary gland asserts that each cell secretes a single hormone (see Table 9-2).18 Evidence accumulated during the past 20+ years, however, points to the existence of subpopulations of multihormonal cells that may be involved in more than one neuroendocrine system.20,21 For instance, mammosomatropes contain both GH and prolactin.22,23 Growing evidence suggests that, in addition to the well-established LH surge, a concomitant surge in other pituitary hormones occurs: GH,24–26 prolactin,27–31 and TSH.32 One hypothesis that would explain such surges is the possibility of secretion of more than simply gonadotropins (LH and FSH) by gonadotropes. The proportion of gonadotropes, somatotropes, and lactotropes does not change during the estrous cycle in sheep.33 It has been demonstrated that GH co-localizes with LH in the ovine anterior pituitary gland21 (Figure 9-1). In contrast with the rat, in which up to 47% of the gonadotropes co-expressed GH messenger RNA (mRNA),34,35 this population was small in sheep, with at most only one tenth of the gonadotropes expressing immunoreactive GH.21 However, the detection of these LH- and GH-co-expressing cells was significantly influenced by the stage of the estrous cycle. The study also demonstrated that such co-expression in gonadotropes is not specific to GH, because both prolactin and TSH were detected within gonadotropes. Prolactin also was expressed within gonadotropes in the ovine anterior pituitary gland, but the stage of the estrous cycle had no influence on presence of these cells. Conversely, no gonadotropes co-localized with ACTH. Additional evidence indicates that receptors for hypothalamic releasing factors may not be restricted to a single pituitary cell type. Specifically, it has been shown in various species that GnRH binding is not restricted to gonadotropes.34–38
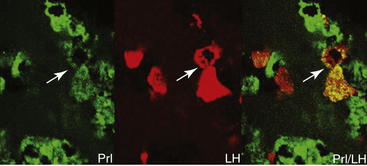
Figure 9-1 Micrographs of ovine pituitary gland from a luteal phase ewe after double immunofluorescence labeling. Prolactin-immunoreactive cells are green, with a clear double-labeled cell for βLH in red. Cells that were counted as double-labeled (arrowheads) are evident as yellow-orange cells in the panel on the right.21
The action of ACTH on the adrenal gland is necessary for the basal secretion of glucocorticoids and aldosterone, as well as for increased secretion of these hormones provoked by various stresses. Corticotropes, which synthesize and secrete ACTH, β-lipotropin, and pro-opiomelanocortin, account for another 15% to 20% of anterior pituitary gland cells.22 The release of ACTH is stimulated by two hypothalamic neuropeptides: CRH and arginine vasopressin (AVP). Although CRH is the most potent ACTH secretagogue in rats and humans, AVP is a more potent secretagogue than CRH in sheep and cattle.39–41 Also, with increases in audiovisual stress, the concentrations of AVP have been shown to increase more than those of CRH in the pituitary portal circulation of sheep.42 Physical injury, stress, hemorrhage, and other physiologic challenges produce afferent impulses that converge on the hypothalamus leading to increased CRH, AVP, and ACTH output. Conversely, glucocorticoids themselves block ACTH secretion through feedback inhibition exerted at the hypothalamic and pituitary levels.43 Engler and co-workers42 reported baseline (20 to 50 pmol/L) and stress-induced (as much as 200 pmol/L) ACTH parameters in sheep.
As expected, GH is obligatory for normal growth and development in young animals. However, it also influences fiber production and processes important for reproduction (e.g., steroidogenesis, gametogenesis, lactation) in adults. Of all of the hormones produced by the anterior pituitary gland, GH is the most abundant. The pituitary gland contains an amount of GH that is 20 to 40 times greater than that of ACTH and 50 to 100 times greater than that of prolactin.20 GH is synthesized in somatotrope cells in the anterior pituitary as a 191-amino-acid peptide in ruminants.44,45 In the classical view, GH was thought to be exclusively produced and secreted by somatotrope cells of the anterior pituitary.46 It is now accepted, however, that GH is produced by other cell types within the anterior pituitary gland.22,23 Moreover, sites of extrapituitary production of GH exist wherein this hormone exerts autocrine and paracrine actions.47 GH is secreted in episodes or pulses in all species studied to date, including small ruminants.48,49 The pattern of GH secretion varies depending on species, photoperiod, and metabolic signals, and it is sexually dimorphic.9,50 Hypothalamic control of GH secretion in mammals is a classic paradigm of the “dual control” system of pituitary hormone secretion: Two hypothalamic peptides with opposing roles, GRH and SS, directly regulate GH secretion from the anterior pituitary gland.9,51 GRH stimulates whereas SS inhibits GH secretion by somatotropes. Episodic GH secretion can be altered by diverse factors from the central nervous system, the pituitary gland, or factors from peripheral tissues.9 In addition to direct activation of various genes, GH mediates to the production of somatomedins. The best characterized somatomedins, insulin-like growth factors I (IGF-I) and II (IFG-II), mediate many actions of GH.
Reproduction in mammals depends on synthesis and secretion of gonadotropins from the anterior pituitary gland. Gonadotropins, FSH and LH, are synthesized and secreted by cells in the anterior pituitary gland (gonadotropes). Gonadotropins control steroidogenesis and gametogenesis in males and females and are members of the glycoprotein hormone family that also includes the pituitary hormone TSH and chorionic gonadotropins. Members of this glycoprotein hormone family are heterodimers, meaning that they are composed of two nonidentical subunits designated α and β. The pituitary glycoprotein hormones (FSH, LH, and TSH) share a common α-subunit identical in structure. However, the β-subunits are unique to each gonadotropin and confer biologic and immunologic specificity to each hormone. A single gene for the α-subunit as well as the β-subunit for LH and FSH has been identified.52 The common α-subunit combines with a different hormone-specific β-subunit in a manner that produces a unique tertiary configuration permitting efficient interaction with the hormone-receptor system in target cells. Gonadotropes, which secrete FSH and LH, make up 10% to 15% of anterior pituitary cells.22 Both synthesis and secretion of LH and FSH are regulated primarily by the central nervous system through the neurosecretion of GnRH and kisspeptin.53,54
Small ruminants exhibit seasonality of breeding activity, and although environmental temperature, nutritional status, social interactions, lambing/kidding date, and lactation period are modulators of this seasonality, photoperiod is the determinant factor. Melatonin, through its duration of nocturnal secretion, is the hormone responsible for translation of the day length information to the reproductive axis by changing the sensitivity of the GnRH pulse generator with consequent modification on the pulsatile secretion of LH. The exact site of action of melatonin within the central nervous system is still controversial and requires further research. Thyroid hormones also have an important role in seasonal reproduction, but the site, mechanisms of action, and integration in the current neuroendocrine model of photoperiodic control of seasonal reproduction need to be further elucidated. The first evidence of the involvement of thyroid hormones in seasonal reproduction of sheep was provided by Nicholls and colleagues,55 who found that ewes thyroidectomized in late anestrus entered normally into the breeding season but continued to exhibit regular estrous cycles throughout the subsequent anestrous season, remaining in this condition for more than 1 year.
Prolactin is a hormone with an important role in functions of lactation and reproduction i.e., it is both lactogenic and luteotropic).56 Lactotropes, which secrete prolactin, account for 10% to 25% of cells within the anterior pituitary gland.22 Prolactin is similar in structure to GH, with a comparable half-life.57 Prolactin, unlike other pituitary hormones, is controlled mainly by inhibitory factors originating from the hypothalamus, the most important of which is dopamine. It is well known that dopamine inhibits prolactin release,20 and in sheep, peripheral administration of bromocriptine, a dopamine D2 receptor agonist, abolishes the prolactin surge normally associated with the LH surge.58 In photoperiodic seasonal breeders such as sheep and goats, seasonal hormonal variation occurs in prolactin concentration.59 The highest circulating prolactin levels (200 to 800 ng/mL) coincide with long days (anestrous season), and the lowest levels (less than 40 ng/mL) coincide with short days (breeding season).60–62 However, during the breeding season at the time of the LH surge, a concomitant prolactin surge (up to 800 ng/mL) has been reported.27,28 Melatonin, rather than dopamine, controls the seasonal secretions of prolactin, independent of input from the hypothalamus.63 Studies in rats have suggested that prolactin-releasing peptide (PrRP) may play a role in the generation of prolactin surges.64 In ewes, however, PrRP is not released in the hypophyseal portal circulation at the time of the prolactin surge.27 Alternatively, melatonin is thought to exert its effects on the anterior pituitary gland via PrRP in the pars tuberalis.65,66 Because high prolactin levels normally are associated with ovarian inactivity, it has been suggested that the seasonally high prolactin concentrations may be responsible for seasonal impairment of reproductive function in sheep.67
Thyroid function is controlled by the TSH from the anterior pituitary gland. The secretion of this tropic hormone is in turn regulated in part by TRH from the hypothalamus and is subject to negative feedback control by circulating levels of thyroid hormones acting on the anterior pituitary gland and the hypothalamus. Thyrotropes, which secrete TSH, account for only 3% to 5% of cells of the anterior pituitary gland and are stimulated by TRH from the hypothalamus.68 TSH is a glycoprotein that is made up of two subunits designated α and β. TSH-α is identical in structure to α-subunits of LH, FSH, and human chorionic gonadotropin (hCG)-α. The functional specificity of TSH is conferred by the β-subunit. The principal hormones secreted by the thyroid gland in response to stimulation by TSH are thyroxine (T4) and triiodothyronine (T3). The negative feedback effect of thyroid hormones on TSH secretion is exerted in part at the hypothalamic level, but it also is due in large part to an effect on the pituitary, because T4 and T3 block the increase in TSH secretion produced by TRH. Therefore, in the absence of adequate thyroid hormones, excessive TSH is released, resulting in enlargement in the thyroid gland (goiter). Exposure to cold temperature also affects thyroid function.69–74
Posterior Pituitary Gland
Structure, Function, and Hormones
Release of AVP, also known as antidiuretic hormone (ADH), is triggered by stimulation of osmoreceptors in the ventrolateral medulla in conditions of hyperosmolarity, hypovolemia, and hypotension. These specialized neurons directly stimulate hormone release from the posterior pituitary by way of catecholaminergic A1 fibers; however, other neurotransmitters, including neuropeptide Y, also play a role in signaling hormone release. Vasopressin acts in the distal collecting tubule of the kidney to increase water retention, decreasing blood osmolarity and increasing urine concentration, by stimulating the insertion of increased numbers of aquaporin 2 in the apical membrane (short-term increase) as well as increasing aquaporin 2 gene expression (long-term change).75 Increased reabsorption of sodium chloride in the ascending loop of Henle, facilitation of aldosterone-stimulated sodium reabsorption, and increased permeability to urea in the distal collecting duct are also mediated in the kidney for an overall increase in urine concentration and antidiuretic effect. In addition to its renal effects, AVP acts directly on vascular smooth muscle to cause peripheral vasoconstriction; however, this constriction does not have an appreciable effect on total blood pressure, because the action of AVP on the postrema of the brain results in an overall decrease in cardiac output.18 Release of AVP decreases as blood pressure and volume increase and osmolarity falls to normal levels. Plasma vasopressin levels vary with hydration status. In sheep, reported levels change from 4.4 ± 0.6 pg/mL to 16.8 ± 1.0 pg/mL in hydrated and dehydrated states, respectively.76 Reported plasma AVP levels in goats allowed free access to water are 1.8 ± 2.9 pmol/L, but these can increase to 19.9 ± 9.4 pmol/L with severe dehydration.77
Diabetes insipidus (DI) results from failure of the kidney to concentrate urine appropriately as a result of impairment of the AVP mechanism. This condition can be classified as central or neurogenic, resulting from dysfunction in the neurohypophysis, or nephrogenic, secondary to inadequate renal response. Although ADH measurement has been described, diagnosis of DI relies on a water deprivation test.78 In this test, water is withheld and urine specimens are collected serially; urine specific gravity fails to increase in affected animals. Administration of exogenous AVP can then differentiate neurogenic from nephrogenic DI: Animals with the central form will respond by increasing urine concentration. Because the test requires that the patient become significantly dehydrated, renal disease should be ruled out beforehand, and patient weight should be monitored. Additionally, it is contraindicated in animals that are already dehydrated, azotemic, or hypercalcemic.78 Central DI was the suspected cause of polyuria and polydipsia in a 7-month-old Suffolk ram and in a 4.5-year-old Black Brown Mountain ram.79,80 Water deprivation testing and response to AVP administration confirmed the diagnosis in the former case; in the latter case, the test was initiated in an effort to prove this hypothesis, but clinical deterioration required euthanasia before its conclusion. In both cases, the DI was believed to be secondary to compression of the neurohypophysis, from external pressure and by a chromophobe adenocarcinoma of the adenohypophysis, respectively.
Syndrome of inappropriate antidiuretic hormone secretion (SIADH), in which AVP is overexpressed, is rare in all species.81–83 It has been experimentally modeled in sheep and goats with use of radiofrequency lesioning in the septal and preoptic regions and administration of exogenous AVP84,85; no naturally occurring cases have been reported thus far.
Oxytocin, the other hormone product of the neurohypophysis, is released in response to stimulation of sensory neurons with distention of the reproductive tract or manipulation of the mammary glands. Afferent impulses generated by milking or suckling of offspring travel to the supraoptic and paraventricular nuclei to trigger oxytocin release. Goats also show discriminatory release of oxytocin only when nursing their own kids; this release appears to be mediated by olfactory signals.86 The hormone acts directly on the myoepithelial cells of the mammary ducts to cause milk ejection and on those of the reproductive tract to cause smooth muscle contraction. Adrenergic stimulation inhibits the milk ejection reflex; therefore stress and the resultant sympathetic arousal can prevent milk ejection. The direct activation of smooth muscle by oxytocin also may play a role in parturition, passage of sperm into the uterine tubes, and propulsion of sperm into the urethra before ejaculation.18 The uterine contraction resulting from oxytocin stimulation has facilitated the wide pharmacologic application of this agent as an ecbolic for treatment of a variety of reproductive disorders (see Chapter 8).
Of interest, prestimulation of dairy goats causes earlier release of oxytocin but does not enhance milk flow rate or overall yield87; this effect is in contrast with findings in cattle and probably is attributable to larger cisternal milk volume. AVP also may have a role in milk ejection, although its exact physiologic mechanism has not been elucidated. The vasopressin V1 receptor is present on myoepithelial cells,88 and infusion of goats with this hormone results in increased milk flow and milk fat content.89 Furthermore, suckling results in measurable increases in both blood oxytocin and AVP levels, although hand milking elicits changes in neither.90
Pituitary Abnormalities
The most common pituitary tumors in domestic animals are found in dogs and horses, and reports in ruminants are very rare, especially in sheep and goats. A majority of pituitary abnormalities described in small ruminants are adenomas,91–95 but more recently, adenocarcinomas have been reported as well.96,97
Pituitary Adenoma
Acidophilic adenomas of the anterior pituitary gland have been reported in both sheep91,93,94 and goats.92,95 In domestic animals, pituitary tumors usually grow by expansion. Dogs and horses do not have a restrictive diaphragma sellae around the infundibular stalk, and pituitary tumors in these species often grow toward and into the hypothalamus.98 Such tumor growth is not observed in other species of domestic animals (including small ruminants) that have a restrictive diaphragma sellae around the infundibular stalk, in which most primary pituitary tumors remain extradural. Pituitary gland adenomas generally compress adjacent pituitary tissue in sheep93,94 and goats.92,95 Pituitary adenomas also can protrude dorsally toward the optic chiasm91 and erode the surrounding sphenoid bone ventrally91,92 (Figure 9-2). Acidophil-type cells generally produce GH and prolactin, both of which are lactogenic.23 Some sheep99 and goats95 with pituitary adenomas exhibit inappropriate lactation. Similar acidophilic adenomas have been reported in other species in association with abnormal mammary development,100 and anterior pituitary adenoma should be considered in the differential diagnosis for ruminants with inappropriate lactation syndrome (ILS). However, no definitive antemortem diagnostic test is currently available for this condition. Several other underlying pathologic conditions have been reported in does with ILS. Two does with ILS had concomitant pheochromocytoma and cystic endometrial hyperplasia.95 One of these animals also had thyroid and follicular ovarian cysts.95
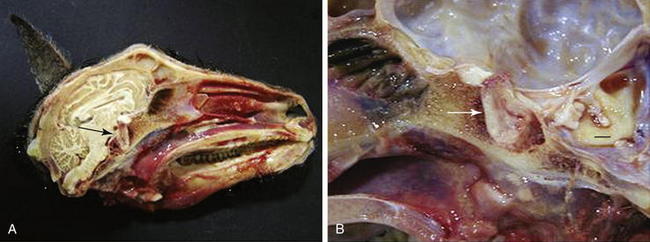
Figure 9-2 A, Gross photograph of the skull and pituitary gland (arrow) of a Pygmy goat demonstrating enlargement of the gland and erosion of the surrounding sphenoid bone. B, Higher-magnification photograph of the pituitary mass (arrow). Erosion and hemorrhage are evident in the sphenoid bone surrounding the sella turcica. Bar = 1 cm.92
Pituitary adenomas also can be endocrinologically inactive but may cause hypopituitarism by mechanical compression of normal pituitary tissue, impaired blood flow to normal tissue, or interference with delivery of hypothalamic regulating hormones through the hypothalamic-hypophyseal portal system.99,101 In humans, pituitary neoplasia is the most common cause of hypopituitarism.101,102 In a doe with a pituitary adenoma, the primary clinical problem was persistent hypoglycemia; however, the complete clinical history and laboratory test results were consistent with partial hypopituitarism, and the doe also was found to have concurrent primary hypothyroidism and hypoadrenocorticism.92
Pituitary Carcinomas
Pituitary carcinomas are uncommon in all species and rare in ruminants. One report described a pituitary carcinoma with metastasis to submandibular lymph nodes in a cow.103 Two reports described pituitary adenocarcinomas in sheep.96,97 In the first of these reports, a ram presented with ocular abnormalities and polyuria and polydipsia, all of which were compatible with a space-occupying lesion in the area of the pituitary gland and in close proximity to the optic chiasm.97 Observations made during the postmortem gross and cytologic examinations were strongly suggestive of a pituitary chromophobe adenocarcinoma.104 A second report described a ewe with a pituitary chromophobe adenocarcinoma (Figure 9-3) that also had two concurrent primary tumors (an intraocular melanoma and an ovine pulmonary adenocarcinoma nodule).96 Unlike in reports of endocrinologically active pituitary adenomas in small ruminants,95,99 pituitary gland adenocarcinomas are either endocrinologically inactive or cause partial hypopituitarism.96,97
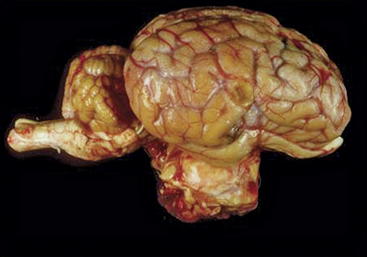
Figure 9-3 Lateral view of the brain of a 14-year-old Rasa Aragonesa ewe showing a neoplastic mass in a ventral position that has replaced the pituitary gland.96 The mass proved to be a pituitary adenocarcinoma.
Differentiation of pituitary adenoma from pituitary carcinoma can be difficult if histopathologic appearance is the sole criterion used for diagnosis. In fact, in human medicine, pathologists consider nonfunctional pituitary tumors to be particularly problematic from a diagnostic standpoint unless extensive clinicopathologic data are available. Even with such data, few prospective clinicopathologic studies have been conducted to develop a clinically useful classification system.105 Furthermore, cellular morphology of adenomas and adenocarcinomas may be similar, and the mitotic rate typically is low for both neoplasms. In some adenocarcinomas, however, histopathologic features may include more frequent mitoses, occasional giant cells, and a greater degree of nuclear pleomorphism.104 Tumor infiltration into the brain parenchyma, large areas of necrosis within the tumor, vascular invasion, the presence of intracerebral metastasis, marked cellular and nuclear pleomorphism, and high mitotic rate are findings consistent with malignancy.104 Because of the permanent and cumulative compression of the posterior lobe, infundibular stalk, and hypothalamus by the mass, the nonmyelinated axons that transport ADH from the site of production in the hypothalamus (supraoptic nucleus and periventricular nucleus) to the site of release in the capillary plexus of the posterior lobe were interrupted.104
Pituitary Abscess Syndrome
Pituitary abscess syndrome is an uncommon condition that occurs in small ruminants, with four and three cases reported in goats and sheep, respectively.106,107 The condition is invariably fatal, and antemortem diagnosis is difficult because of the variety of possible clinical signs. Definitive diagnosis is made at necropsy (Figure 9-4). The most common clinical manifestations reported by owners were anorexia, ataxia, depression, and recumbency. Abnormalities in cranial nerve function are evident in most animals with pituitary abscess syndrome. The most frequent neurologic abnormality is that associated with ocular dysfunction, and the second most frequent finding is dysphagia; the cranial nerve deficits usually are asymmetric. Previous reports of pituitary abscess in cattle and sheep indicated a predilection of the disease for males.106,108 Reports of this condition in sheep and goats, however, suggest a predilection for females.106,107 In light of the importance of the pituitary gland as an endocrine organ, an interesting hypothesis postulates the presence of hormonal imbalances in animals with pituitary abscess syndrome. Determining hormone concentrations might be of value and probably would detect pituitary insufficiency; however, this determination is unlikely to be helpful in clinical practice in light of the acute and fatal course of the disease.
Diagnostics in Pituitary Disease
When the clinical findings are suggestive of a pituitary abnormality, baseline serum hormone concentrations and dynamic hormone testing should be considered, to identify pituitary dysfunction in a timely manner. Unfortunately, the literature contains very few reports of normal pituitary hormone parameters in small ruminants. Available values for normal baseline and stimulated hormone concentrations are listed in Table 9-4. Noninvasive imaging techniques can be used for diagnosis and monitoring treatment. Contrast-enhanced computed tomography (CT) enables direct visualization of the pituitary gland,109,110 but magnetic resonance imaging (MRI) may be more sensitive than CT in detection of a hypothalamic-pituitary pathologic process. MRI is considered the method of choice to detect expansion of tumors into the suprasellar region and compression of structures adjacent to the pituitary gland.111,112
TABLE 9-4 Useful Endocrinologic Hormone Concentrations
Hormone | Abbreviation | Normal Range |
---|---|---|
Thyroxine | T4 | 38-100.5 nmol/L (sheep)118 38.6-54.4 nmol/L (goat)118 |
Triiodothyronine | T3 | 0.97-2.30 nmol/L (sheep)118 1.3-2.92 nmol/L (goat)118 |
Parathyroid hormone | PTH | 99.7 ± 9.3 pmol/L (sheep)232 3.47 ± 0.38 pmol/L (intact PTH, goat)233 |
Parathyroid hormone–related protein | PTHrP | 3.3 ± 1.5 pmol/L (goat)234 |
Calcitonin | CT | 144.6 ± 25.7 pg/mL (sheep)232 |
Cortisol | 62 ± 10 nmol/L (sheep)173 65 ± 8 nmol/L (goat)173 | |
Aldosterone | 28.9 ± 9 pmol/L (sheep)190 5.5 ± 4.3 ng/dL (goat)189 | |
Insulin | 2 ± 20 μIU/mL |
A specific method to differentiate neurogenic DI from primary nephrogenic DI is the modified water deprivation test, which includes, if necessary, an AVP response test.113
Thyroid Gland
Structure and Function
The functional unit of the thyroid gland is the follicle, which consists of a layer of cuboidal epithelial cells surrounding the colloid. The colloid is a clear, viscous fluid that serves as a reservoir for thyroid hormone in the form of the thyroglobulin-hormone complex. The size of the follicles and the length of their cells vary according to the functional stage of the gland, ranging from an inactive squamous cell to the highly active, tall columnar cell. Interspersed between the follicles are thyroid C cells (parafollicular cells), so called because they are the source of calcitonin, the hormone associated with calcium metabolism. A third type of hormonal tissue, the parathyroid, is embedded within the thyroid or located in close proximity.114 These tissues and their hormone products are discussed in greater detail later in the chapter under “Calcium Homeostasis and Parathyroid Hormone.”
Thyroid Hormones and the Hypothalamic-Pituitary-Thyroid Axis
Thyroid-releasing hormone (TRH) is secreted from the paraventricular nucleus of the neurohypophysis and stimulates release of thyroid-stimulating hormone (TSH), or thyrotropin, from the anterior pituitary. This in turn causes secretion of thyroxine (T4) and 3,3′,5-triiodothyronine (T3) from the thyroid gland. The latter is the active form of the hormone; in sheep, T4, T3, and reverse T3 (rT3) account for 90.4%, 8.8%, and 0.7%, respectively, of the secretory product of the thyroid gland.115 T4 can be converted to the more biologically active T3 by 5′-monodeiodinase, which is present in peripheral tissues, especially the liver and kidney. Alternatively, it can be converted to biologically inert rT3 by 5-monodeiodinase, which also may be found in some tissues. The relative concentrations of these enzymes in different tissues play an integral role in determining local hormone concentration and tissue responsiveness.
Most of the effects of thyroid hormone are mediated through binding to thyroid hormone–responsive transcription factors in the nucleus to increase gene expression; however, binding to membrane transporters also may be responsible for mediating some portion of the cellular response. The results of T3 stimulation include increases in heat generation, oxygen utilization, basal metabolic rate, lipid metabolism, cardiac output, neural transmission, glucose availability (glycolysis, gluconeogenesis, absorption), and protein synthesis and catabolism.116–118 Thyroid hormone has been associated with wool growth, weight gain, milk production, and reproduction, and disorders of the hypothalamic-pituitary-thyroid axis may affect any or all of these. (Note: Hormone levels may fluctuate with illness or nutritional issues and therefore reflect systemic status.)
Hypothyroidism
A hypothyroid state may stem from deficits within the gland itself—primary hypothyroidism—or higher up in the axis with TRH or TSH. Alternatively, changes may occur in peripheral deiodination of thyroxine to T3. Decreased 5′-deiodinase activity has been associated with endotoxin insult and expression of cytokines (interleukins and TNF-α) with inflammation or illness.116,119 Results include decreased T3 concentrations, increased rT3 concentrations, and (in severe cases) decreased T4; inflammatory cytokines also can decrease TSH release from the adenohypophysis. The decreased activity of thyroid hormones associated with inflammation and illness is known as euthyroid sick syndrome. It has been well characterized in companion animals such as the dog and in other ruminants, but published studies in small ruminants are lacking at present.
Thyroid Hormone and Reproduction
Thyroid hormone levels play an integral role in the physiology and seasonality of reproduction in small ruminants. Hypothyroidism has been implicated in multiple aspects of reproductive failure in different species, including altered spermatogenesis, reduced testicular growth, irregular cycling, delayed onset of puberty, abortion, still birth, birth of weak offspring, silent heat, and nymphomania.116–117120
Thyroid hormones affect seasonality and transition into anestrus. Ewes thyroidectomized during anestrus began cycling with their euthyroid counterparts but failed to return to anestrus after the breeding season.121 Additionally, thyroid hormone supplementation resulted in earlier onset of anestrus.122 Experiments in the Saanen goat suggest that these changes may be mediated by altered seasonal expression of type II deiodinase in the hypothalamus with changing photoperiod; because this enzyme catalyzes the formation of active T3 from inactive T4, these changes account for increased bioavailability of active hormone in the mediobasal hypothalamus with longer photoperiod.123 Thus thyroid hormone expression in the central nervous system is greater with increased day length; inhibition of GnRH and LH at this level mediates the transition to anestrus and plays a central role in reproductive physiology of the female.
In growing lambs, induction of hypothyroidism by thiourea administration resulted in significant reduction in testicular size, ill-developed seminiferous tubules, and testosterone levels less than 0.02 ng/mL.120 The effects of thyroid hormone deficiency in males are more pronounced in young animals as a consequence of higher numbers of T3 receptors on Sertoli cells, which decrease with age124; because these receptors are virtually gone in the adult animal, these changes are irreversible.
Decreased reproductive efficiency associated with iodine deficiency in adults may be improved with appropriate treatment. Iodine supplementation with 1 mL of Lipiodol (providing 480 mg of iodine) in animals with low urinary iodine concentrations resulted in significantly increased thyroid hormone levels and fertility as compared with iodine-deficient control animals.125
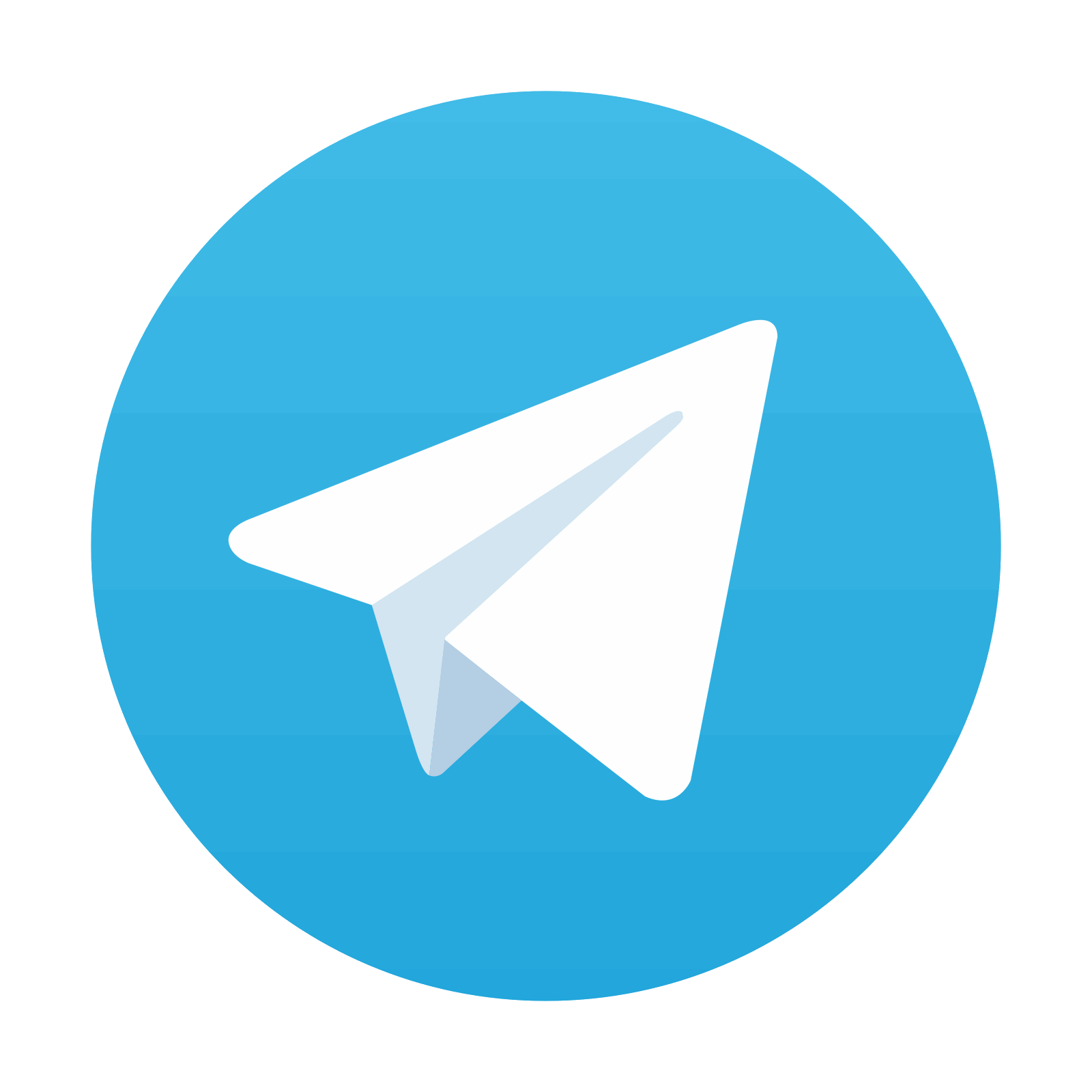
Stay updated, free articles. Join our Telegram channel

Full access? Get Clinical Tree
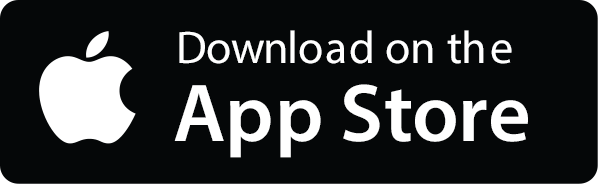
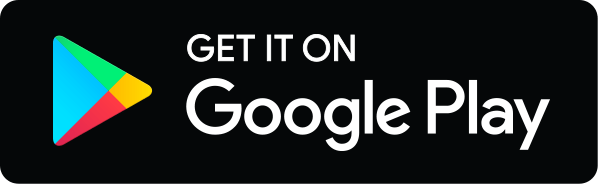